Venous thromboembolism, which comprises deep vein thrombosis (DVT) and pulmonary embolism (PE), is a leading cause of morbidity and mortality. In the United States, it is the third leading cause of cardiovascular death after myocardial infarction and stroke (1). Moreover, approximately 600,000 cases of venous thromboembolism have been estimated to occur annually in the United States (2).
DVT is a process that can affect each one of the deep veins in the body, but is more frequently present in the deep veins of the lower extremity. If undiagnosed and left untreated, both acute and chronic complications can occur. PE is the acute complication that occurs most frequently. Clinical PE occurs in 26% to 67% of patients with untreated proximal deep venous thrombosis and is associated with a mortality rate of 11% to 23% if not treated (3). It is estimated that 40% to 50% of patients with proximal DVT will suffer from silent PE (4).
Phlegmasia cerulea dolens is another rare but serious acute complication of DVT, developing in 1% of patients with iliofemoral thromboses (5). This occurs in the setting of an extensive iliofemoral DVT. Symptoms include acute massive edema, cyanosis, and severe pain. Risk factors for the development of phlegmasia cerulea dolens include underlying advanced malignancy, severe infections, surgery, fractures, etc. Potentially devastating complications include PE that occurs in up to one third of patients, and lower extremity gangrene due to compartment syndrome that occurs as frequently as 50% in some reports (2). Endovascular treatment for this condition and decreasing the risk of PTS will be discussed below.
Post-thrombotic syndrome (PTS) is the major chronic complication of lower extremity DVT. Persistent venous outflow obstruction leads to venous hypertension, which is estimated to occur in over half of patients with ileofemoral DVT and one third of patients with calf vein thrombosis (6). Risk factors for PTS include proximal location ofDVT, male gender, and high D-dimer levels despite anticoagulation. Typical symptoms include leg pain and swelling. Symptoms of venous claudication may occur and are exacerbated by standing and physical activity, and are relieved with rest and elevation of the lower extremities. Venous claudication occurs most commonly in patients treated for ileofemoral DVT with standard anti-coagulation, as the majority of ileofemoral DVT will fail to recanalize with routine anti-coagulation. This persistent proximal venous obstruction leads to reduced outflow of blood, causing increased pressure with muscle contractions that occur with ambulation. Patients who develop PTS are at an increased risk of recurrent venous thromboembolism when compared to patients with uncomplicated infra-inguinal DVT. Finally, lower extremity ulceration, typically over the medial aspect of the lower leg, is a late manifestation of post-thrombotic syndrome.
ANATOMIC CONSIDERATIONS
Veins are larger in caliber and more numerous than arteries. The venous system has a much greater volume capacity than the arterial system. In the lower extremity, the venous circulation can be divided into three distinct systems that include the deep venous system, the superficial venous system, and the perforating or communicating system (See Fig. 30.3). The deep veins include anterior and posterior tibial, peroneal, popliteal, femoral, and iliac veins. These veins can be divided into regions: iliofemoral including iliac and femoral veins, femoral–popliteal including femoral and popliteal veins, and infrapopliteal including peroneal, anterior, and posterior tibial veins. These deep veins supply 90% to 95% of venous outflow from the lower extremity (3).
Valves exist along the entire length of the deep veins of the lower extremity, with the exception of the common iliac veins. They are more numerous and closely situated in the smaller, more distal veins, where the force of gravity is the greatest. The main function of these valves is to ensure antegrade flow of blood and to prevent backflow away from the heart. Blood flow is normally diminished around valves in the venous vasculature, and typically most venous thrombi form behind valve pockets, where the vein wall is slightly dilated behind each of the leaflets.
PATHOPHYSIOLOGY
In 1856 Rudolph Virchow, a German pathologist, postulated three factors precipitating venous thrombosis: venous stasis, endothelial damage to the vessel wall, and hypercoagulability. The main conditions contributing to the formation of venous thrombus in the deep veins of the legs are related to these three basic risk factors described by Virchow and include advanced age, prolonged bed rest, and major surgery. The rate for venous thromboembolism among elderly patients is approximately 20 times that of young adults, making advanced age an important risk factor (1). Major abdominal and orthopedic (e.g., total hip and knee replacement) surgeries are associated with the highest risk. Additional risk factors include: previous venous thrombosis, malignancy, trauma, chronic venous insufficiency, pregnancy and the postpartum period, use of contraceptive pills, and primary and secondary hypercoagulable states. Finally, obesity is also becoming another important risk factor, given the epidemic of this disorder in the United States. Obese individuals have a two to three times likelihood of developing venous thromboembolism (7).
Deep venous thrombosis most often originates in the venous sinuses of the calf muscles, but occasionally originates in the proximal veins of the lower extremity (8,9). Sometimes, the thrombi propagate from the calf veins to the proximal veins. Specifically, 25% of untreated calf thrombi extend into the proximal veins, which is where they are more likely to embolize (7). PE occurs in up to 50% of patients with proximal deep venous thrombosis (8,9). Conversely, calf vein thrombosis rarely leads to significant PE (8).
DIAGNOSTIC TESTING
Unfortunately, the clinical diagnosis of lower extremity DVT is extremely inaccurate, with the classic signs and symptoms of DVT being as common in patients without DVT as they are in those with confirmed DVT. Therefore, if DVT is suspected, objective confirmation is mandatory.
D-dimer is a byproduct in the degradation of cross-linked fibrin by plasmin. In the setting of acute DVT, levels of D-dimer are typically elevated. Nonetheless, D-dimer levels are also increased by conditions such as recent major surgery, hemorrhage, trauma, pregnancy, malignancy, or acute arterial thrombosis. As a result, D-dimer assays are sensitive but not specific. The high sensitivity makes it possible to exclude DVT as a diagnosis, but the low specificity and positive predictive value force confirmatory non-invasive testing after a positive result.
Historically, ascending venography was the gold standard for the diagnosis of acute DVT. However, it is an invasive test, not easily repeatable, and impossible to perform or interpret in 9% to 14% of patients. Ascending venography also fails to visualize all venous segments in 10% to 30% of studies. Interobserver disagreements occur in 4% to 10% of studies. Because of these limitations, ascending venography has been replaced by venous duplex ultrasonography as the most widely used diagnostic test for acute DVT.
The sensitivity and specificity of venous ultrasonography for the diagnosis of symptomatic proximal DVT are 97% and 94%, respectively (10). A complete ultrasound evaluation of the lower extremities includes an assessment of venous compressibility, the presence of intraluminal echoes, venous flow characteristics, and luminal color filling. Venous incompressibility, or failure to completely coapt the venous walls with gentle probe compression, is the most widely used diagnostic criterion for acute DVT. Adjunctive gray-scale findings include the appearance of echogenic thrombus within the vein lumen and dilation of an acutely thrombosed segment. Normal flow in the proximal veins should be spontaneous and should vary with respiration (increasing during expiration and decreasing during inspiration).
Other non-invasive imaging techniques for the diagnosis of lower extremity DVT include magnetic resonance venog-raphy (MRV) and CT angiography. These studies are comparable to venography, but they cannot be performed at the bedside. In addition, CT angiography carries the risk of contrast allergy and radiation exposure, whereas MRV requires the use of gadolinium, which limits its applicability in patients with renal insufficiency. Both studies are more expensive than venous duplex ultrasonography.
A combined strategy using an assessment of clinical probability, D-dimer testing, and venous duplex ultrasonography provides the greatest diagnostic potential to confirm or reject the diagnosis of DVT. The negative predictive value of this approach is almost 100% in outpatients with a low pretest clinical probability for DVT.
TREATMENT
There are four generally accepted goals for the treatment of lower extremity DVT: (1) diminish the severity and duration of lower extremity symptoms, (2) prevent PE, (3) minimize the risk of recurrent venous thrombosis, and/or (4) prevent PTS. It is uniformly agreed upon that anti-coagulation is required to prevent thrombus growth and PE. Intravenous unfractionated heparin was historically the medication of choice, but low-molecular-weight heparin (LMWH) has emerged as the anti-coagulant of choice in the management of DVT. While both agents are effective and relatively safe in lower extremity DVT management, LMWH is suitable for outpatient therapy because of improved bioavailability and more predictable anticoagulant response. Serious potential complications of heparin therapy, such as heparin-induced thrombocytopenia and osteoporosis (with long-term use), occur less commonly with LMWH. Either unfractionated heparin or LMWH is used as a bridge to warfarin therapy, which should ideally be initiated within 24 hours of the diagnosis of DVT. The ideal international normalized ratio (INR) is 2.0 to 3.0. The optimal duration of warfarin therapy is controversial and dependent on the clinical situation: 6 to 12 weeks for a symptomatic isolated calf vein thrombosis, 3 months for the first DVT event with a known reversible or time-limited risk factor for venous thromboembolic disease, such as trauma or surgery, at least 6 months for the first episode of idiopathic venous thromboembolic disease, and at least 12 months to lifelong therapy for recurrent idiopathic venous thromboembolic disease or permanent risk factor such as thrombophilia (11). It is likely that there will be a major shift in recommendations for the treatment of DVT following the recent approval of the oral direct thrombin inhibitor, dabigatran. This agent, which appears to be equal in efficacy to warfarin for the prevention in stroke for patients with atrial fibrillation has the advantage of not requiring routine monitoring, and is not influenced by diet. On that basis, it is likely to replace warfarin as the default chronic therapy for DVT.
Although medical therapy with anti-coagulation is the mainstay of the initial management of lower extremity DVT, many patients, particularly those with large proximal iliofemoral DVT, have persistent leg edema, pain, and difficulty ambulating. These symptoms arise from venous hypertension caused by outflow obstruction. Relief of outflow obstruction is one of the primary goals of therapy for lower extremity DVT, but this is typically not accomplished with anti-coagulation alone. Thrombus regression occurs in only 50% of patients with ileofemoral DVT (3). Endovascular techniques, including catheter-directed thombolysis (CDT), mechanical thrombectomy, and stenting, offer practical options in treating ileofemoral DVT.
Catheter-Directed Thrombolysis
In the early 1990s, Semba and Drake (12) first reported the feasibility of CDT for iliofemoral thrombosis as an alternative to systemic anticoagulation, systemic thrombolysis, or surgical venous thrombectomy.
CDT, or the delivery of thrombolytic agents directly into the thrombus, offers significant advantages over systemic therapy, which may fail to reach and penetrate an occluded venous segment. Because thrombolytic agents activate plasminogen within the thrombus, the delivery of the drug to that site enhances its effectiveness. CDT allows the delivery of higher concentrations of the drug to the treatment site, resulting in improved lysis rates, reduced duration of treatment, and reduced complications associated with exposure of the patient to systemic thrombolytic therapy. After successful CDT, it is hoped that preservation of valvular function and removal of the obstructing thrombus will result in a lower incidence of PTS. In addition, this endovascular approach allows for the detection and correction of any underlying venous obstructive lesions with balloon angioplasty and/or stents that should help reduce the rate of recurrent events.
Currently, no thrombolytic agents have been approved for CDT for the treatment of DVT by the US Food and Drug Administration (FDA). Therefore, the use of thrombolytic agents in CDT for venous thrombosis constitutes an “off-label” use. There are five thrombolytic agents available in the United States that can be used during CDT for venous thrombosis. Although the various agents have unique properties that might theoretically imply superiority of one over another, there is no peer-reviewed consensus on a superior or “best choice” agent for CDT for venous thrombosis. The literature on CDT for venous thrombosis has a paucity of prospective randomized comparative trials. Therefore, the choice of thrombolytic agent is usually individualized based on the physician’s discretion.
The largest published experience with CDT has come from the National Venous Thrombolysis Registry (12) that included 287 patients treated with urokinase and monitored for up to 1 year. Overall, 71% of the patients were treated for iliofemoral DVT. Complete dissolution of thrombus was achieved in 31% of cases, and partial thrombus dissolution was reported in an additional 52%. Primary patency at 1 year was 60%. Preservation of valvular competence was demonstrated in 72% of the patients with complete thrombolysis. Table 27.1 reviews the available clinical experience for CDT in the treatment of DVT.
The decision to perform CDT for lower extremity DVT must be individualized to each case based on a risk-benefit analysis. The CDT technique for lower extremity DVT is not standardized; however, the primary goal is to deliver the lytic agent directly into venous clot.
Access
The location of the lower extremity DVT and the patients’ symptoms determine the specific venous access site chosen. For most cases of iliofemoral DVT, the ipsilateral popliteal vein is favored if the clinical situation allows. With the patient prone on the angiographic table, the venous access site should be accessed under ultrasound guidance with a small-gauge echogenic needle (e.g., 21-gauge micropuncture needle). Should the popliteal vein be thrombosed, the ipsilateral posterior tibial vein can be cannulated. After popliteal vein cannulation, a 5 Fr. short sheath is introduced, through which all subsequent catheters are exchanged. Next, a baseline venogram is obtained using the venous sheath, and then a combination of 0.035” straight and curved glidewires is used to cross the occluded venous segment. After wire and then catheter traversal of the occluded venous segment, venography is repeated to confirm the intraluminal position of the catheter. The catheter is then exchanged for a 5 Fr. infusing coaxial system, consisting of a proximal multiside-hole infusion catheter (e.g., Cragg— McNamara, ev3) and a distal infusion wire (ProStream, ev3). The Cragg—McNamara infusion catheter comes in 4 and 5 Fr. sizes, and varies in both the entire length of the catheter (40 to 135 cm) and the active infusion length (5 to 50 cm). Similarly, the ProStream infusion wire varies in the entire length of the wire (145 to 175 cm) and the active infusion length (6 to 12 cm).It is essential to position the system directly into the thrombus to maximize plasminogen activation at the site of obstruction.
Indications for placement of an IVC filter during CDT for lower extremity DVT fall within the same spectrum as that for routine prophylactic IVC filter placement (Table 29.1). The use of IVC filters during lower extremity venous thrombolysis has always been controversial because of the low incidence of complications that occur when lysis is performed without filter protection. With the advent of retrievable IVC filters, the authors’ practice is to implement them routinely when performing CDT for iliofemoral DVT. This seems particularly reasonable for patients in whom the venogram defines a true “free-floating” iliac vein thrombus or for those with a documented pulmonary embolus and limited cardiopulmonary reserve for additional emboli.
Following placement of the infusion catheter/wire and/ or IVC filter placement, the patient is monitored in the inter-ventional recovery unit as the thrombolytic agent is infused. It is quite common, particularly with extensive thrombus burden, for the duration of therapy to exceed 24 hours. Follow-up venography should be performed every 12 hours to assess and/ or reposition the infusion catheter and/or wire directly into any remaining thrombus. Weighing the risk versus the benefit of lytic therapy, the infusion should be continued until adequate lysis is achieved.
If venous patency has been restored and there is no underlying stenotic/occlusive lesion, thrombolysis is discontinued and anticoagulation is initiated. Hemodynamically significant lesions that are uncovered in the iliac veins should be considered for endovascular stenting, although the long-term benefits of venous stenting are not known. However, if left untreated, a significant iliac vein stenosis appears to be a significant risk of early rethrombosis (Fig. 27.1).
Use of metallic stents has been associated with good outcomes in central veins such as the IVC and iliac veins (13,14). If an underlying stenosis after successful CDT is believed to be secondary to iliac vein compression, stent deployment is required, because vein compression typically does not respond to stand-alone angioplasty. This iliac vein compression, or May— Thurner syndrome, typically involves extrinsic compression of the left common iliac vein by the crossing right common iliac artery, at the iliocaval junction (Fig. 27.2). Self-expanding stents are usually preferred because of their longitudinal flexibility and ability to conform to various venous configurations. In the iliac veins, we usually utilize self-expanding stents between 10 and 16 mm in diameter, with 12- to 14-mm-diameter stents being the most commonly used. Post-dilation angioplasty should be performed and should be gauged to the patient’s perception of pain during balloon inflation. A significant limitation of using undersized stents and balloons is the high rate of restenosis and thrombotic reocclusion. Therefore, appropriate sizing of both stents and balloons is essential to achieve favorable outcomes. Care should be exercised to avoid stenting across the common femoral vein, particularly at the saphenofemoral junction.
Percutaneous Mechanical Thrombectomy (PMT)
Important limitations of CDT for lower extremity DVT include the time required to achieve lysis, need for intensive care monitoring, hemorrhagic risks, and cost. With these issues in mind, PMT is conceptually attractive because such a technique may result in a shorter time to vein patency, shorter length of hospital stay, reduction in hemorrhagic risk, and overall cost savings because of reduced hospitalization and elimination or reduction in use of thrombolytic drugs.
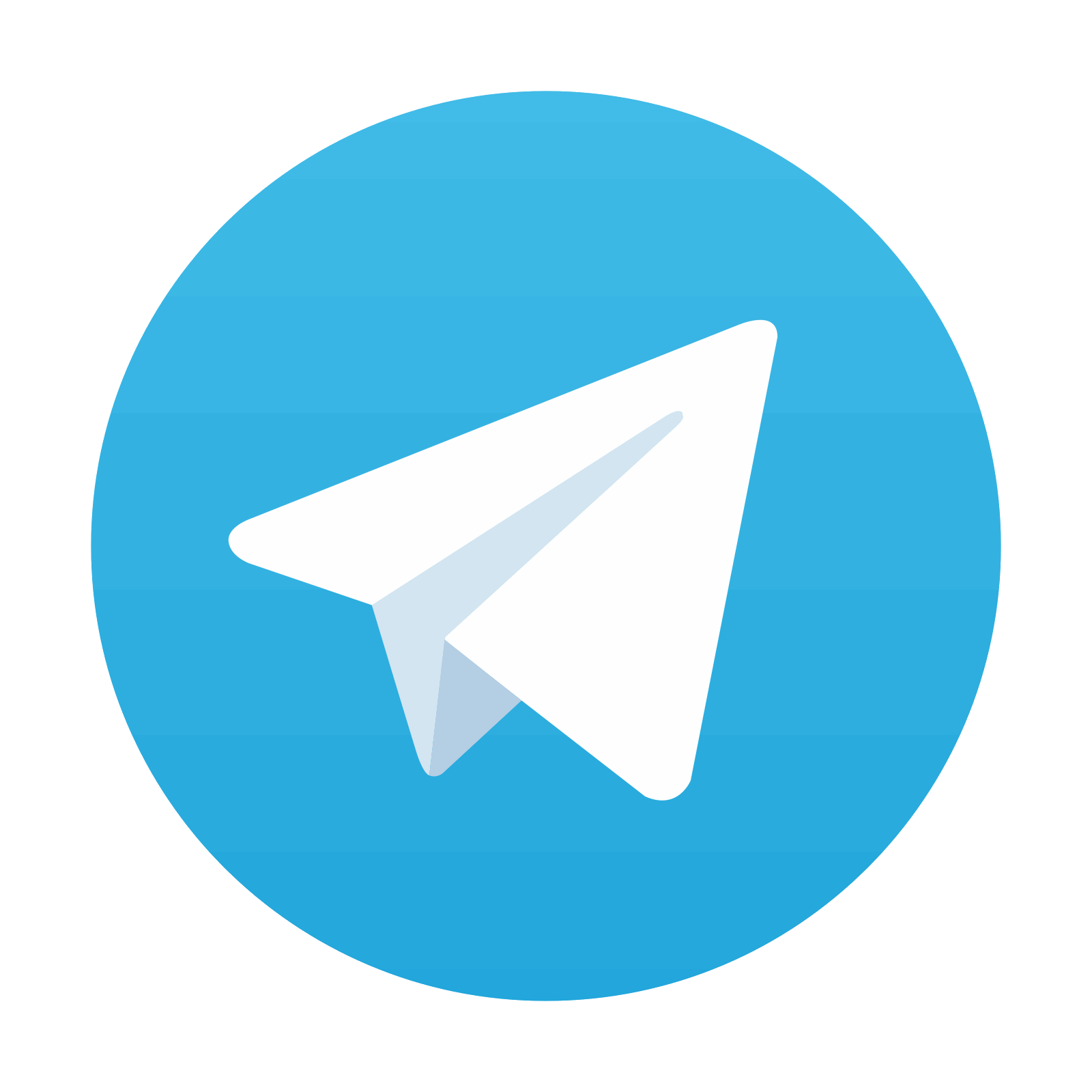
Stay updated, free articles. Join our Telegram channel

Full access? Get Clinical Tree
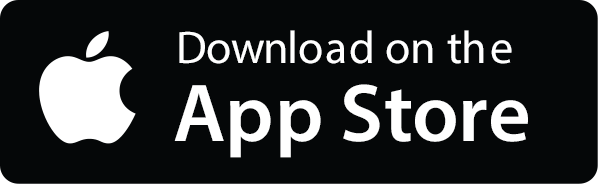
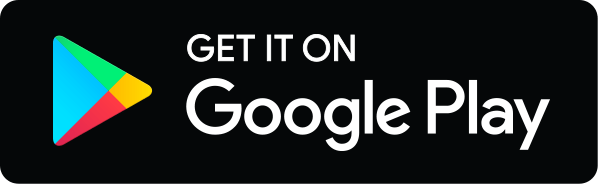