Background
The aim of this study was to evaluate the value of noninvasive assessment of cardiac allograft vasculopathy (CAV) in heart-transplanted patients by exercise stress myocardial deformation and coronary flow reserve (CFR) assessment.
Methods
Fifty-eight heart-transplanted patients underwent semisupine exercise echocardiography with assessment of left ventricular (LV) longitudinal myocardial deformation. CAV was assessed by coronary angiography and noninvasive CFR by 15 O-H 2 O positron emission tomographic imaging and Doppler echocardiography. Patients were divided into three groups on the basis of angiographic CAV: no CAV ( n = 21), mild CAV ( n = 19), and severe CAV ( n = 18).
Results
Patients with severe CAV had significantly lower LV global longitudinal strain (GLS) at rest (no CAV, −16 ± 2%; mild CAV, −15 ± 2%; severe CAV, −12 ± 4%; P < .001), failed to increase LV GLS during exercise (no CAV, −5.7 ± 2.0%; mild CAV, −3.3 ± 2.9%; severe CAV, −0.2 ± 2.8%; P < .0001), and had significantly lower echocardiographic coronary flow velocity reserve (CFVR) (no CAV, 3.2 ± 0.4; mild CAV, 2.7 ± 0.7; severe CAV, 1.8 ± 0.5; P < .0001) and PET CFR (no CAV, 3.4 ± 0.9; mild CAV, 3.1 ± 0.9; severe CAV, 1.9 ± 0.8; P < .0001). Furthermore, patients with mild CAV had significantly lower exercise LV GLS and echocardiographic CFVR than patients with no CAV. Exercise LV GLS, echocardiographic CFVR, and PET CFR were significantly correlated with the presence of severe CAV in a logistic regression model (LV GLS odds ratio, 0.71; 95% CI, 0.60–0.84; P < .0001; echocardiographic CFVR odds ratio: 0.06; 95% CI, 0.01–0.23; PET CFR odds ratio, 0.17; 95% CI, 0.07–0.46). This relation remained significant after adjustment for symptoms and time since transplantation.
Conclusions
Noninvasive assessment of LV longitudinal myocardial deformation during exercise is feasible and strongly associated with the presence and degree of CAV. Exercise stress myocardial deformation analysis, echocardiographic CFVR, or PET CFR may serve as a noninvasive model for the detection of CAV.
Cardiac allograft vasculopathy (CAV) remains the leading long-term cardiac cause of death after heart transplantation (HTX). CAV is characterized by diffuse, concentric intimal thickening involving both epicardial vessels and the coronary microvascular system. CAV is often clinically silent because of denervation of transplanted hearts. Traditional CAV surveillance involves annual or biannual coronary angiography (CAG) and estimation of left ventricular (LV) systolic and diastolic function by echocardiography. At some centers, CAG is combined with either intravascular ultrasound or optical coherence tomography. CAG, intravascular ultrasound, and optical coherence tomography are invasive, time-consuming and expensive, and associated with significant patient discomfort. Noninvasive screening is therefore desirable. Noninvasive coronary flow velocity reserve (CFVR) by Doppler echocardiography, coronary flow reserve (CFR) assessment by 13 N-ammonia positron emission tomographic (PET) imaging, single-photon emission computed tomography, and magnetic resonance perfusion have shown significant correlation with CAV but have not yet found a significant role in CAV monitoring. Oxygen-15-H 2 O is considered the ideal perfusion tracer for PET evaluations, and 15 O-H 2 O PET imaging has shown high diagnostic performance in patients with ischemic heart disease. However, the diagnostic performance of 15 O-H 2 O PET imaging for CAV assessment remains to be determined. The subendocardial longitudinally oriented layers of the myocardium are very sensitive to ischemia, edema, and fibrosis, and studies of patients with ischemic heart disease have shown that CFVR strongly correlates with the degree of LV longitudinal myocardial deformation. Assessment of LV systolic global longitudinal strain (GLS) during resting conditions by two-dimensional (2D) speckle-tracking echocardiography has been shown to be superior to traditional parameters such as LV ejection fraction (LVEF) and Doppler parameters for the assessment of CAV-induced graft function involvement. However, the value and feasibility of 2D speckle-tracking echocardiography during exercise for CAV assessment remains to be determined.
Thus, in the present study we aimed to evaluate the value of LV deformation capacity during exercise, assessment of echocardiographic CFVR, and assessment of 15 O-H 2 O PET CFR for the noninvasive assessment of functional significant CAV.
Methods
Study Population
The study population consisted of 58 HTX patients who were referred for annual follow-up in the period from September 2013 until March 2015. Only patients ≥18 years of age were included, after informed and written consent was obtained according to the principles of the Declaration of Helsinki. The study was approved by the local scientific ethics committee. All individuals underwent comprehensive echocardiographic assessment of their myocardial function during rest and semisupine exercise testing. We assessed myocardial perfusion by echocardiographic CFVR and 15 O-H 2 O PET CFR. Resting and stress echocardiography and echocardiographic CFVR were performed within a 3-hour period. The 15 O-H 2 O PET examinations were performed <3 weeks before or after echocardiography.
Echocardiography
We used a commercially available ultrasound system (Vivid 9; GE Vingmed Ultrasound AS, Horten, Norway) with a 3.5-MHz phased-array transducer (M5S). Frame rate in all patients was >60 frames/sec both at rest and during exercise.
All patients were in sinus or atrial pace rhythm at the time of examination. From an apical view, 2D LVEF measurements were based on the biplane method of disks. Peak systolic mitral annular velocities (S′) was estimated from the tissue velocity image as an average of septal, lateral, anterior, and posterior velocities. The magnitude of systolic GLS was obtained from frame-by-frame tracking of speckle patterns throughout the left-sided myocardium in standard 2D cine loops. The speckle area of interest was manually adjusted for optimal tracking results. Segments with unacceptably low tracking quality were excluded. GLS was calculated at the time in systole when the value peaked for the endocardial, middle (LV GLS), and epicardial layers using a 17-segment model. Systole was defined as the time from aortic valve opening to aortic valve closing. Wall motion score index was calculated using a 16-segment model. At each stage of semisupine bicycle exercise, we measured biplane LVEF, wall motion score index, LV GLS, S′, and pulsed Doppler mitral flow. Our group has previously reported repeatability for LV GLS and found very low intra- and interobserver variation in HTX patients.
Full-volume three-dimensional data sets were obtained using a 3.5-MHz matrix-array transducer (4V-D). Three-dimensional LVEF was calculated from an electrocardiographically gated data set sampled through six cardiac cycles during apnea. We measured LV mass using full-volume images and calculated LV mass index as LV mass/body surface area.
Data were analyzed using dedicated software (EchoPAC PC SW-Only version 113; GE Healthcare, Milwaukee, WI) by a single investigator (T.S.C.) blinded to clinical status, biopsy status, and vasculopathy status.
Assessment of CFVR
Echocardiographic CFVR was assessed using echocardiography in a modified apical view of the distal part of the left anterior descending coronary artery using a high-frequency broadband transducer (S6-D; GE Healthcare). All subjects abstained from caffeine-containing drinks for 24 hours before testing. The coronary flow velocity was obtained by color Doppler flow mapping guidance. Subsequently, a sample volume was positioned on the color signal in the left anterior descending coronary artery using pulsed-wave Doppler. After baseline recording, adenosine was administered by intravenous infusion (140 μg/kg/min) for ≥120 sec, and hyperemic flow profiles were recorded. Both baseline and hyperemic flow velocities were estimated as averages of two consecutive heartbeats. Echocardiographic CFVR was estimated as the ratio of hyperemic to baseline peak diastolic coronary flow velocity.
Position Emission Tomography
PET scans were performed both at rest and after adenosine-induced hyperemic conditions within the same session on a Siemens Biograph 64 TruePoint TrueV PET/CT (Siemens Healthcare, Erlangen, Germany). All subjects abstained from caffeine-containing drinks for 24 hours before testing. A single low-dose computed tomographic scan was performed for attenuation correction. Afterward, a list-mode emission scan was started simultaneously with a bolus injection of 400 MBq of 15 O-H 2 O using an automated injection system, followed by a 20-mL saline flush. Adenosine infusion (140 μg/kg/min) was started 2 min before the injection of 15 O-H 2 O and continued for the 6-min PET scan. Emission data were reconstructed into a dynamic scan consisting of 22 time frames (1 × 10 sec, 8 × 5 sec, 4 × 10 sec, 2 × 15 sec, 3 × 20 sec, 2 × 30 sec, and 2 × 60 sec), with a matrix size of 4 × 4 × 4 mm/frame using the TrueX reconstruction algorithm (three iterations, 21 subsets, postfilter 5-mm three-dimensional Gaussian). Dynamic data were analyzed using Cardiac VUer, and absolute measures of resting and hyperemic myocardial blood flow were obtained. We calculated PET CFR as the ratio between hyperemic and resting blood flow on both a global level and a regional level with respect to coronary anatomy.
Exercise Protocol
All patients performed a multistage, symptom-limited, semisupine bicycle exercise test using the Echo Cardiac Stress Table (Lode B.V., Amsterdam, The Netherlands). Workload started at 0 W and was increased by 25 W every 3 min. Patients were encouraged to maintain a fixed pedaling speed of 60 rounds/min and to exercise until exhaustion (Borg > 18).
Angiography and CAV
CAG was performed through a 6-F sheath inserted in the right femoral artery. Coronary arteries were imaged after administering intracoronary nitroglycerin (200 μg) into the left main and right coronary arteries. At least two projections of each coronary artery were acquired. All three major branches were analyzed offline using 2D quantitative coronary angiographic (QCA) imaging. Maximal stenosis of each vessel was automatically calculated using a proximal and distal reference area. Patients were divided into three groups on the basis of QCA analysis of maximal stenosis: no CAV, no visual stenosis by eyeballing and maximal QCA stenosis <25%; mild CAV, visual stenosis or QCA stenosis of 25% to 70%; severe CAV, occluded vessel or QCA stenosis >70%. The cutoff value for severe CAV is in accordance with the guidelines of the International Society for Heart and Lung Transplantation (ISHLT). In this classification, CAV is divided into four groups: ISHLT class 0 (no CAV), ISHLT class 1 (<50% left main stenosis and <70% stenosis of a major branch), ISHLT class 2 (<50% left main stenosis and >70% stenosis of a major branch), and ISHLT class 3 (>50% left main stenosis or >70% stenosis of two or more major branches). ISHLT classes 1 and 2 are classified as ISHLT class 3 if restrictive LV physiology or reduced LVEF is demonstrated. We classified patients with previous lesions demanding percutaneous coronary intervention with severe vasculopathy even if no present severe lesions were seen, because of the common conception that CAV is a diffuse disease affecting both epicardial vessels and the microvascular system.
Endomyocardial Biopsy
Previous acute rejection episodes were histopathologically graded in three forms, according to the guidelines of the ISHLT, and biopsy scores were calculated as previously described.
Statistical Analysis
Normally distributed data are presented as mean ± SD, and data not normally distributed are presented as medians and interquartile ranges. Categorical data are presented as counts with percentages. Histograms and Q-Q plots were used to check continuous values for normality. Between-group differences were assessed by analysis of variance for normally distributed data and the Kruskal-Wallis rank test for data not normally distributed. Bonferroni correction was used to counteract the effect of chance by multiple comparisons. A linear regression model was used to compare continuous variables. We adjusted for time since transplantation and New York Heart Association (NYHA) functional class in a multivariate logistic regression model.
All tests were two sided, and P values < .05 were considered to indicate statistical significance. Analyses were performed using Stata/IC 12 (StataCorp LP, College Station, TX).
Results
Study Population
Twenty-one patients (36%) had no CAV, 19 patients (33%) had mild CAV, and 18 patients (31%) had severe CAV according to 2D QCA findings. Three of the 18 patients with severe CAV had no significant stenosis (>70%) at the time of examination but had previously been treated with percutaneous coronary intervention. Table 1 displays patients’ demographics in the three CAV groups. Patients with severe CAV were more symptomatic ( P < .0001) and had longer time since HTX ( P < .001) than those with no and mild CAV. Patients with severe CAV were more likely to be treated with cyclosporine ( P < .01) and less likely to be treated with tacrolimus ( P < .01) at the time of examination. Resting troponin T ( P < .05) and N-terminal pro–brain natriuretic peptide ( P < .05) were significantly higher in the group with severe CAV than in patients with no or mild CAV.
Variable | No CAV | Mild CAV | Severe CAV | ANOVA P |
---|---|---|---|---|
( n = 21) | ( n = 19) | ( n = 18) | ||
Men | 76% | 74% | 67% | 0.80 |
Donor age (y) | 38 ± 11 | 43 ± 15 | 45 ± 9 | 0.22 |
Age (y) | 52 ± 14 | 52 ± 11 | 56 ± 13 | 0.58 |
Time since HTX (y) | 5 ± 5 | 9 ± 5 | 13 ± 6 | <0.001 ∗ |
BMI (kg/m 2 ) | 25 ± 4 | 26 ± 4 | 25 ± 4 | 0.94 |
NYHA class > I | 0% | 16% | 72% | <0.0001 ∗ |
Rejection score | 7.7 ± 3.9 | 10.1 ± 7.7 | 10.4 ± 4.4 | 0.25 |
Medication | ||||
Prednisolone | 52% | 32% | 22% | 0.13 |
Cyclosporine | 38% | 16% | 67% | <0.01 ∗ |
Tacrolimus | 62% | 84% | 33% | <0.01 ∗ |
Mycophenolate | 86% | 79% | 56% | 0.09 |
Everolimus | 19% | 21% | 50% | 0.07 |
Statins | 86% | 89% | 89% | 0.93 |
ACE inhibitor/ARB | 76% | 68% | 72% | 0.87 |
Calcium blocker | 48% | 58% | 33% | 0.34 |
Furosemide or bumetanide (%) | 14% | 11% | 39% | 0.07 |
Thiazide | 14% | 32% | 22% | 0.44 |
Aspirin | 29% | 58% | 94% | <0.0001 ∗ |
Biochemistry | ||||
Creatinine (μmol/L) | 83 (80–117) | 101 (82–138) | 122 (80–151) | 0.08 |
Total cholesterol (mmol/L) | 4.4 (3.8–5.4) | 4.6 (3.7–4.8) | 5.0 (4.2–6.2) | 0.14 |
Troponin T (ng/l) | 11 (5–13) | 11 (5–18) | 21 (5–45) | <0.05 ∗ |
NT-proBNP (ng/L) | 297 (147–397) | 316 (154–773) | 1,257 (432–1,765) | <0.05 ∗ |
Resting Echocardiography
Table 2 displays the echocardiographic parameters at rest in the three CAV groups. Traditional parameters of LV filling did not differ between the groups, except for the E/e′ ratio, which was significantly higher in the group with severe CAV ( P < .05). Left atrial volume tended to be larger in the severe CAV group than the no and mild CAV groups ( P = .06). LV systolic function measured by 2D and three-dimensional LVEF was slightly impaired in the group with severe CAV. We found significantly prolonged time to systolic peak deformation ( P < .01) in addition to reduced longitudinal deformation of all myocardial layers in patients with severe CAV. LV GLS was −12 ± 4% in the group with severe CAV compared with −16 ± 2% in the group with no CAV ( P < .001). No significantly difference in LVEF or LV GLS was seen between patients with no CAV and those with mild CAV.
Variable | No CAV | Mild CAV | Severe CAV | ANOVA P |
---|---|---|---|---|
( n = 21) | ( n = 19) | ( n = 18) | ||
3D echocardiography | ||||
LV mass index (g/m 2 ) | 73 ± 10 | 77 ± 11 | 85 ± 11 | <0.01 † |
3D full-volume LVEF (%) | 63 ± 5 | 61 ± 7 | 55 ± 11 | <0.05 † |
2D echocardiography | ||||
2D LVEF (Simpson biplane) (%) | 65 ± 6 | 62 ± 8 | 57 ± 12 | 0.07 |
LV EDV (mL) | 97 ± 31 | 83 ± 15 | 103 ± 37 | 0.33 |
LV ESV (mL) | 35 ± 16 | 32 ± 9 | 47 ± 27 | <0.05 † |
WMSI | 1.02 ± 0.1 | 1.06 ± 0.1 | 1.28 ± 0.35 | <0.01 † |
LA volume (mL/m 2 ) | 39 ± 13 | 42 ± 16 | 54 ± 22 | 0.06 |
LVOT CO (L/min) | 5.5 ± 1.5 | 5.0 ± 1.0 | 5.3 ± 1.8 | 0.57 |
Mitral Doppler flow | ||||
E/A ratio | 2.1 ± 0.6 | 2.0 ± 0.9 | 2.1 ± 0.7 | 0.86 |
E-dec (msec) | 159 ± 27 | 169 ± 56 | 155 ± 60 | 0.65 |
IVRT (msec) | 64 ± 14 | 68 ± 23 | 70 ± 30 | 0.89 |
E/e′ ratio | 8.4 ± 2.5 | 9.9 ± 6.9 | 13.2 ± 5.8 | <0.05 † |
Tissue Doppler | ||||
LV S′ mean (cm/sec) | 6.2 ± 1.2 | 5.8 ± 1.1 | 5.1 ± 1.1 | <0.05 † |
2D STE | ||||
LV GLS (%) | −16 ± 2 | −15 ± 2 | −12 ± 4 | <0.001 † |
LV GLS endocardial (%) | −19 ± 3 | −19 ± 3 | −15 ± 4 | <0.001 † |
LV GLS epicardial (%) | −13 ± 2 | −13 ± 2 | −10 ± 3 | <0.001 † |
LV TTP (msec) | 38 ± 13 | 42 ± 11 | 55 ± 15 | <0.01 † |
LV GCS (%) | −21 ± 4 | −19 ± 3 | −17 ± 5 | <0.05 † |
Hemodynamics | ||||
Heart rate (beats/min) | 83 ± 11 | 81 ± 17 | 88 ± 13 | 0.33 |
Diastolic blood pressure (mm Hg) | 85 ± 8 | 82 ± 10 | 87 ± 12 | 0.30 |
Systolic blood pressure (mm Hg) | 126 ± 13 | 131 ± 13 | 135 ± 19 | 0.23 |
Double product ∗ | 10,465 ± 1,912 | 10,646 ± 2,363 | 11,848 ± 2,563 | 0.14 |
∗ Heart rate × systolic blood pressure.
Figure 1 displays the imaging modalities used in the study in a patient with moderate to severe CAV and a patient without CAV.

Two-Dimensional QCA Analysis, Echocardiographic CFVR, and PET CFR
Table 3 displays the results of 2D quantitative CAG, echocardiographic CFVR, and PET CFR. The maximal stenosis was 78 ± 21% in the group with severe CAV, 39 ± 8% in the group with mild CAV, and 20 ± 3% in the group with no CAV. A stepwise significant reduction between groups was seen for echocardiographic CFVR and PET CFR ( Figure 2 ).
Variable | No CAV | Mild CAV | Severe CAV | ANOVA P |
---|---|---|---|---|
( n = 21) | ( n = 19) | ( n = 18) | ||
Coronary flow | ||||
Echocardiography | ||||
Coronary flow velocity rest (m/sec) | 0.2 ± 0.0 | 0.3 ± 0.1 | 0.3 ± 0.1 | <0.001 ∗ |
Coronary flow velocity hyperemia (m/sec) | 0.7 ± 0.1 | 0.7 ± 0.2 | 0.6 ± 0.2 | 0.20 |
Echocardiographic CFVR (ratio) | 3.2 ± 0.4 | 2.7 ± 0.7 | 1.8 ± 0.5 | <0.0001 ∗ |
Positron emission tomography | ||||
Myocardial blood flow rest (mL/g/min) | 1.1 ± 0.3 | 1.2 ± 0.3 | 1.2 ± 0.2 | 0.82 |
Myocardial blood flow hyperemia (mL/g/min) | 3.8 ± 0.9 | 3.5 ± 0.9 | 2.4 ± 1.2 | <0.001 ∗ |
PET CFR (ratio) | 3.4 ± 0.9 | 3.1 ± 0.9 | 1.9 ± 0.8 | <0.0001 ∗ |
2D QCA analysis | ||||
Maximal stenosis (%) | 20 ± 3 | 39 ± 8 | 78 ± 21 | <0.0001 ∗ |
Number of affected vessels | 0 | 1.2 ± 1.2 | 2.5 ± 0.8 | <0.0001 ∗ |

In a linear regression model, we found good correlation between PET CFR and echocardiographic CFVR ( r = 0.80, P < .0001). Likewise, we found highly significant correlations between maximal stenosis by 2D quantitative CAG and echocardiographic CFVR ( r = −0.71, P < .0001) and between maximal stenosis by 2D quantitative CAV and PET CFR ( r = −0.60, P < .0001) ( Figure 3 ).
Exercise Echocardiography
Echocardiographic parameters at peak exercise in the three CAV groups is presented in Table 4 . A stepwise significant reduction in peak workload was seen comparing the groups ( P < .05). During exercise, no differences in peak diastolic Doppler parameters or Δ values were seen between the groups. LV systolic function remained reduced in the group with severe CAV compared with the groups with no and mild CAV. LVEF increased uniformly in all three groups. In contrast, patients with severe CAV failed to increase LV GLS during exercise (LV GLS rest −12.1 ± 3.8% vs LV GLS peak −12.4 ± 5.5%, P = .72), whereas LV GLS increased significantly in patients with no and mild CAV. Interestingly, patients with no CAV had a significantly higher increase in LV GLS during exercise compared with the mild CAV group (−5.7 ± 2% vs −3.3 ± 2.9%, P < .05). This led to borderline higher LV GLS peak in the group without CAV than the mild CAV group (−22 ± 3% vs 19 ± 3%, P = .05).
Variable | No CAV | Mild CAV | Severe CAV | ANOVA P |
---|---|---|---|---|
( n = 21) | ( n = 19) | ( n = 18) | ||
Peak (W) | 113 ± 37 | 92 ± 40 | 78 ± 28 | <0.05 † |
Exercise echocardiography | ||||
LVEF (Simpson biplane) (%) | 73 ± 6 | 68 ± 8 | 63 ± 12 | <0.01 † |
ΔLVEF (%) (peak stress to rest) | 8.3 ± 4.2 | 6.5 ± 7.2 | 6.4 ± 7.0 | 0.56 |
LV EDV (mL) | 96 ± 24 | 93 ± 17 | 103 ± 37 | 0.93 |
ΔLV EDV (mL) | −0.3 ± 15 | 9.8 ± 13 | −0.1 ± 19 | 0.09 |
LV ESV (mL) | 26 ± 10 | 29 ± 6 | 40 ± 25 | 0.32 |
ΔLV ESV (mL) | −8.4 ± 8.5 | −3.0 ± 7.1 | −6.4 ± 11.5 | 0.19 |
WMSI | 1.02 ± 0.1 | 1.04 ± 0.1 | 1.36 ± 0.4 | <0.0001 † |
ΔWMSI (peak stress to rest) | 0 | −0.02 ± 0.1 | 0.08 ± 0.2 | <0.05 † |
LVOT CO (L/min) | 11.3 ± 3.4 | 10.6 ± 2.4 | 9.5 ± 1.5 | 0.16 |
ΔLVOT CO (L/min) (peak stress to rest) | 5.6 ± 2.4 | 5.6 ± 2.3 | 3.9 ± 1.3 | <0.05 † |
E/A ratio | 1.7 ± 0.7 | 2.1 ± 1.7 | 2.4 ± 1.0 | 0.27 |
ΔE/A ratio (peak stress to rest) | −0.3 ± 0.7 | 0.2 ± 1.2 | 0.2 ± 1.0 | 0.31 |
E-dec (msec) | 110 ± 23 | 94 ± 42 | 99 ± 33 | 0.33 |
ΔE-dec (msec) (peak stress to rest) | −48 ± 28 | −75 ± 57 | −49 ± 53 | 0.15 |
IVRT (msec) | 23 ± 13 | 23 ± 15 | 31 ± 11 | 0.13 |
ΔIVRT (msec) (peak stress to rest) | −42 ± 16 | −45 ± 21 | −42 ± 25 | 0.90 |
E/e′ ratio | 10 ± 3 | 11 ± 5 | 13 ± 6 | 0.12 |
ΔE/e′ (peak stress to rest) | 1.6 ± 2.0 | 1.5 ± 3.5 | 1.0 ± 4.2 | 0.86 |
LV S′ mean (cm/sec) | 9.5 ± 2.0 | 8.4 ± 2.1 | 7.0 ± 2.4 | <0.01 † |
ΔLV S′ mean (cm/sec) (peak stress to rest) | 3.3 ± 1.8 | 2.6 ± 1.8 | 1.8 ± 1.7 | 0.05 |
LV GLS (%) | −22 ± 3 | −19 ± 3 | −12 ± 5 | <0.0001 † |
ΔLV GLS (%) (peak stress to rest) | −5.7 ± 2.0 | −3.3 ± 2.9 | −0.2 ± 2.8 | <0.0001 † |
LV GLS endocardial (%) | −26 ± 4 | −22 ± 4 | −15 ± 7 | <0.0001 † |
ΔLV GLS endocardial (%) (peak stress to rest) | −6.4 ± 2.5 | −3.8 ± 3.7 | −0.3 ± 3.7 | <0.0001 † |
LV GLS epicardial (%) | −18 ± 3 | −15 ± 3 | −10 ± 5 | <0.0001 † |
ΔLV GLS epicardial (%) (peak stress to rest) | −5.0 ± 1.7 | −2.6 ± 2.5 | −0.1 ± 2.5 | <0.0001 † |
LV TTP (msec) | 29 ± 11 | 32 ± 8 | 44 ± 15 | <0.001 † |
ΔLV TTP (msec) (peak stress to rest) | −10 ± 14 | −9±11 | −13 ± 17 | 0.77 |
Hemodynamics | ||||
Heart rate (beats/min) | 131 ± 16 | 133 ± 19 | 131 ± 15 | 0.94 |
Diastolic blood pressure (mm Hg) | 85 ± 15 | 90 ± 17 | 102 ± 17 | <0.01 † |
Systolic blood pressure (mm Hg) | 201 ± 30 | 203 ± 27 | 192 ± 35 | 0.50 |
Double product ∗ | 26,594 ± 6,168 | 27,132 ± 6,239 | 25,097 ± 5,981 | 0.61 |
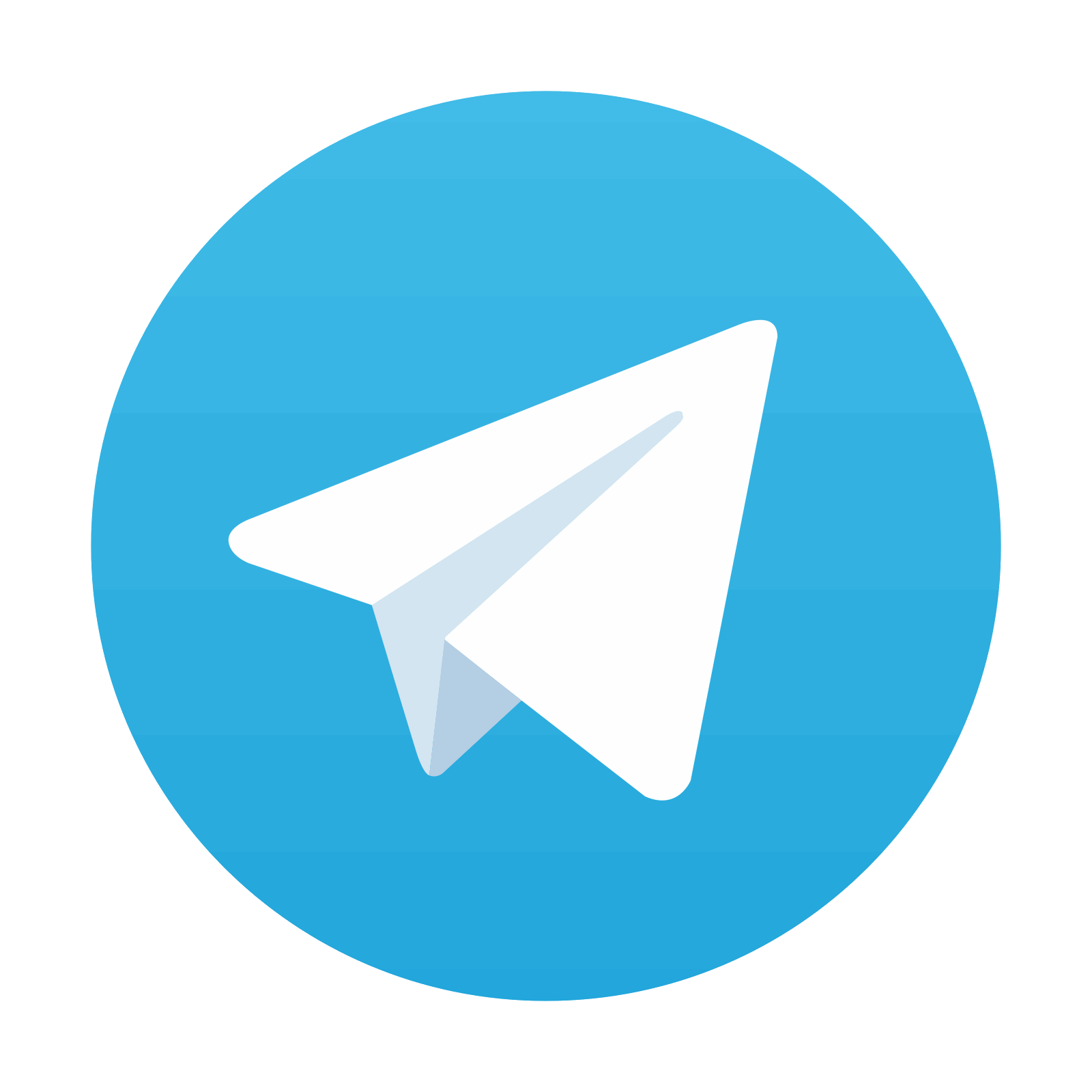
Stay updated, free articles. Join our Telegram channel

Full access? Get Clinical Tree
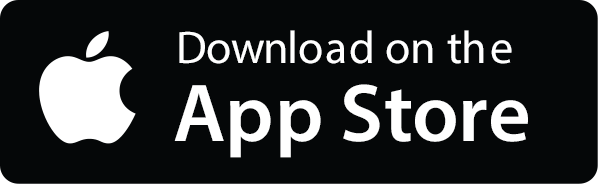
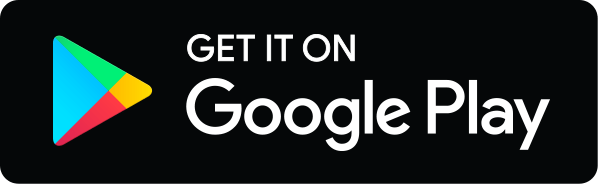
