Background
Given the complex morphologic nature of the right ventricle, three-dimensional (3D) approaches would be more appropriate for assessing right ventricular (RV) function than two-dimensional approaches. Thus, the investigators have developed a novel 3D speckle-tracking echocardiographic (STE) system specialized for the right ventricle. The aim of this study was to assess the characteristics of RV global and regional deformation as well as changes on stress tests using the 3D STE system in experimental studies.
Methods
In 10 sheep, sonomicrometry crystals were implanted to validate 3D STE data in the RV endocardium of seven RV segments, including the basal and mid anterior, lateral and inferior wall, and outflow free wall. Full-volume 3D STE data sets and sonomicrometric data were acquired at baseline, during pulmonary artery banding (PAB)–induced moderate (peak RV pressure > 40 mm Hg) and severe (peak RV pressure > 60 mm Hg) RV pressure increases, and during propranolol infusion. The 3D STE area change ratio (ACR), longitudinal strain (LS), and circumferential strain (CS) were measured, and RV global and all segmental deformation data were compared between baseline and stress tests. To assess clinical feasibility, 30 control subjects and 11 patients with pulmonary arterial hypertension were enrolled.
Results
All combined 3D STE data were significantly correlated with the sonomicrometric data (ACR, R 2 = 0.88; LS, R 2 = 0.84; CS, R 2 = 0.82; P < .001). In all seven segments, the 3D STE data correlated with the sonomicrometric data ( R 2 = 0.72–0.90, P < .001). Global ACR and LS data showed significant differences among baseline, moderate PAB, and severe PAB; however, CS differed only between baseline and severe PAB. The magnitudes of segmental deformation in the free wall were larger than those in the septum and apex under all conditions ( P < .05) except LS during severe PAB. Segmental analyses also showed similar responses during stress tests; the ACR in each segment differed significantly between conditions. In all but the apical segments, LS showed significant reductions from moderate PAB; in contrast, CS was significantly reduced with severe PAB in all segments. In this clinical study, the acquisition rate of adequate images for analysis of the RV outflow tract was lower (75.6%) compared with the rate in other segments (from 85.4% to 100%). However, the pulmonary arterial hypertension group had lower RV global deformation values than the control group (ACR and LS, P < .001; CS, P = .003), the ACR and LS in basal and middle segments differed significantly between groups, and the outflow and apex did not differ.
Conclusions
A novel 3D STE system specialized for the right ventricle is reliable for RV deformation analyses and may provide additional information about RV global and segmental function. The clinical feasibility of this system is acceptable.
Cardiopulmonary diseases may cause right ventricular (RV) dysfunction, and in recent years, many studies have confirmed the prognostic importance of RV function. Echocardiography is widely used to evaluate RV function using tricuspid annular plane systolic excursion and RV fractional area change. However, assessing RV function using conventional echocardiography is difficult because of the complex nature of the RV structure and contraction in contrast to the ellipsoidal left ventricle. As with the left ventricle, RV deformation imaging would provide additional information about RV pathophysiology. Two-dimensional (2D) speckle-tracking echocardiographic (STE) systems have been used to quantitate RV myocardial deformation, which could be assessed by longitudinal strain (LS) but not circumferential strain (CS), because of the complex nature of the RV structure in contrast to the ellipsoidal left ventricle. Thus, RV global and regional deformation has not been well studied noninvasively, because an appropriate system has not yet been developed.
Given the complex nature of the right ventricle, a three-dimensional (3D) approach would be more appropriate than a 2D approach. We developed a 3D STE system for the left ventricle, and the pathophysiology and clinical feasibility of various strain components as possible imaging biomarkers were subsequently studied. To overcome the limitation of 2D speckle-tracking echocardiography for assessing RV function, we recently reported on the preliminary use of a 3D STE system designed for left ventricular (LV) analysis and showed that 3D speckle-tracking echocardiography is useful for evaluating RV function with deformation data. Similarly, Smith et al . reported that 3D STE deformation data were associated with clinical outcomes in patients with pulmonary hypertension using the same system. However, the current 3D STE system, which is specialized for the left ventricle, may be inadequate for accurately demonstrating RV structure and function. Thus, we developed a new 3D STE system that features a specialized algorithm for the right ventricle and sought to assess the characteristics of RV global and regional deformation with the system in experimental studies and to confirm the clinical feasibility of evaluating global and regional deformation data in normal subjects and in a small group of patients with pulmonary arterial hypertension (PAH).
Methods
Animal Preparation
In this study, we used 10 male hybrid Suffolk sheep (Japan Lamb, Ltd, Hiroshima, Japan) weighing, on average, 27.5 kg. The study was approved by the Institutional Animal Experiment Committee of the University of Tsukuba and conducted in compliance with our university’s regulations for animal experiments and the “Fundamental Guideline for Proper Conduct of Animal Experiment and Related Activities in Academic Research Institutions” of the Ministry of Education, Culture, Sports, Science and Technology.
General anesthesia was induced with thiamylal sodium (10-15 mg/kg intravenously), and endotracheal intubation was performed. Anesthesia was maintained with isoflurane (1.5%-2%) and oxygen. Two 5-F micromanometer-tipped catheters (Millar Instruments, Houston, TX) were inserted into the right ventricle via the jugular vein and into the left ventricle via the femoral artery to measure the ventricular pressure and maximal rate increase in LV pressure (dP/dt max) for each condition. Pressure data were digitized and stored on a personal computer for analysis with dedicated software. A median sternotomy was performed, and an incision was made in the pericardium avoiding the apical area.
Sonomicrometry
We implanted sonomicrometry crystals (2-mm diameter; Sonometrics Corporation, London, ON, Canada) at the RV endocardium in seven segments, including the basal and mid anterior, lateral and inferior wall, and outflow free wall. (For detailed methods, see Supplemental Figure 1 .) Sonomicrometry crystals were implanted in four or five of the seven segments in each sheep, because they could not be implanted simultaneously in all segments.
Sonomicrometric data were acquired immediately after the corresponding echocardiographic images were recorded, and the data were analyzed using CardioSOFT Pro (Sonometrics Corporation). Strain was calculated as [ L ( t ) − L 0 ]/ L 0 , where L ( t ) is segment length at time t , and L 0 is segment length at the onset of the QRS complex. The LS of the basal segment was measured between the basal and mid endocardial crystals, whereas the LS of the mid segment was measured between the mid and apical endocardial crystals. The CS of the basal and mid segments was measured between each endocardial crystal pair. The area change ratio (ACR) was calculated using a function in CardioSOFT that can multiply two sonomicrometric curves. The ACR was calculated with a longitudinal and circumferential curve of each segment. All strain data were calculated by averaging data from 10 consecutive heartbeats. Any curves that were not recorded clearly were excluded from the analysis.
Echocardiography
Echocardiographic examinations were performed with an ARTIDA ultrasonography system (Toshiba Medical Systems, Tochigi, Japan). Full-volume electrocardiography-gated 3D data sets with six sectors were acquired in the apical position using a matrix-array 2.5-MHz transducer. In each study, the heart was repositioned within the apical pericardium to control for apical motion. A peritoneal incision was made to acquire the appropriate 3D apical images through the diaphragm. The animal’s breathing was stopped during the image acquisition process. The volume rate of each image was set at 30 to 40 Hz. The data were stored and transferred to a computer (Inspiron 1300; Dell, Inc, Round Rock, TX) for offline analysis. The images were analyzed with prototype software for the right ventricle.
We used a 3-MHz transducer for conventional echocardiography. LV volume and ejection fraction were measured using the modified Simpson’s rule. RV diameter and function were determined with RV diameter (length, papillary muscle, and basal level), fractional area change, tricuspid annular plane systolic excursion, and peak systolic velocity of the tricuspid annulus using the tissue Doppler method.
Three-Dimensional Wall Motion Tracking Algorithm
First, the tracking points were distributed on the 3D curved surfaces of the RV myocardium at the end of the diastolic phase, which were estimated by traced lines on multiplanar reconstruction images ( Figure 1 ). In the template-matching process, the template volume in the current frame was generated from an approximately 10 × 10 × 10 mm cube. The most similar point in the next volume was found by the comparison of a template volume with the cube in the next volume. We used the 3D sum of squared differences method to test image similarity. Finally, the motion vectors were interpolated using a 3D interpolation algorithm. After these steps, arbitrary points of interest on the cardiac wall could be tracked by integrating the interpolated motion vectors over all frames during a single cardiac cycle. We visually identified tracking quality according to the tracking quality of the endocardial trace line on the multiplanar reconstruction images. The 3D RV images were then automatically obtained and divided into a total of 14 segments, including five basal, five middle, two apical, and two RV outflow ( Figure 2 ). All strain data were measured on a single heartbeat. The temporal changes in the strain data were displayed as a color-coded “plastic bag” image as well as time-strain curves including global and segmental RV strain ( Figure 3 ). The surrogate point used to measure deformation data was set at the minimum point (maximum deformation point) of the global deformation data on the strain-time curve ( Figure 3 ).



Experimental Protocol
After the baseline measurements were recorded, partial ligations of the main pulmonary artery were performed to increase RV afterload in two stages. The first stage caused moderately increased afterload (moderate pulmonary artery banding [M-PAB]), such that RV systolic pressure increased by ≥40 mm Hg. The 3D echocardiographic and sonomicrometric data during M-PAB were recorded about 5 min after pulmonary artery banding (PAB) to obtain data during stable condition. After a 10-min rest period, we confirmed that hemodynamics and RV function recovered to the baseline. Then, RV systolic pressure increased to ≥60 mm Hg, leading to severely increased afterload (severe PAB [S-PAB]). The data acquisitions during S-PAB were performed immediately after RV pressure exceeded 60 mm Hg, as hemodynamic collapse would occur in a few minutes. After a 10-min rest period, we confirmed that hemodynamics and RV function recovered to the pre–propranolol infusion test baseline. Next, a continuous infusion of propranolol (4-8 μg/kg/min) was initiated to reduce contractility. The infusion dose was increased and maintained until the RV dP/dt max had decreased by ≥25% compared with that at baseline. The 3D echocardiographic and sonomicrometric data were recorded during the steady administration of propranolol.
Reproducibility
All the baseline data were used to assess intra- and interobserver reproducibility. To test intraobserver variability, a single observer analyzed the data on two occasions with a 1-month interval. To test interobserver variability, a second observer analyzed the data without knowledge of the first observer’s measurements. Reproducibility was assessed using the mean absolute percentage error (absolute difference divided by the mean of the two observations).
Clinical Studies
The 3D and 2D echocardiographic examinations were performed in the same setting as the experimental studies. Thirty normal subjects and 11 patients with PAH were enrolled. Normal subjects were healthy volunteers, while patients with PAH were previously diagnosed according to current guidelines. Subjects with atrial fibrillation or frequent premature beats were excluded. We assessed the acquisition rate of adequate images by confirming clearly defined endocardial contours in each segment as well as 3D STE parameters for comparing normal subjects and patients with PAH. The study was approved by an institutional review committee, and all subjects provided informed consent.
Statistical Analysis
Data are shown as mean ± 95% CI. The data were statistically analyzed using linear regression and Bland-Altman analysis to determine the bias and limits of agreement between the modalities. A mixed-model analysis was used to compare results among variables at baseline and during M-PAB and S-PAB. When significant intergroup differences were present, a Bonferroni test was performed to compare individual groups. A paired t test was used to compare the baseline and propranolol infusion results. In addition, mixed-model analyses were performed to determine the differences among segments at same condition.
P values < .05 were considered to indicate statistical significance. We used SPSS version 22 for Mac (SPSS, Inc, Chicago, IL) for the statistical analyses.
Results
Table 1 summarizes the hemodynamic and conventional echocardiographic data. RV systolic pressure ranged from 41.6 to 56.4 mm Hg during M-PAB and from 60.2 to 69.8 mm Hg during S-PAB. During propranolol infusion, RV dP/dt max was significantly reduced compared with baseline (changes in RV dP/dt; range, −29.1% to −63.6%). RV dimensions increased and LV volume decreased during S-PAB. Fractional area change and tricuspid annular plane systolic excursion were reduced during S-PAB compared with baseline and M-PAB; however, they did not differ between baseline and M-PAB. Peak systolic velocity of the tricuspid annulus was reduced in each stress test compared with baseline; however, it did not differ between M-PAB and S-PAB.
Variable | Baseline | M-PAB | S-PAB | P value, ANOVA | Propranolol infusion | P value, base vs propranolol infusion |
---|---|---|---|---|---|---|
HR (beats/min) | 110.4 ± 3.4 | 109.8 ± 5.2 | 105.2 ± 5.0 ∗ † | <.001 | 92.8 ± 5.6 | .001 |
LVSP (mm Hg) | 82.3 ± 9.0 | 76.6 ± 9.0 | 50.6 ± 9.0 ∗ † | <.001 | 60.8 ± 9.0 | .004 |
LV dP/dt | 2,050 ± 223 | 1,597 ± 268 | 1,031 ± 196 ∗ † | <.001 | 1,183 ± 178 | .001 |
RVSP (mm Hg) | 22.1 ± 2.9 | 47.4 ± 3.4 ∗ | 63.2 ± 2.2 ∗ † | <.001 | 22.1 ± 2.4 | .99 |
RV dP/dt | 679.8 ± 46.2 | 542.2 ± 36.6 ∗ | 449.7 ± 52.4 ∗ † | <.001 | 350.3 ± 35.2 | <.001 |
LVEDV (mL) | 26.6 ± 4.8 | 24.7 ± 4.6 | 15.7 ± 4.2 ∗ † | .004 | 28.1 ± 5.0 | .71 |
LVESV (mL) | 6.8 ± 2.0 | 5.9 ± 1.8 | 3.7 ± 1.9 ∗ | <.001 | 9.8 ± 1.8 | .10 |
LVEF (%) | 75.6 ± 3.9 | 77.0 ± 4.3 | 77.6 ± 4.2 | <.001 | 65.3 ± 4.2 | .01 |
RV-long (mm) | 42.3 ± 3.3 | 40.1 ± 3.6 | 46.3 ± 3.8 ∗ † | .045 | 42.8 ± 3.4 | .81 |
RV-PM (mm) | 17.9 ± 2.2 | 16.9 ± 2.3 | 23.8 ± 2.8 ∗ † | .001 | 19.5 ± 2.1 | .88 |
RV-base (mm) | 22.7 ± 2.5 | 23.1 ± 2.5 | 26.1 ± 2.6 ∗ | .02 | 25.5 ± 2.1 | .13 |
FAC (%) | 63.2 ± 9.0 | 54.4 ± 8.9 | 40.7 ± 8.3 ∗ † | .23 | 52.4 ± 10.1 | .07 |
TAPSE (mm) | 8.8 ± 0.9 | 7.2 ± 1.5 | 5.2 ± 0.9 ∗ † | .001 | 7.0 ± 1.2 | .03 |
TA S′ (cm/sec) | 10.4 ± 1.4 | 7.5 ± 1.8 ∗ | 4.7 ± 1.4 ∗ | <.001 | 6.7 ± 1.4 | .03 |
Three-Dimensional Speckle-Tracking Echocardiography versus Sonomicrometry
One hundred twenty data sets were available for analysis of the correlation of strain data between sonomicrometry and 3D speckle-tracking echocardiography. Figure 4 shows the correlations between sonomicrometry and the 3D STE data. Each parameter was strongly correlated between the two methods. In the Bland-Altman plots, the fixed bias and 95% limits of agreement were −0.38% and −6.56% to 6.18% for the ACR, −0.77% and −5.67% to 4.90% for LS, and −0.98% and −6.24% to 5.26% for CS, respectively. In each segment, the 3D STE data were significantly correlated with the sonomicrometric data ( Table 2 ).
Variable | Basal | Mid | Outflow | ||||
---|---|---|---|---|---|---|---|
Anterior | Lateral | Inferior | Anterior | Lateral | Inferior | ||
ACR | 0.88 | 0.81 | 0.90 | 0.86 | 0.85 | 0.90 | 0.88 |
LS | 0.88 | 0.86 | 0.79 | 0.82 | 0.81 | 0.90 | 0.83 |
CS | 0.86 | 0.72 | 0.85 | 0.81 | 0.79 | 0.77 | 0.86 |
Changes in RV STE Data Induced by Stress Tests
A representative case is shown in Figure 3 , showing the dramatic changes to the RV strain profile induced by S-PAB. The magnitudes of RV global ACR and LS during M-PAB and S-PAB were significantly lower than those at baseline ( Figure 5 ). For a detailed description of the changes in 3D STE RV segmental deformation data by stress tests, see Supplemental Figure 2 .
Reproducibility
Intra- and interobserver reproducibility was 6.9% and 7.7%, respectively, for the ACR measurements; 8.6% and 11.1%, respectively, for the LS measurements; and 6.0% and 8.6%, respectively, for the CS measurements.
Clinical Study
Table 3 shows the baseline characteristics and echocardiographic data. The number (proportion) of cases for which 3D echocardiographic image quality was suitable for speckle-tracking analysis was low in the outflow segments at 31 cases (75.6%) in the outflow free wall and outflow septum ( Table 4 ). In the remaining segments, the lowest rate of feasibility was observed in the mid anterior wall in the control group (83.3%). The magnitude of global strain was significantly lower in the PAH group than in the normal control group (ACR, −26.7 ± 4.0% vs −12.2 ± 3.3%, P < .001; LS, −12.2 ± 3.3% vs −5.8 ± 3.0%, P < .001; CS, −10.1 ± 3.6% vs −4.6 ± 2.4%, P < .001). In all the basal and mid segments, the magnitudes of ACR, LS, and CS in the PAH group were significantly lower than those in the control group. No significant differences in apical and outflow free wall segments were common to all of the deformation data.
Variable | Normal | PAH | P |
---|---|---|---|
( n = 30) | ( n = 11) | ||
Age (y) | 49.1 ± 17.2 | 47.0 ± 13.5 | .74 |
Sex (male) | 18 (60%) | 4 (36.4%) | .49 |
BSA (m 2 ) | 1.58 ± 0.16 | 1.51 ± 0.16 | .77 |
SBP (mm Hg) | 124.8 ± 17.2 | 118.1 ± 16.0 | .19 |
DBP (mm Hg) | 71.1 ± 16.4 | 71.8 ± 10.3 | .91 |
HR (beats/min) | 66.1 ± 11.6 | 81.5 ± 14.6 | .01 |
RVSP by echocardiography (mm Hg) | 22.9 ± 5.5 ( n = 27) | 83.5 ± 36.1 | <.001 |
LVEDV (mL) | 73.0 ± 17.2 | 48.2 ± 20.3 | .001 |
LVESV (mL) | 23.9 ± 6.4 | 13.8 ± 13.8 | .01 |
LVEF (%) | 66.8 ± 6.1 | 73.1 ± 5.2 | .004 |
RV-long (mm) | 62.8 ± 9.2 | 77.7 ± 8.4 | <.0001 |
RV-PM (mm) | 28.1 ± 5.2 | 40.7 ± 8.1 | <.0001 |
RV-base (mm) | 31.3 ± 5.4 | 44.8 ± 8.5 | <.0001 |
FAC (%) | 48.9 ± 4.2 | 27.9 ± 7.8 | <.0001 |
TAPSE (mm) | 21.0 ± 2.3 | 15.5 ± 3.7 | .001 |
TA S′ (cm/sec) | 13.3 ± 1.7 | 10.3 ± 2.2 | .003 |
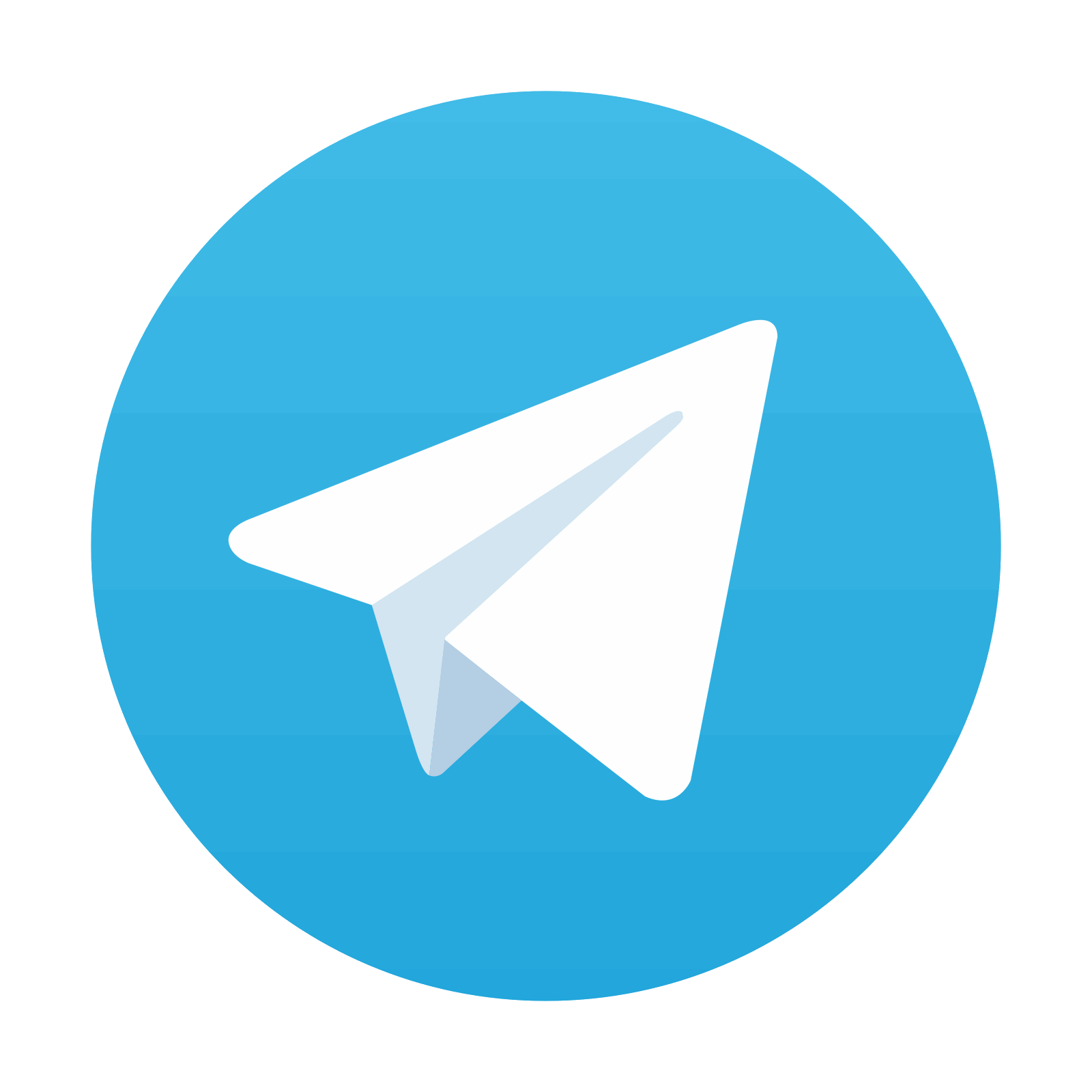
Stay updated, free articles. Join our Telegram channel

Full access? Get Clinical Tree
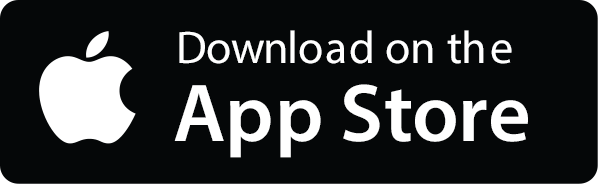
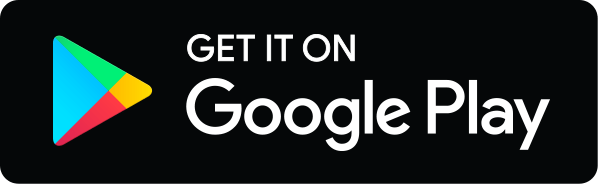
