Statin drugs are leading medication prescribed for treatment of dyslipidemic patients aimed at preventing both primary and secondary incidences of atherosclerosis-related cardiovascular events. Statin drugs competitively inhibit HMG-CoA reductase enzyme activity, thereby inhibiting cell-mediated cholesterol synthesis and reducing the low-density lipoprotein (LDL) cholesterol concentration of plasma. Conversely, the mechanism by which statins increase high-density lipoprotein (HDL) cholesterol concentration of plasma is not well understood. The plaque array method was used to examine the effect of statins on in vitro cholesterol particle formation. We observed that statins induced high-density cholesterol particle formation in buffer solution with or without the addition of human serum. Besides, simvastatin and lovastatin in their inactive pro-drug forms modulate formation of LDL and HDL cholesterol particles, indicating a novel nonenzymatic mechanism of statins. In a pilot study, screening of serum samples in the assay showed variation among patient samples in response to different statins. Specifically, screening of 50 serum samples with high cholesterol and statin treatment, compared with standard LDL-based measurement of statin efficacy, showed a good correlation for simvastatin (88%) and atorvastatin (84%). Taken together, our data indicate that statins, in addition to inhibiting enzyme-mediated cholesterol synthesis, have the capability to nonenzymatically modulate formation of LDL and HDL cholesterol particles in vitro. Similar interactions occurring in serum may provide a means to alter cholesterol particle formation in vivo.
Numerous clinical and epidemiologic studies have demonstrated that both very low–density lipoprotein and low-density lipoprotein (LDL) cholesterols promote atherosclerotic plaque formation, whereas the high-density lipoprotein (HDL) cholesterol play an atheroprotective role mitigating plaque-induced damages to the blood vessel wall. In addition, a reduction in plasma levels of HDL cholesterol is identified as an independent risk factor for developing atherosclerosis-related CVD. Statin therapy significantly reduces LDL cholesterol, and its effect on increasing the HDL cholesterol in the plasma varies among patients. Besides, the underlying mechanism by which statin induced increases in the HDL concentration is not well understood. It is possible an independent mechanism of statin, not related to inhibition of HMG CoA reductase, may play a role in increasing HDL in the plasma.
Among various statins, simvastatin and lovastatin are administrated as inactive prodrugs, and they are converted in vivo into active metabolites by liver microsomal CYP3A4 and CYP3A5 enzymes. These enzymes hydrolyze the lactone ring of simvastatin producing active metabolites that competitively inhibit HMG-CoA reductase enzyme. The HMG-CoA reductase enzyme inhibitory constant (K i ) values for statins range from 2 to 250 nM, whereas the K m value for natural substrate HMG-CoA is 4 μM concentrations. It is observed that 5% to 20% of patients treated with statins show intolerance to these drugs causing adverse side effects including muscle syndromes.
Statins are known to possess pleiotrophic biologic effect and are prescribed extensively to treat patients with CVD. Thus, a further understanding of additional mechanisms of action is warranted. We recently reported the development of a plaque array method that uses flow cytometry in combination with mass spectrometry for the detection, isolation, and proteomics analysis of serum-derived amyloid and cholesterol particles. In the present study, we applied the plaque array method to evaluate the effect of statins on in vitro cholesterol particle formation. Using this method, we uncovered a novel nonenzymatic mechanism by which statins may modulate low-density lipoprotein particle (LDL-P) and high-density lipoprotein particle (HDL-P) formation.
Methods
Lyophilized powder of TopFluor fluorescent cholesterol was purchased from Avanti Polar Lipids (Alabaster, Alabama). Powders of atorvastatin, simvastatin, fluvastatin, and lovastatin were purchased from Cayman Chemicals (Ann Arbor, Michigan). Simvastatin acid sodium salt (SVA) control was purchased from Toronto Research Chemicals (North York, Ontario, Canada). Reagents for measuring serum levels of apolipoprotein A, apolipoprotein B, LDL, HDL, C-reactive protein, glucose, cholesterol, and triglycerides were purchased from Roche Diagnostics (Indianapolis, Indiana). Fifty serum samples (25 simvastatin treated and 25 atorvastatin treated) collected from patients with high cholesterol receiving statin therapy from 24 to 36 months were purchased from BioServe (Beltsville, Maryland). Serum samples of patients with atherosclerotic lesions and a history of myocardial infarction, coronary stent insertion, and CT scan–positive lesions were obtained from the Palo Alto Medical Foundation (Palo Alto, California) after Institutional Review Board approval. Serum samples from normal subjects were obtained from Dx Biosamples (San Diego, California) and the Stanford Blood Center, Stanford University (Palo Alto, California).
Fluorescent-labeled lyophilized TopFluor cholesterol (1 mg) (Ex/Em = 495 nm/507 nm) powder was solubilized in 1 ml of 100% alcohol. After centrifugation for 5 minutes at 2,040 × g , the supernatants were used as stock solution. From this stock solution, 5 μg was diluted in phosphate-buffered saline (PBS) and analyzed by flow cytometer for the presence of insoluble particles. Solutions containing soluble cholesterol aggregates with no fluorescence-positive particles or containing fewer than 300 particles/ml were used in the assay.
Powders (2 mg) of simvastatin (inactive pro-drug), simvastatin acid (active form), lovastatin (inactive pro-drug), and atorvastatin were individually solubilized in 1 ml of 100% alcohol. After centrifugation for 5 minutes at 2,040 × g , the supernatants were used as stock solution. From this stock solution, 2 doses (2.5 and 5 μM) were used for incubation with fluorescent-labeled cholesterol aggregates to examine statin-induced effect on formation of cholesterol particles. Similarly, powder (2 mg) of fluvastatin was solubilized in 1 ml of deionized water to make stock solution of 2 mg/ml. After centrifugation for 5 minutes at 2,040 × g , the supernatants were used to study its effect on formation of cholesterol particles. Separately, for use as positive control in the assay, we prepared the self-formed insoluble cholesterol particles by incubating 5 μg of soluble fluorescent cholesterol aggregates at 37°C for 36 hours. The self-formed cholesterol particles were obtained by in vitro conversion of the soluble fluorescent-cholesterol aggregates into insoluble cholesterol particles in the PBS without addition of serum.
To examine the effect of statin on low-density cholesterol-like particles (LD-CP) and high-density cholesterol-like particles (HD-CP) in vitro formation, 2 doses (2.5 and 5 μM) of atorvastain, simvastatin, simvastatin acid, lovastatin, and fluvastatin were individually added in 200 μl of PBS followed by the addition of 5 μM soluble fluorescent-labeled cholesterol aggregates. The samples were incubated at 37°C with agitation at 200 rpm/min for 45 minutes. For negative control, each statin (5 μM) was incubated in PBS buffer without fluorescent-labeled cholesterol aggregates. After incubation, 200 μl of PBS was added to the mixture, and the resulting samples were acquired by flow cytometry (FACScan; BD Biosciences, San Jose, California). The samples were acquired using settings with excitation 488 nm and emission detection at 540/20 nm.
Serum levels of analytes, such as LDL, HDL, cholesterol, apolipoprotein B, apolipoprotein A, glucose, C-reactive protein, and triglycerides, were measured using Roche Cobas Integra 400 plus chemistry analyzer (Roche Diagnosis). Abnormal concentration of the analytes was defined based on their concentration identified beyond the upper and lower reference values for each analyte as recommended by the supplier (Roche Diagnosis). Serum samples identified for having abnormal value for ≥1 analytes were selected for screening against statins.
A total of 80 serum samples were selected for testing statins effect on LDL-P and HDL-P formation. Of the 80 serum samples, 50 were selected from high cholesterol and the subjects were on statin treatment, whereby LDL cholesterol results were obtained at baseline and after a period of 2 to 3 years of statin treatment. The remaining 30 samples were identified for having abnormal profile for ≥1 analytes that are related to diagnosis of CVD. Each serum sample was tested against simvastatin, atorvastatin, fluvastatin, and lovastatin. Our preliminary screening of serum samples using simvastatin showed that dose ranges from 1 to 5 μM had detectable effect on modulating LDL-P and HDL-P formation (data not shown). Based on this observation, 5 μM dose of statin concentration was selected to screen all serum samples. Accordingly, in a 200-μl reaction using PBS, diluted serum sample (2.5%) was mixed with each statin (5 μM) followed by addition of fluorescent-labeled cholesterol aggregates (2 μM). For control experiment without statin, diluted serum samples were mixed with fluorescent cholesterol aggregates. The assays were performed in triplicate wells in a 96-well protein nonbinding microtiter plate (Greiner-Bio one, Monroe, North Carolina), and the assay plate was incubated in a thermoshaker at 37°C, 200 rpm/min for 45 minutes. After incubation, the plate was loaded into Guava EasyCyte 8HT flow cytometer (EMD Millipore, Hayward, California) for sample acquisition. The acquisition setting in the flow cytometer was adjusted to count 2,000 particles or 60 seconds with flow rate of 0.6 μl/second, and sample fluorescence was measured using 488 nm for excitation and 540/20 nm for emission.
After the sample acquisition, to calculate LDL and HDL cholesterol particles in the samples, InCyte software (EMD Millipore, Hayward, California) was used. The concentration of LDL-P and HDL-P in the samples was determined by absolute counts by the Guava EasyCyte 8HT System. We established the lower and upper detection limit (500 to 60,000 particles/ml) of cholesterol particles using this system. Two gates were drawn in the regions of LDL-P and HDL-P identified in the histogram, and both percentage and concentration of LDL-P and HDL-P were calculated. To determine statin-induced increase in HDL-P for each statin, first, the percentage of HDL-P1 was calculated for control assay performed in the absence of statin; second, percentage of HDL-P2 was calculated for the same sample performed in the presence of each statin; and finally, the value of HDL-P2 was subtracted from HDL-P1 value to determine the percentage of HDL-P gain for each serum sample. Among triplicate values obtained for each sample, the LDL-P and HDL-P percentage within ±2 SD range was accepted for calculation of the statin effect.
Results
The plaque array is a functional biochemical assay developed to examine plaque particle formation in vitro by incubating soluble plaque-forming oligomer/aggregates with serum samples. In the assay, the soluble plaque-forming aggregates/oligomer act as “substrate” and serum components act as “catalyzer” converting the soluble plaque components into insoluble plaque particles. During the sample acquisition process by flow cytometry, the soluble plaque-forming oligomer/aggregates are not detected, whereas the end products, insoluble plaque particles, are detected and enumerated. In our previous study using plaque array method, we observed that fluorescently labeled soluble cholesterol aggregates when incubated with serum causes formation of 2 major fractions of cholesterol particles.
In the present study, to analyze the effect of statins on formation of cholesterol particles, fluorescent-labeled cholesterol aggregates were incubated with lovastatin (pro-drug) in PBS buffer. Interestingly, it was observed that cholesterol aggregates incubated with lovastatin showed increases in the formation of HD-CP and relative reduction in LD-CP ( Figure 1 ). In the positive control, self-formed insoluble cholesterol particles showed LD-CP and HD-CP. In the negative control, lovastatin incubated in PBS showed a few particles in the fluorescent plot. This result suggests that lovastatin in the pro-drug form can modulate formation of cholesterol particles.
To further examine the statin effect, fluorescent-labeled cholesterol aggregates were incubated with simvastatin (pro-drug) and simvastatin acid (active form) separately. Analysis of the resultant samples showed a dose-dependent increase in HD-CP and relative reduction in LD-CP formation in the buffer for both simvastatin and simvastatin acid ( Figure 2 ). Expanding this observation, the assay was repeated using atorvastatin (active form) and fluvastatin (active form). Analysis of the resulting samples showed that atorvastatin induced HD-CP formation, whereas no significant increase in HD-CP formation was observed with fluvastatin ( Figure 3 ).
The preceding results were obtained from assays performed in buffer solution in the absence of serum. Hence, in the next step, we examined the effect of statins on LDL-P and HDL-P formation in the presence of serum samples. In a pilot study, 50 serum samples were selected for screening in this cholesterol particle formation assay, and these samples were identified for high cholesterol levels and for those patients who underwent either atorvastatin or simvastatin treatment. Each serum sample was screened against simvastatin, atorvastain, fluvastatin, and lovastatin. In the control experiment, each serum sample was analyzed for LDL-P and HDL-P formation without statin. Examples of screening of 3 serum samples in the formation of cholesterol particles assay using simvastatin are shown in Figure 4 . Analysis of the results showed diversity among the serum samples in response to simvastatin, atorvastain, fluvastatin, and lovastatin. Based on the standard LDL cholesterol measurement of the serum samples collected from statin therapy, the statin efficacy was ranked into 3 categories (no or low response 0% to 10%, moderate response 11% to 60%, and high response 61% to 100%). This established response ranges for simvastatin and atorvastatin was compared with their effect on increasing HDL-P formation in our assay.
First, for 25 serum samples collected from simvastatin treatment, following the standard LDL measurement method, 6 showed no or low response, 13 with moderate response, and 6 with high response. In a direct comparison with our study demonstrating simvastatin effect, we found 23 of 25 (88%) correlation with standard LDL-based measurement of statin efficacy ( Table 1 ). Of the 2 subjects treated with simvastatin who did not agree with the prediction made by the cholesterol particle formation assay, 1 patient showed a medium reduction in LDL cholesterol (PGN4-SIM). Our assay predicted that none of the statins would have been effective (simvastatin, atorvastatin, fluvastatin, and lovastatin). The other patient showed a high reduction in LDL cholesterol (PGN10-SIM). Although our assay predicted no response to simvastatin, atorvastatin, and fluvastatin, a medium response was predicted by use of lovastatin.
Serum sample | After statin LDL mg/dL | Baseline LDL mg/dL | LDL Serum response | Cholesterol particles formation without statin | Simvastatin induced HDL-P gain | Atorvastatin induced HDL-P gain | Fluvastatin induced HDL-P gain | Lovastatin induced HDL-P gain | |
---|---|---|---|---|---|---|---|---|---|
LDL-P | HDL-P | ||||||||
PGN1-SIM | 408 | 352 | 0 (%) | 85 (%) | 6 (%) | 6 (%) | 0 (%) | 0 (%) | 1 (%) |
PGN2-SIM | 177 | 238 | 25 (%) | 91 (%) | 8 (%) | 41 (%) | 16 (%) | 0 (%) | 55 (%) |
PGN3-SIM | 165 | 260 | 37 (%) | 85 (%) | 11 (%) | 41 (%) | 12 (%) | 4 (%) | 40 (%) |
PGN4-SIM | 219 | 253 | 13 (%) | 84 (%) | 3 (%) | 6 (%) | 0 (%) | 3 (%) | 8 (%) |
PGN5-SIM | 188 | 452 | 58 (%) | 87 (%) | 10 (%) | 38 (%) | 20 (%) | 0 (%) | 13 (%) |
PGN6-SIM | 106 | 246 | 57 (%) | 88 (%) | 11 (%) | 30 (%) | 4 (%) | 0 (%) | 38 (%) |
PGN7-SIM | 238 | 139 | 0 (%) | 84 (%) | 13 (%) | 3 (%) | 0 (%) | 3 (%) | 52 (%) |
PGN8-SIM | 161 | 258 | 38 (%) | 94 (%) | 4 (%) | 60 (%) | 6 (%) | 0 (%) | 46 (%) |
PGN9-SIM | 230 | 177 | 0 (%) | 82 (%) | 16 (%) | 2 (%) | 20 (%) | 0 (%) | 7 (%) |
PGN10-SIM | 68 | 280 | 76 (%) | 81 (%) | 19 (%) | 9 (%) | 6 (%) | 0 (%) | 50 (%) |
PGN11-SIM | 85 | 230 | 63 (%) | 76 (%) | 23 (%) | 66 (%) | 43(%) | 4 (%) | 63 (%) |
PGN12-SIM | 112 | 231 | 52 (%) | 78 (%) | 21 (%) | 42 (%) | 20 (%) | 19 (%) | 59 (%) |
PGN13-SIM | 74 | 234 | 68 (%) | 82 (%) | 16 (%) | 63 (%) | 27 (%) | 13 (%) | 65 (%) |
PGN14-SIM | 223 | 231 | 3 (%) | 84 (%) | 16 (%) | 8 (%) | 3 (%) | 21 (%) | 18 (%) |
PGN15-SIM | 101 | 260 | 61 (%) | 72 (%) | 25 (%) | 70 (%) | 32 (%) | 21 (%) | 64 (%) |
PGN16-SIM | 185 | 271 | 32 (%) | 72 (%) | 26 (%) | 20 (%) | 10 (%) | 6 (%) | 25 (%) |
PGN17-SIM | 126 | 320 | 61(%) | 89 (%) | 10 (%) | 65 (%) | 61 (%) | 33 (%) | 70 (%) |
PGN18-SIM | 83 | 245 | 67 (%) | 81(%) | 18 (%) | 61(%) | 20 (%) | 20 (%) | 65 (%) |
PGN19-SIM | 190 | 240 | 21 (%) | 74 (%) | 24 (%) | 40 (%) | 18 (%) | 30 (%) | 61 (%) |
PGN20-SIM | 350 | 380 | 8 (%) | 90 (%) | 9 (%) | 5 (%) | 11(%) | 3 (%) | 8 (%) |
PGN21-SIM | 158 | 235 | 33 (%) | 70 (%) | 27 (%) | 42 (%) | 12 (%) | 7 (%) | 55(%) |
PGN22-SIM | 156 | 210 | 26 (%) | 83(%) | 16 (%) | 38 (%) | 14 (%) | 7 (%) | 25(%) |
PGN23-SIM | 266 | 288 | 8(%) | 76 (%) | 20 (%) | 17 (%) | 12 (%) | 14 (%) | 9 (%) |
PGN24-SIM | 125 | 269 | 54 (%) | 87 (%) | 11 (%) | 41(%) | 16 (%) | 6 (%) | 45 (%) |
PGN25-SIM | 158 | 232 | 32 (%) | 70 (%) | 17 (%) | 45 (%) | 40 (%) | 9 (%) | 50 (%) |
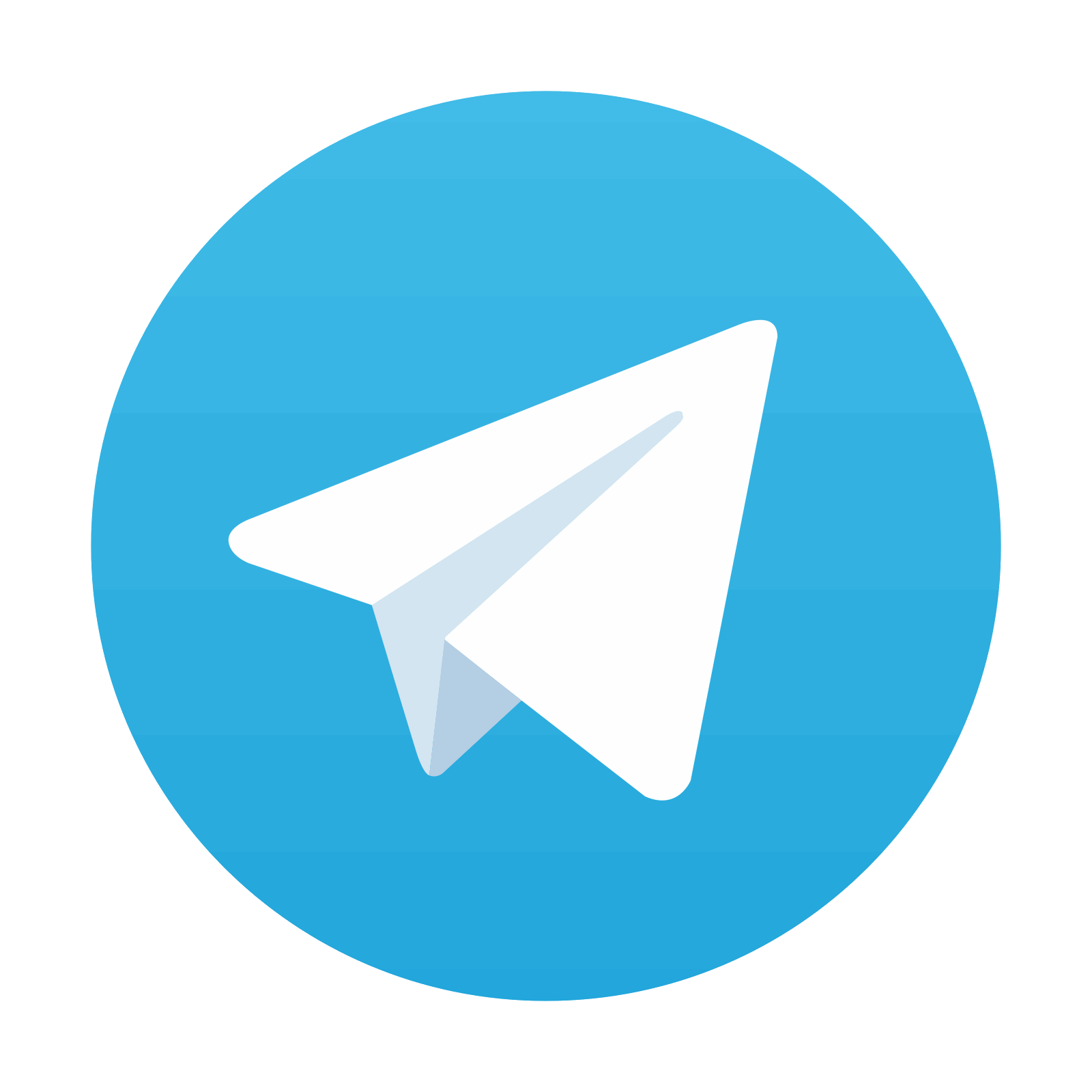
Stay updated, free articles. Join our Telegram channel

Full access? Get Clinical Tree
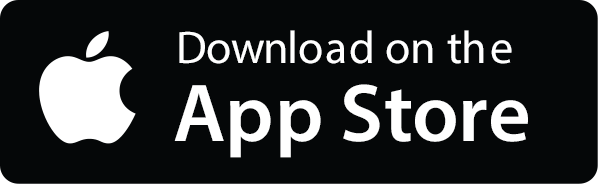
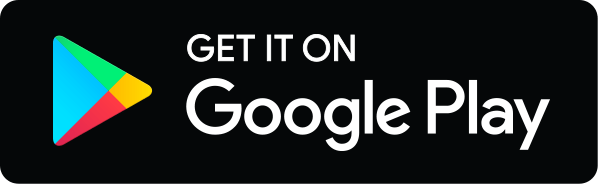
