Fig. 4.1
a and b: Top panel illustrates a 2-min period of breathing during REM sleep in a patient with OHS characterized by repetitive obstructive events and severe nocturnal desaturation. Upper airway obstruction is a common finding in OHS. In contrast, the bottom panel for another patient shows REM hypoventilation with sustained hypoxemia in the absence of identifiable hypopneic events. SpO 2 —oxygen saturation measured by pulse oximetry; TcCO 2 —transcutaneous carbon dioxide
At least moderate OSA is seen in the majority of individuals with OHS [11, 66, 93]. Although AHI has been identified as a significant predictor of hypercapnia by a number of studies [19, 21, 37], it was unclear how these events during sleep could lead to carbon dioxide retention during wakefulness, or why only a minority of patients even with severe OSA developed chronic hypoventilation. Data collected over the past few years has led to the development of a model describing a potential two-stage process which may explain this phenomenon [94]. The first stage involves the development of acute carbon dioxide retention during sleep. Accumulation of carbon dioxide occurs during apneic and hypopneic events, with the degree of carbon dioxide unloading that then occurs being dependent on the length of the interapneic period. If periods of abnormal breathing are prolonged and/or the time interval available to clear carbon dioxide is short, or respiratory efforts are reduced, then a net accumulation of carbon dioxide could occur [95–97]. In response to this, there would be a gradual compensatory increase in bicarbonate during sleep. However, during wakefulness, apnea disappears and carbon dioxide along with bicarbonate would return back to baseline levels. The next stage in the process occurs if there is insufficient time or ability of the individual to clear the bicarbonate load during wakefulness. The following sleep cycle would then commence with a slightly raised carbon dioxide level. Through modeling studies of whole body kinetics, Norman and colleagues [98] demonstrated that when the ventilatory response to carbon dioxide and renal bicarbonate excretion were normal, no change in either PaCO2 or bicarbonate occurred. However, if the carbon dioxide response was abnormally low or renal bicarbonate excretion inadequate, modest rises in carbon dioxide were seen over time. Most notably, when both situations arose together, the impact on carbon dioxide increase over time was synergistic [94, 98] (Fig. 4.2). This model highlights the various elements that need to be in play at any time for the development of awake hypercapnia in patients with upper airway obstruction, and helps to explain why only a portion of obese patients with OSA may develop hypercapnia.


Fig. 4.2
The potential link between upper airway obstruction at night and the development of chronic daytime hypercapnic respiratory failure. During apneic and hypopneic events, carbon dioxide loading occurs which is then unloaded during the interevent period. However, the ability to eliminate carbon dioxide is influenced by the duration of the apneic relative to the interevent period [95] and the magnitude of ventilation during the postevent breathing period [97]. These factors can produce an acute rise in carbon dioxide overnight which is compensated for by bicarbonate. The transition to chronic hypercapnia requires the persistence of elevated bicarbonate levels or a reduction in respiratory drive, or both [94, 98]. (From Borel et al., Respirology 2012, with permission)
The model proposed by Norman and colleagues [98] is also consistent with clinical findings. In hypercapnic OSA patients, significant overnight rises in transcutaneous carbon dioxide occur which are not seen in those with eucapnia, with these nocturnal rises significantly influencing early morning carbon dioxide levels [99]. Elevated bicarbonate is known to blunt the HCVR in OHS patients [100], and lower awake HCVR is associated with a higher proportion of time spent hypoventilating during REM sleep [66]. Even mildly raised daytime serum bicarbonate levels (> 27 mEq/L) have been shown to be a sensitive indicator for the presence of hypercapnia in patients with morbid obesity [21].
Changes in breathing related to upper airway obstruction and sleep hypoventilation result in a substantially greater burden of hypoxemia in people with OHS compared to eucapnic obese individuals. Studies have shown that the proportion of sleep time spent less than 90 % (% total sleep time (TST) < 90 %) is strongly associated with the development of awake hypercapnia [37]. In healthy nonobese males sustained hypoxia delays arousal from sleep in response to resistive loading [101]. This mechanism could play a role in worsening daytime respiratory failure in morbidly obese patients with sleep hypoxemia altering the synthesis of various neurotransmitters involved in central respiratory drive and chemoresponsiveness [102, 103].
Nocturnal Positive Airway Pressure Therapy
Nocturnal PAP can be applied to the patient in a number of different ways. The simplest and most common therapy is continuous positive airway pressure (CPAP) where a single level of pressure is applied to the airways. In contrast to CPAP, noninvasive positive pressure ventilation (NPPV) is used to actively increase the patient’s tidal volume, and can be delivered as either pressure preset (most commonly bilevel) or volume preset ventilation. As the name implies, fixed bilevel PAP therapy applies two fixed levels of pressure, with the transition between the inspiratory and expiratory pressures being entirely patient triggered (spontaneous mode), entirely machine driven (timed mode) or, more commonly, a combination of the two (spontaneous/timed or ST mode). In addition, a hybrid mode of bilevel PAP therapy—volume-targeted pressure support—has been developed where a target tidal volume is delivered through variation in the level of inspiratory support. This mode has been proposed to provide the benefits of a consistent level of ventilation arising from the “volume” aspects of the flow delivered with the comfort and leak compensation advantages of pressure ventilation. A purely volume-preset mode of ventilation has also been used to treat OHS, especially in the early years of NPPV prior to the widespread availability of bilevel devices [104]. However, volume preset ventilation has largely been replaced by pressure preset ventilation [15] and is now only used occasionally [29, 92], primarily where volume-targeted pressure support therapy is unavailable.
CPAP Therapy
A number of uncontrolled trials have demonstrated the utility of CPAP in controlling SDB [105], reversing awake respiratory failure [93, 106], and improving quality of life [107] in patients with OHS. Improved ventilatory responses to hypercapnia with nocturnal CPAP have also been shown [62, 74]. To a large extent it is not surprising that a significant number of patients with OHS would respond to CPAP given the high incidence of upper airway obstruction in this population [11, 29, 66] and the relationship between severe OSA and hypercapnia [21, 37, 94]. By preventing apneas and hypopneas, acute accumulation of carbon dioxide would be prevented, helping to normalize not only oxygen saturation but also carbon dioxide. In addition, even low pressure CPAP (7 cmH2O) has been shown to reduce inspiratory pressure swings and neural drive substantially in obese persons [45]. This is possibly due to offsetting the PEEPi that develops in the supine position in these individuals [45]. The volume-inflating effect of CPAP may also help to stabilize the upper airway via caudal traction on the trachea rendering it less collapsible [59], and improve oxygenation by increasing functional residual capacity.
Data from observational and controlled trials suggest that in up to 80 % of patients presenting with stable OHS to sleep laboratories, upper airway obstruction and nocturnal gas exchange are likely to be corrected by CPAP either during the first night of pressure titration [108] or following a period of bilevel therapy [93]. Similarly, in those patients presenting with acute respiratory failure requiring assisted ventilation initially, a significant proportion can be managed adequately with CPAP in the longer term [33, 92].
Several factors appear to distinguish those individuals responding to CPAP and those that “fail” CPAP (non-responders). In a study of “super” obese patients with OHS (BMI > 50 kg m−2), 43 % of subjects failed CPAP (defined in this study as > 20 % of TST spent with SpO2 < 90 %) during the initial night of therapy [105]. Baseline awake blood gases and spirometric measurements could not distinguish CPAP responders from non-responders. However, CPAP non-responders were significantly more obese (BMI mean (SE): 62(2) versus 57(1) kg m−2, p = 0.02) and spent more time with SpO2 < 90 % while asleep during the diagnostic study night compared to those responding to CPAP [105]. In a study looking at patients with OHS initially managed with bilevel PAP therapy, Perez de Llano et al. [92] found that a higher FVC% predicted, less time spent with SpO2 < 90 % and a higher AHI at baseline were characteristics of those able to be switched to longer term CPAP therapy. Taken together, these data suggest that in individuals with OHS in whom upper airway obstruction is the predominant mechanism underlying the development of hypercapnia , CPAP is likely to be effective long-term therapy. In contrast, where mechanical and neurohormonal factors arising from severe obesity are primarily driving the development of hypoventilation , an incomplete response to CPAP therapy is more likely [108]. At present, there are insufficient data regarding threshold values for AHI, BMI, or vital capacity (VC) which can predict an individual’s clinical response to CPAP, and overnight manual titration of PAP therapy remains the standard method of determining pressure needs. This is best carried out in a sleep laboratory, monitoring not only oxygen saturation but also some surrogate of carbon dioxide such as transcutaneous carbon dioxide monitoring to identify the degree to which sleep hypoventilation is corrected.
A major limitation in the evidence regarding the long term use of CPAP is the paucity of data regarding clinical outcomes. Most studies have followed patients managed with CPAP up to 3–6 months with regard to blood gases and quality of life [93, 107]. However, little is known about how these individuals fare compared to those managed with bilevel PAP therapy with regard to longer term adherence to therapy, treatment failure, improvement in cardiometabolic risk factors, or survival. It is also unclear what degree of residual breathing abnormality and hypoxemia can be accepted during the initial CPAP titration night without affecting longer term outcomes, since a progressive improvement in response to CPAP seems to be possible in some [93, 104, 105, 109].
Bilevel PAP Therapy
Most of the literature regarding the management of OHS has focused on the use and outcomes of bilevel PAP therapy rather than CPAP. In many countries, OHS is now the main indication for home NPPV [14, 16]. Bilevel PAP therapy is generally introduced when sustained nocturnal hypoxemia persists despite correction of upper airway obstruction [65, 108], or if the patient is intolerant of CPAP [110]. However, the most effective mode of bilevel PAP therapy for OHS has yet to be determined, and there are very few studies comparing clinical outcomes with different modes of bilevel PAP in the longer term.
Until recently, no randomized trials had compared NPPV to conservative management in terms of its impact on sleep and breathing. Borel and colleagues [111] allocated 37 patients with OHS and mild chronic hypercapnia to either bilevel PAP or lifestyle counseling over a 1-month period. They demonstrated that bilevel PAP (ST mode) was significantly more effective in improving sleep and daytime blood gas parameters than counseling. Although both sleep macro- and microstructure improved significantly under bilevel PAP therapy, subjective daytime sleepiness did not improve to any greater extent than with the conservative management approach.
In a 3-month randomized study comparing CPAP to spontaneous mode fixed bilevel PAP, Piper et al. [93] found no difference between modes with respect to changes in awake PaCO2, adherence to therapy, vigilance, or generic quality of life measures in 36 patients with OHS of mild to moderate severity. However, findings of this study are limited, as patients in whom sustained falls in oxygen saturation below 80 % for more than 10 min during an initial CPAP trial were not enrolled. Nevertheless, those randomized to CPAP therapy still spent 39 % of total sleep time with an oxygen saturation < 90 % at optimal pressures during their first night of CPAP therapy. However, after 3 months of treatment, CPAP was effective in normalizing nocturnal gas exchange in 30 of the 36 subjects. In a retrospective study of OHS patients with concomitant OSA, Mokhlesi et al. [108] reported no difference in the degree to which either of these two modes of PAP improved awake blood gases, suggesting that bilevel PAP therapy is not inherently superior to CPAP in patients with concomitant OSA so long as the PAP therapy used effectively controls SDB.
The majority of trials evaluating NPPV in OHS have compared ST mode bilevel PAP therapy with or without the addition of volume-targeted pressure support [112–115]. Although volume-targeted pressure support may increase ventilation and reduce carbon dioxide during sleep to a greater extent than standard fixed bilevel pressure support [112, 113, 115], clinically important differences between the two modes have yet to be shown. In a comprehensive study of 50 OHS patients (mean BMI 50 ± 7 kg m−2; PaCO2 49 ± 6 mmHg) randomized to volume-targeted pressure support or fixed pressure bilevel ST support over a 3-month period, no difference between modes was seen in terms of arterial blood gas improvement, weight loss, daytime sleepiness, quality of life or physical activity levels [114].
For patients who present in acute hypercapnic respiratory failure , or those without significant upper airway obstruction during sleep, bilevel support is generally used [33, 109, 116, 117]. Even in these groups, a switch to CPAP therapy may be possible longer term [92], with one study finding a significant proportion of patients presenting initially with purely sleep hypoventilation went on to develop OSA when taken off NPPV once hypercapnia was corrected [109].
CPAP Titration
A common approach for determining CPAP in patients with OHS and OSA is to perform an in-laboratory overnight titration study, increasing pressure to prevent obstructive apneic and hypopneic events [65, 75, 118]. If prolonged periods of flow limitation with persisting hypoxemia occur [65], pressure is further increased until the inspiratory flow pattern on the flow trace is normalized [105, 119], and SpO2 is maintained > 90 % [65, 120]. Since persisting hypoventilation is most likely to occur during REM sleep, particular attention needs to be paid to gas exchange and breathing during this sleep stage. If supplemental oxygen has been added to maintain SpO2 > 90 % in the absence of apneas and hypopneas, persisting hypoventilation may be masked. In these circumstances, transcutaneous carbon dioxide monitoring can be a useful tool in identifying the problem [121, 122] and guiding the choice of alternative interventions.
At present there is no evidence to support the safety or efficacy of using auto-titrating CPAP technology for either determining a fixed pressure to correct SDB or as a long-term therapy option in patients with OHS [123]. The algorithms used by auto-titrating CPAP devices have not been adequately evaluated in terms of the persistence of hypopneas that are not pressure responsive (i.e., hypopneas arising from reduced effort and hence decreased tidal volume rather than flow limitation and upper airway instability). In the unattended setting, oximetry is infrequently used during auto-titration studies. Consequently, ongoing hypoventilation and hypoxemia despite control of obstructive events may go unrecognized [124].
Bilevel PAP Titration
Despite the widespread use of NPPV for OHS [14, 16], there is limited evidence to guide what mode and settings most effectively manage this disorder, although general recommendations for the overnight titration of fixed bilevel PAP therapy have been published [120]. While the goals of NPPV are to prevent upper airway obstruction and reverse hypoventilation, therapy itself may induce abnormal breathing events, producing asynchrony between the patient efforts and support from the ventilator [125]. Inadequate or excessive settings may also induce various problems. Patients may not be aware of these events [126] nor may their presence be obvious during routine daytime clinical review [127]. However, asynchrony may contribute to patient discomfort, poor tolerance of therapy, or an incomplete response to treatment. Poor patient–machine synchrony occurs commonly and the importance of overnight monitoring to identify such problems and to improve the efficacy of nocturnal bilevel PAP is increasingly recognized. Consequently, PSG studies for bilevel titration is highly recommended to optimize settings and compare various modes of PAP therapy between different sleep stages and body positions [112, 120]. A systematic description of the types of respiratory events that may occur during NPPV has been developed with the aim of optimizing ventilatory support during PSG studies [128]. However, the relevance of these events with regard to adherence to therapy, quality of life, and survival has yet to be fully evaluated.
Mode
The spontaneous mode has been recommended as the default setting for bilevel PAP unless the patient is unable to trigger the machine consistently, or has a significant number of central events or a low spontaneous breathing rate [120]. Studies reporting positive outcomes with bilevel PAP in OHS patients have used both spontaneous and ST modes [15, 93, 114]. In order to determine if one mode offers greater benefits over another, Contal and colleagues [126] performed a randomized trial evaluating three different backup respiratory rate (BURR) settings over three consecutive nights in ten patients with OHS previously established on bilevel PAP. The three settings were spontaneous mode (i.e., no BURR), a low BURR (ST mode set at two breaths below the average nocturnal respiratory rate recorded over the previous 2 weeks, approx 11 breaths/min), and a high BURR (ST mode set at the nocturnal 95th percentile of respiratory rate of the past 2 weeks, approx 21 breaths/min). Compared to both ST mode settings, the AHI and the oxygen desaturation index were significantly higher in the spontaneous mode, with the majority of events being central or mixed in nature. There was little objective difference in sleep architecture or microarousal index between the three modes apart from a longer sleep latency in the spontaneous mode. Subjectively, patients noticed little difference in sleep quality and comfort between the spontaneous and ST modes. Based on these findings, the authors questioned the wisdom of using a spontaneous mode in this population. However, the protocol used in this study may have influenced the findings. The levels of IPAP and EPAP used across all three nights were not altered from the usual home settings which had previously been determined using only oximetry and transcutaneous carbon dioxide monitoring. The high rate of obstructive events persisting during the spontaneous and low BURR nights suggests the level of EPAP was insufficient to stabilize the upper airway. Repeated arousals from sleep due to upper airway obstruction could have contributed to breathing instability and central events [129]. Mean tidal volume and its variability were also higher during the spontaneous mode compared to the other modes. Although not statistically different, the mean overnight transcutaneous carbon dioxide was 3 mmHg lower during the spontaneous mode night, and this small reduction in carbon dioxide could have favored the development of central events. It is of interest however that in a post hoc analysis, Murphy and colleagues [114] found that patients who had a lower triggering rate (i.e., more machine controlled breaths) during NPPV experienced greater improvements in nocturnal and awake gas exchange and health-related quality of life (HRQoL). These findings provide some supporting evidence that using a mode with a BURR sufficient to achieve a more controlled mode of ventilation may be more effective in this population, and should be further evaluated [130].
Pressure Settings
Comprehensive guidelines for setting IPAP and EPAP levels and adjusting pressure support during in-laboratory titrations have been published [120]. While the general recommendation is to aim for tidal volumes based on 6–8 ml/kg ideal weight, values of 8–10 ml/kg may be more effective in OHS [12, 114]. Although it has been suggested that IPAP above 20 cmH2O may be poorly tolerated [92], other studies have used pressures higher than this without affecting adherence [12, 114]. The EPAP level required to maintain upper airway patency can vary considerably between individuals, with some studies using a fixed pressure of 4–5 cmH2O for all patients [12, 117, 131] while others have used up to 14–16 cmH2O [29, 65, 114]. Appropriate nocturnal monitoring to adjust settings adequately, particularly EPAP, is important to ensure normalization of breathing pattern and gas exchange during sleep. However, significant residual upper airway obstruction or oxygen desaturation persists for many patients despite bilevel PAP therapy [114, 125, 132] (Fig. 4.3a and b). In many of the reported studies, NPPV settings have been determined by nocturnal oximetry and awake blood gases [15, 92, 131]. However, it may not be easy to judge the level of EPAP required to stabilize the upper airway in all sleep stages and body positions based on these two parameters alone. Importantly, sleep fragmentation and impaired sleep quality can occur in the absence of changes in SpO2 or nocturnal carbon dioxide [125], but could nevertheless affect clinical outcomes or acceptance of therapy. These findings support the use of nocturnal polygraphy or PSG to titrate IPAP, EPAP, and BURR settings as well as adjusting other features of the bilevel device to optimize patient–ventilator interactions [125].


Fig. 4.3
a and b: The first panel shows the presence of upper airway obstruction during a bilevel titration during NREM sleep with the machine set in spontaneous mode. Note the paradoxical chest wall movement and the absence of airflow. The second panel demonstrates upper airway obstruction during ST mode of bilevel support. Continued efforts are present but with significant truncation of the airflow signal. These events are causing repetitive desaturation
Oxygen Therapy
Baseline awake hypoxemia and the requirement for supplemental oxygen have both been found to be independent predictors of mortality in long-term retrospective studies in OHS populations [12, 29]. This has largely been attributed to the presence of pulmonary arterial hypertension (PAH), a common sequela of chronic hypoxemia [12]. Therefore, when NPPV alone is inadequate to maintain daytime and/or nocturnal SpO2 greater than 90 %, supplemental oxygen is prescribed. Current recommendations and practices surrounding the prescription and titration of supplemental oxygen in OHS are based on studies in patients with COPD, despite the obvious pathophysiological differences between the two disorders.
Oxygen alone does not correct hypoventilation and may worsen symptoms and clinical complaints [133]. However, it appears to be prescribed for a significant number of patients with OHS as first line therapy [33]. As seen in other groups with hypoventilation, excessively high concentrations of supplemental oxygen producing hyperoxia are potentially harmful to individuals with OHS, and can lead to significant worsening of carbon dioxide levels and respiratory acidosis in some even during wakefulness [134, 135]. Importantly, more hypoxemic patients are more likely to experience larger rises in carbon dioxide with high concentrations of supplemental oxygen [134]. Based on the potential risks surrounding high concentration oxygen in people with OHS, oxygen flow rates should be carefully titrated to maintain SpO2 within a target range (e.g., 88–92 %) [120, 135], whether during NPPV titration, for daytime use or in the acute care setting.
Supplemental oxygen is required in approximately 15–40 % of patients with stable OHS during NPPV initiation [29, 93, 108] and as many as 80 % of those commenced on domiciliary NPPV during an admission to hospital with acute respiratory failure [92]. After the initial phase of 1–3 months, an improvement in nocturnal and daytime gas exchange can be expected [104, 108]. At this time, the need for supplemental oxygen should be reassessed and can often be withdrawn, with only a small proportion (less than 10 %) of individuals with OHS requiring oxygen on a long-term basis [93, 108, 136].
Prior to adding nocturnal supplemental oxygen, NPPV settings should be optimally titrated to otherwise correct SDB and hypoventilation [120]. Residual hypoxemia despite NPPV may arise from the therapy itself related to large leaks or intolerance of high IPAP. Atelectasis or underlying parenchymal lung disease may also contribute to the problem [29]. Clinical guidelines recommend that oxygen be added into the NPPV circuit (via a T-connector at the device outlet) when awake supine SpO2 is less than 88 % while breathing room air in the supine position, or after NPPV settings have been optimally titrated but SpO2 remains less than 90 % for more than 5 min [120]. Oxygen flow rate should be commenced at 1 L/m and increased in 1 L/min increments every 5 min until a stable SpO2 greater than 90 % is achieved [120]. Although these recommendations are not specific to patients with OHS, similar oxygen titration protocols have been successfully implemented in this group [12, 29, 46, 93, 108].
Acute Respiratory Failure and OHS
A significant proportion of patients with OHS present with acute hypercapnic respiratory failure [29, 33, 92, 137, 138], and are successfully managed with NPPV. However, in contrast to patients with acute exacerbations of COPD [139], the same level of evidence for the efficacy of NPPV in OHS patients presenting with acute respiratory acidosis is lacking due to a paucity of controlled trials [116]. Nevertheless, clinical response appears to be as good as or better than that reported for COPD. In a prospective study of 716 consecutive patients receiving NPPV for severe acute hypercapnic respiratory failure caused by either COPD or OHS, Carillo et al. [33] found NPPV to be equally effective in both groups (failure rate 11 % in COPD versus 6 % OHS, p = 0.11) despite a similar degree of acidosis (pH 7.22 ± 0.08 both groups). Furthermore, rates for late NPPV failure, readmission to ICU, and hospital mortality were all lower in patients with OHS than for those with COPD. Over the follow-up period, hospital readmission rates were not significantly different, being around 50 % in both groups, while unadjusted survival was better for those with OHS. However, the 12-month outcome in the OHS group was poorer than that previously reported, and may have been a consequence of the older age of this group and greater severity of their respiratory acidosis compared to previous reports [29, 92]. It is also of note that at discharge just half of the OHS patients were prescribed home PAP therapy, and while reasons for this were not given, it may have influenced readmission and survival rates compared to previous observational studies of NPPV users .
At the extreme end of the spectrum of severity in OHS are those presenting with acute severe hypercapnic respiratory failure and multisystem dysfunction requiring ICU management. This has been labeled the malignant obesity hypoventilation syndrome (MOHS) , and carries with it significant management and prognostic implications [34]. In a retrospective chart review on a single ICU over an 8-month period, Marik and Desai [34] identified 61 patients (8 % of all admissions) with OHS and multiorgan dysfunction. Of note, all had been admitted to the Emergency Department or as an inpatient at least once in the preceding 24 months, with an average of 6 admissions per patient (range 1–39). During each admission, PaCO2 was above 45mmHg. Forty-six patients (75 %) had been diagnosed and treated for COPD or asthma, and during the index hospitalization all had been admitted with a diagnosis of COPD exacerbation and/or heart failure. Twenty four of these individuals had previously been prescribed CPAP or bilevel PAP therapy for SDB, but only three had been diagnosed with OHS. Bilevel PAP therapy was used in 58 patients (three already had tracheostomies), with 23 failing and requiring intubation. Eleven patients (18 %) died. The presentation and outcomes in this subgroup of patients with OHS highlights a very important issue. Individuals with OHS need to be identified early in their disease course to minimize the development of advanced multisystem dysfunction. The high proportion of patients still presenting acutely [29, 33] and the severity of their comorbidities by the time intervention occurs highlights the need for better early screening of morbidly obese individuals to reduce the burden of disease and functional disability [26, 27, 140].
Outcomes of PAP Therapy in OHS
The most immediate and obvious improvements arising from the use of PAP therapy relate to improvements in daytime gas exchange [29, 92, 93, 108, 111, 114] and amelioration of symptoms, especially daytime sleepiness [66, 92, 93, 135]. In uncontrolled studies, hospitalization rates in the 2–3 years following the initiation of NPPV are significantly reduced [15, 26]. Although not well described, sleep architecture is improved with both CPAP and bilevel PAP therapy, with increased amounts of REM and slow wave sleep and reduced stage 1 sleep reported [66, 74, 93, 105, 111].
The use of PAP therapy is associated with improved HRQoL, although whether significant differences between modes of therapy are present is unclear. In a prospective study of Japanese patients with either OSA or mild-to-moderate OHS, both groups reported significantly impaired HRQoL (measured by the Medical Outcome Survey Short Form questionnaire (SF-36)) compared to age matched controls [107]. Following 3–6 months of CPAP, all domains apart from bodily pain showed significant improvements. In a randomized trial comparing CPAP and bilevel PAP therapy in the spontaneous mode over a 3-month period, Piper et al. [93] reported significant within group improvements in the domains of physical functioning, role physical, vitality, and social functioning of the SF-36 in the bilevel PAP group. Only vitality improved significantly in the CPAP group. However, no between treatment differences were observed. The SF-36 is a generic instrument and may not be sensitive enough to identify subtle change in quality of life. Studies comparing bilevel PAP ST therapy to volume-targeted pressure support using a disease-specific instrument (the Severe Respiratory Insufficiency (SRI) questionnaire) have reported significant improvements in HRQoL with NPPV compared to baseline, but not between treatment differences [114, 132], even when better control of nocturnal carbon dioxide with volume-targeted pressure support was achieved [132] .
Reports of the impact of fixed bilevel PAP on lung function in OHS have been inconsistent. One study found increases in TLC of 7.5 % after as little as 4 weeks of NPPV [111] while two other studies found significant improvements in VC and TLC [12, 50] as well as ERV [50] which appeared to plateau at 12 months [50]. In contrast, a number of controlled and observational studies have failed to find any significant change in lung volumes during follow-up periods of 3–50 months [12, 92, 114, 136]. Longer term follow-up of lung function in patients with OHS managed with CPAP has not been documented.
With both CPAP [62, 74] and bilevel PAP therapy [66, 117, 131] improvements in HCVR have been reported, with changes observable within 2 weeks in some studies [62, 66, 74]. However, normalization of HCVR may not be achieved in all patients [66, 117]. One study found substantial individual difference in both baseline HCVR as well as changes following therapy [66]. In another study no significant change in HCVR was seen following 4 weeks of effective NPPV despite a mean fall in awake PaCO2 of 5 mmHg and in bicarbonate of 2 mmol/L [111]. However, these patients were at the mild end of the severity spectrum in OHS, and baseline HCVR was in the low range of normal values.
Pulmonary hypertension is more common and more severe in patients with OHS than those with OSA or overlap syndrome (the coexistence of COPD and OSA) [11]. The likelihood of developing cor pulmonale is also high [26]. In a small, prospective observational study, bilevel PAP therapy used over a 6-month period significantly reduced pulmonary artery systolic pressure and increased 6-min walk distance in patients with OHS with right ventricular overload [141]. CPAP has been shown to reduce pulmonary artery pressure and hypoxic pulmonary vascular reactivity in patients with eucapnic OSA [142]. However, in patients with OHS mild to moderate pulmonary hypertension persists in a substantial proportion of individuals despite bilevel PAP and is inversely related to therapy use [143]. The presence of pulmonary hypertension despite PAP is associated with greater impairments in HRQoL, physical functioning and daytime sleepiness compared to OHS individuals without pulmonary hypertension [143], highlighting the importance of early recognition of the disorder and the need to promote strategies to maximize adherence to therapy .
Once treated with NPPV, survival rates of 97 % at 1 year and 70–80 % at 3 years can be expected [12, 29, 137, 138] which are significantly better than those reported for patients remaining untreated [25, 92]. Similar data for patients managed with CPAP alone have not been reported. In one study, a reduction in PaCO2 (> 23 %) and in hemoglobin following the commencement of NPPV was associated with better survival [12]. Nevertheless, at present there is a lack of evidence to show that normalization of gas exchange has a significant effect on cardiovascular risk factors such as blood pressure, arterial stiffness, or endothelial function [111]. The persistence of cardiovascular comorbidities despite NPPV remains a significant mortality risk [137]. These findings reinforce the need to identify and treat OHS prior to the development of significant comorbid conditions [27] and once present, the importance of effectively managing these complications [137, 144] .
Despite the clinical and survival benefits of NPPV , around 20 % of patients will refuse or be non-adherent with therapy longer term [12, 29, 50, 92, 137]. In contrast to CPAP therapy for OSAS where a significant body of literature has looked at barriers to CPAP use [145–147] and the use of different approaches to improve adherence [148–150], few studies have considered these issues in patients with OHS. Being female [29, 92] and the presence of psychiatric illness [92] have been associated with a higher likelihood of refusal of therapy in patients with OHS. On the other hand, initiating treatment in the acute setting or during a period of clinical stability does not appear to influence acceptance or adherence to NPPV [29]. In those patients continuing on therapy long term, adherence is high with an average nightly use of 5–7 h reported [12, 15, 29, 50]. Somewhat similar to CPAP, it appears that around 4–4.5 h per night of NPPV is the minimum nocturnal usage required to achieve significant changes in PaCO2 [108, 114].
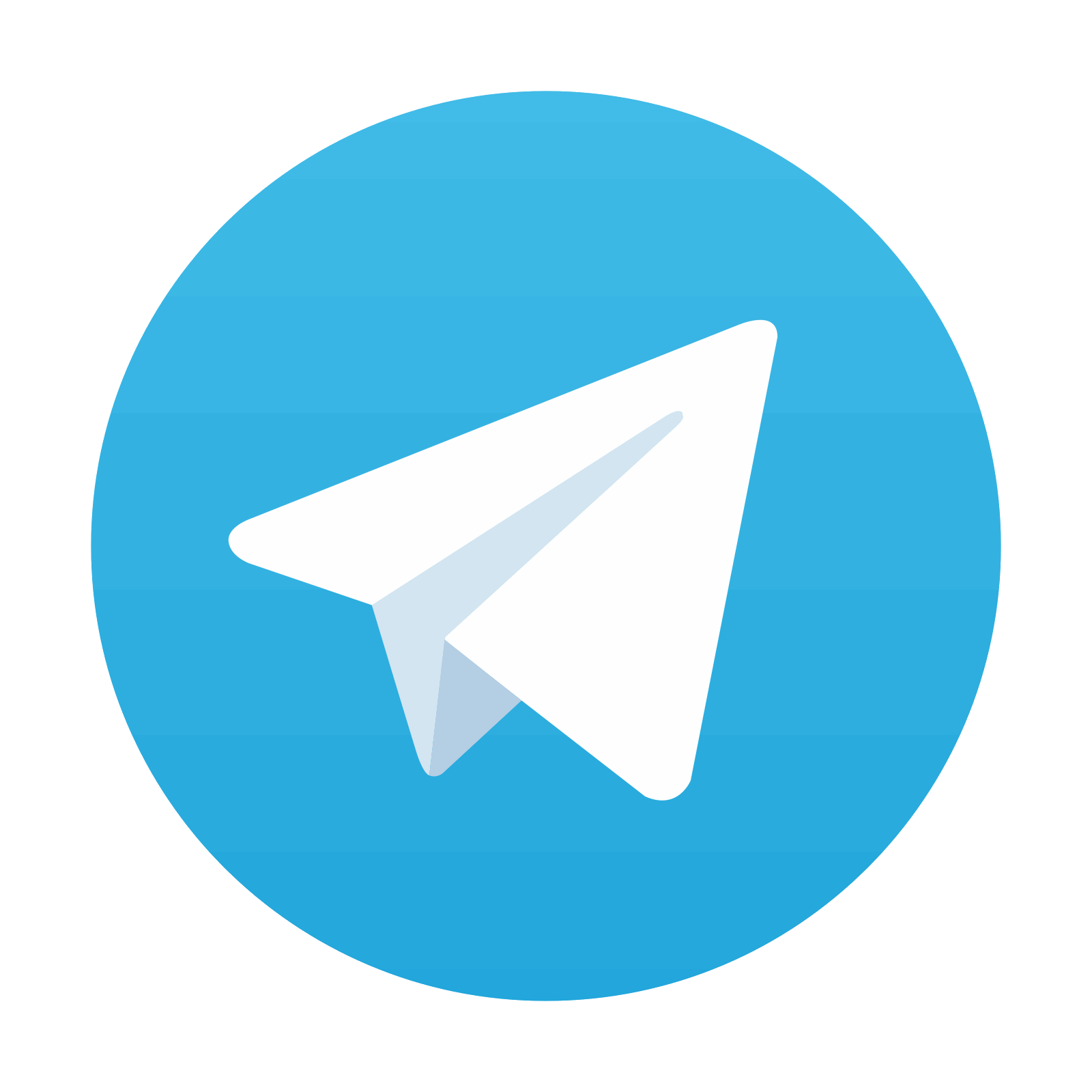
Stay updated, free articles. Join our Telegram channel

Full access? Get Clinical Tree
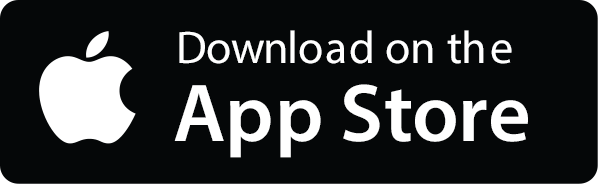
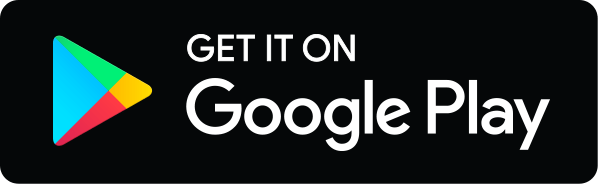