Central Mechanisms
Voluntary-Breathing Controllers
Awake respiration is primarily driven by voluntary-breathing controller mechanisms located in the parietal cerebral cortex. Stimulation of the cortex occurs with routine wake behaviors such as vocalization and physical exertion. Centers within the cortex send signals for inspiration or expiration which are then conducted through corticospinal tracts in the spinal cord to motor neurons [3].
Automatic-Breathing Controllers
Automatic breathing is regulated via a complex system of respiratory generating centers located in the medulla and pons, nerve tracts located in the brainstem, as well as chemical and mechanical feedback mechanisms. During wakefulness, the automatic-breathing system is functional, but respiration is primarily driven by the voluntary-breathing mechanism from the cortex. During both Non-REM and REM sleep, the voluntary-breathing mechanism is absent and respiration is driven entirely by the automatic-breathing mechanism. Destabilization of the automatic breathing centers that can occur in some neurologic diseases has a significant clinical impact on nocturnal (i.e., sleep) respiratory function [4–6].
Peripheral Mechanisms
Lower Motor Neurons
Lower motor neurons are composed of cell bodies located in the anterior horn cell of the spinal cord, and spinal nerve roots, which leave the spinal cord to stimulate the respiratory muscles. At the muscle fiber, the nerve roots interface with the muscle membrane at anatomical junctions known as motor endplates. With nerve firing, acetylcholine is released and subsequently binds to receptors on the muscle side of the motor endplate resulting in depolarization and subsequent muscle contraction [7].
Respiratory Muscles
The respiratory muscles are the mechanical effectors of the respiratory system and consist of four major groups: inspiratory muscles, expiratory muscles, accessory muscles, and muscles of the upper airway. The diaphragm is the major muscle of inspiration, contributing approximately 70 % of inspiratory tidal volume in non-diseased individuals [8]. Innervation of the diaphragm is via the phrenic nerve, which has nerve roots at C3–C5. Stimulation of external intercostal muscles as well as the accessory muscles (sterncleidomastoid, scalene, trapezii, latissimus dorsi, pectoralis major and minor, and platysma) further assists with inspiration via expansion of the rib cage.
Abdominal muscles including rectus abdominus, internal oblique, external oblique, and transverse abdominus are typically thought of as expiratory muscles, but may also play a minor role in inspiration [9]. Internal intercostal muscle stimulation further assists with expiration via contraction of the rib cage.
The muscles of the upper airway (abductors of the vocal cords, palatal elevators, retractors of the tongue, and dilators of the nares) are often included as respiratory muscles and function to maintain airway patency and ensure air exchange.
Feedback Control
Maintenance and fine control of respiration are sustained via feedback networks comprised of chemical and neural receptors in both the central and peripheral nervous system. Neural receptors (muscle spindles, stretch/mechanical/irritant receptors) respond to physical stimulation and are present in the upper airway, respiratory muscles, and vasculature. Chemoreceptors responsive to changes in PaCO2, PaO2, and pH are found both centrally and peripherally. Peripheral chemoreceptors include the carotid and aortic bodies which primarily sense and respond to changes in PaO2, with lesser response to PaCO2 and pH [10]. Central chemoreceptors are located in the brainstem and sense and respond to changes in PaCO2 which is primarily mediated through resultant alterations in cerebrospinal fluid pH [11].
Respiration in Sleep
During sleep, numerous physiologic changes occur (even in normal individuals), which potentially jeopardize respiration. These changes include the absence of voluntary respiration activity, alterations in chemo-responsiveness, and decreased respiratory muscle function with resultant reduction in airway patency and ventilation. Ventilation commonly is most affected in REM sleep, because of the muscle atonia that occurs in that stage of sleep, which spares only ocular and diaphragmatic function. Ventilation in REM sleep is thus largely dependent on diaphragmatic functioning and may demonstrate the earliest manifestations of SRBDs.
Neuromuscular disorders can impact any or all of the aforementioned physiologic mechanisms resulting in significant nocturnal (sleep) respiratory dysfunction. As expected, diaphragmatic dysfunction is a key pathway for SRBDs in this patient population. Diaphragmatic impairment may present in conjunction with generalized muscular weakness due to disease progression, or as an isolated dysfunction attributed to phrenic nerve impairment. Respiratory function may further be impaired in patients with neuromuscular disorders via the subsequent development of restrictive physiology, chronic atelectasis, and aspiration [12].
The aforementioned impairments commonly result in one of, or a combination of SRBDs. Nocturnal hypoventilation is the most common SRBD in this patient population and occurs when ventilation is inadequate to perform needed gas exchange, with resultant ≥ 10 mmHg increase in sleep PaCO2 compared to awake values [13]. Nocturnal hypoventilation should be suspected in patients with documented daytime hypercapnea and hypoxemia. Dysfunction of pharyngeal muscle tone is also commonly seen in neuromuscular patients during sleep, and allows soft tissue in the back of the throat to block the pharyngeal airway with resultant decreased airflow, decreased gas exchange, increased work of breathing, and stimulation of brief arousals to allow resumption of normal breathing. These findings place patients with neuromuscular disease at risk for OSA. Prevalence of OSA in NMD depends on the particular disease with those with bulbar involvement at higher risk. This pattern of repetitive collapse (apnea) and/or partial collapse (hypopnea) can repeat itself hundreds of times throughout the night resulting in significantly fragmented sleep patterns. CSA is characterized by the cyclical or intermittent absence of inspiratory effort in the presence of a patent airway. CSA is less common than hypoventilation and OSA in the neuromuscular patient population, but is often seen with disease associated central neurologic impairment. The overall degree of clinical and physiologic complexity seen in SRBDs emphasizes the importance of thorough sleep evaluation including consideration of polysomnography (PSG).
Clinical Presentation
Impairment of nocturnal breathing in neuromuscular disease patients is often insidious in onset and patients commonly will not be aware of, or volunteer obvious signs/symptoms of, dysfunction. This underscores the importance of routine questioning and clinical assessment. Clinical indicators of impaired nocturnal breathing commonly include fatigue, daytime sleepiness, snoring, and witnessed apnea. Other complaints reported by patients include nocturia, night sweats, heartburn, sexual dysfunction, and morning headaches reflecting the multisystem effects of this disorder . Snoring is a sensitive indicator of OSA, but is a more specific finding when it is very loud and occurring habitually (> 3 days/week). Witnessed apneas and self-report of choking/gasping episodes are also specific indicators [14]. The variable clinical presentation of neuromuscular disorders may confound the identification of examination findings and patient symptoms attributable to the underlying neuromuscular process versus SRBDs.
Routine screening of this patient population for nocturnal as well as daytime symptoms should be standard of practice. This includes interviewing and questioning bed partners and caretakers. Questionnaires for daytime somnolence such as the Epworth Sleepiness Scale (ESS) have shown to be effective screening tools for patients in the general population with OSA [15]. The correlation of such questionnaires with measures of hypoventilation commonly seen in neuromuscular patients is less clear [16]. Efforts to design a questionnaire specific to neuromuscular patients include the five question sleep-disordered breathing in neuromuscular disease questionnaire (SiNQ-5). Values greater than 5 on this questionnaire have been shown to demonstrate abnormal nocturnal respiration with sensitivity of 86 %, specificity of 89 %, positive predictive value of 69 %, and negative predictive value of 96 % [17] (Table 5.1).
Table 5.1
SiNQ-5 questionnaire for neuromuscular disease patients
Do you feel breathless if | |||
---|---|---|---|
You lie down? | Yes(2) | Sometimes (1) | No (0) |
You lean forward? | Yes(2) | Sometimes (1) | No (0) |
You swim in water or lay in a bath? | Yes(2) | Sometimes (1) | No (0) |
Have you changed your position when in bed? | Yes(2) | No (0) | |
Have you noticed a change in your sleep (waking more, getting up, and poor quality sleep)? | Yes(2) | No (0) |
Disease specific patient symptom scales such as the amyotrophic lateral sclerosis functional rating scale revised (ALSFRS-R) questionnaire are commonly recorded in data collection, but there is limited evidence for correlation with SRBDs [18].
Established risk factors for SRBDs in the general population include obesity (BMI > 30), increased neck circumference (> 17 in. in men and > 16 in. in women), middle and older age, postmenopausal women, smoking, craniofacial abnormalities, use of central acting medications, congestive heart failure, and family history of SRBDs [14]. It is likely that these risk factors also make SRBDs more likely in the neuromuscular disease population, although data in this area are lacking.
The variable clinical picture in neuromuscular diseases can make it difficult to identify findings predictive of SRBDs aside from the documented risk factors. Indicators of respiratory muscle weakness while awake such as orthopnea, accessory muscle use, paradoxical breathing, tachypnea, shallow breathing, and cyanosis should alert the provider to likely nocturnal respiratory disturbances and merit further investigation.
Neuromuscular Diseases
Neuromuscular diseases are perhaps most easily classified based on the anatomic area in the nervous system where they create dysfunction, with common groupings including: cerebral cortex, brainstem, basal ganglia, spinal cord, motor neuron, neuromuscular junction , and muscle. Tables 5.2 and 5.3 list various neuromuscular disease processes according to their site of action in either the central or peripheral nervous system. The broad spectrum of disease courses emphasizes the importance of careful consideration of individual patient findings with regard to their underlying disease process (Tables 5.2, 5.3)
Table 5.2
Central nervous system diseases
Cerebral cortex | Brainstem | Basal ganglia | Spinal cord |
---|---|---|---|
Stroke | Multiple sclerosis | Parkison’s disease | Trauma |
Neoplasm | Encephalitis | Chorea | Demyelinating disease |
– | Multiple system atrophy | – | Motor neuron disease |
– | Primary alveolar hypoventilation | – | Pontine myelinolysis |
– | Stroke | – | Poliomyelitis |
– | Neoplasm | – | Stroke |
– | – | Neoplasm |
Table 5.3
Peripheral nervous system diseases
Motor neuron | Neuromuscular junction | Muscle |
---|---|---|
A myotrophic lateral sclerosis | Myasthenia gravis | Muscular dystrophy |
Guillain–Barre syndrome | Lambert–Eaton syndrome | Myotonic dystrophy |
Poliomyelitis | – | Polymyositis |
Spinal muscular atrophy | – | Glycogen-storage disease |
Vasculitides | – | Acid maltase deficiency |
– | – | Nemaline myopathy |
– | – | Mitochondrial myopathy |
Diseases of the Central Nervous System
Voluntary Breathing
Voluntary breathing may be impaired by lesions affecting the descending corticospinal or corticobulbar tracts, and is particularly impaired in association with destructive vascular lesions of the basal pons or the medullary pyramids causing what is known as the “locked in” syndrome [19, 20]. Interruption of these voluntary pathways leads to a regular and unvarying respiratory pattern during wakefulness as well as sleep, with loss of the ability to take a deep breath, hold a breath, or produce a voluntary cough [19]. Respiration is then entirely dependent on automatic breathing, largely based on changes in PaCO2. Lesions impacting voluntary breathing are most commonly secondary to ischemic stroke, but may also be secondary to pontine tumor, pontine myelinolysis, high cervical demyelination, syphilitic arteritis of the medulla, diffuse cortical vascular disease, and head injury [21, 22]. Extrapyramidal disorders such as Parkinsonism have also been associated with impaired voluntary control of breathing as well as SRBDs [23].
Automatic Breathing
“Ondine’s Curse” is the preservation of voluntary respiratory control in the setting of disrupted automatic breathing resulting in central apnea and subsequent failed respiration while asleep. Common associated lesions include bilateral medullary infarction, bulbar poliomyelitis, bilateral cervical tractotomy, and congenital central hypoventilation [21] . Abnormal patterns of respiratory rate and rhythm such as ataxic respiration and Cheyne–Stokes breathing are often a reflection of impairment of the automatic respiratory centers in the brainstem [19]. Ataxic respiration is characterized by an irregular respiratory cycle of variable rate and tidal volume alternating with periods of apnea. It is associated with medullary lesions such as brainstem stroke or compression from rapidly expanding lesions and may indicate impending respiratory arrest. Cheyne–Stokes breathing is classified as a type of CSA and is characterized by a cyclical crescendo–decrescendo waxing and waning of respiratory rate and tidal volume separated by periods of apnea [13, 24]. CSA and in particular Cheyne–Stokes breathing is a common finding post cerebrovascular accident [25]. A prospective study of 161 patients with first-ever transient ischemic attack (TIA) or stroke demonstrated 71 % of patient to have evidence of sleep disordered breathing with 26 % manifesting Cheyne–Stokes breathing. At 3-month follow-up, 62 % of patients demonstrated sleep disordered breathing with 7 % manifesting Cheyne–Stokes breathing. These findings were independent of stroke subtype or event location [26].
Brainstem and Spinal Cord
Spinal cord lesions are most commonly secondary to traumatic injury, but may also include vascular injury, tumor, epidural abscess, transverse myelitis, and syringomyelia [27]. The level of spinal cord injury has a direct impact on the type and severity of ventilatory compromise. The diaphragm is innervated via C3–C5 spinal nerve roots and its loss of function due to C3 and higher lesions typically results in continuous ventilatory requirements. Lesions affecting C3–C5 nerve roots result in varying impact of ventilatory function and will require varying levels of ventilatory support [28]. Lesions below the C5 spinal roots result in preserved diaphragmatic function, but may still significantly impact accessory respiratory muscle function and sympathetic bronchomotor tone resulting in impaired cough and secretion clearance [28, 29].
Poliomyelitis is a disease that affects the anterior horn cell in the spinal cord as well as the motor nerves. Although acute polio is rarely seen in the USA, it is still prevalent in underdeveloped countries [30]. In developed nations, the late effects of polio constitute a collection of findings known as the post-polio syndrome (PPS). PPS is a poorly understood process characterized by muscular weakness, joint pain, and fatigue, which affects as many as half of patients with history of poliomyelitis [31]. Nearly 50 % of patients with PPS report sleep complaints including daytime fatigue and somnolence, headaches, and restless leg symptoms [32]. PSG findings have confirmed increased frequency of nocturnal hypoventilation , CSA, OSA, cortical arousals, and periodic limb movements. Further, kyphoscoliosis is a common complication in PPS patients and is associated with increased frequency of restrictive respiratory dysfunction [33–35].
Diseases of the Peripheral Nervous System
Motor Nerves and Neuromuscular Junction
Disorders of the motor nerves and neuromuscular junction represent a broad spectrum including acute processes such as Guillain–Barré syndrome, botulism, and drug toxicities to more chronic processes such as myasthenia gravis and amyotrophic lateral sclerosis (ALS). Myasthenia gravis is a neuromuscular junction disorder manifest by production of acetylcholine receptor antibodies with resultant generalized muscle weakness and bulbar signs (dysarthria, dysphagia, and diplopia). Respiratory muscle involvement is common in uncontrolled disease states such as myasthenia crises [36]. While there is little debate to the occurrence of SRBDs such as hypoventilation and CSA during periods of disease activity, the occurrence of SRBDs in stable myasthenia patients is much less clear and is limited by quantity of studies as well as small study populations. Early studies suggested increased PSG findings of varying prevalence of CSA, OSA, as well as hypoventilation [37–39]. Later data demonstrated no increased prevalence of CSA and OSA, and no correlation between pulmonary function and SRBDs [40].
ALS is a neurodegenerative disease characterized by the progressive degeneration of motor neurons in the cerebral motor cortex, the corticospinal tracts which conduct impulses to the brainstem, the structures of the brainstem, and the spinal cord [41]. The resultant combination of upper and lower motor neuron dysfunction causes progressive, irreversible weakness affecting respiratory muscles required for airway patency, clearing of secretions, and ventilation. This commonly manifests as a combination of hypoventilation with central and/or OSA. The presence of bulbar dysfunction has not shown to be associated with increased rate of OSA [42–44].
Observational studies have demonstrated > 50 % of ALS patients to report sleep complaints with the most common symptoms being nocturia, sleep onset and maintenance insomnia, nocturnal muscle cramping, morning headaches, and daytime sleepiness [45, 46]. PSG analysis in ALS patients has demonstrated reduced sleep efficiency, increased sleep fragmentation, increased frequency of cortical arousals, increased periodic limb movements, and increased frequency of obstructive and central events as well as hypoventilation [43, 45, 47]. ALS patients with documented diaphragmatic weakness have been shown to be at particular risk for nocturnal hypoventilation and subsequent shorter survival [44, 47]. Bourke and colleagues demonstrated quality of life assessments to be more reliant on respiratory muscle function than PSG data such as the apnea hypopnea index (AHI) [48].
Despite attempts at early recognition and treatment, respiratory failure remains the most common cause of death in the ALS population, with forced vital capacity (FVC) values shown to decline as much as 2.4 % every 30 days [20]. A randomized controlled study by Bourke and colleagues demonstrated that in ALS patients without severe bulbar dysfunction, NPPV improves survival and quality of life. In patients with severe bulbar impairment, NPPV was shown to improve quality of life, but failed to confer a large survival advantage [48]. In summary, the use of NPPV in ALS patients has shown to improve survival, improve quality of life, slow the rate of respiratory muscle decline, and prolong the need for tracheostomy [48–50].
Respiratory Muscles
Neurologic disorders of respiratory muscle function span a broad spectrum of acute and chronic disease processes including myotonic dystrophy, muscular dystrophy, glycogen-storage diseases, and myopathy. Respiratory muscle involvement is common in this disease population with diaphragmatic weakness and SRBDs typically occurring later in the disease course. Acid maltase deficiency (Pompe disease) is an exception to this rule, with early diaphragmatic involvement and nocturnal hypoventilation often preceding limb or other muscle weakness [51].
A prototype of chronic progressive muscular disease affecting respiration is Duchenne muscular dystrophy (DMD), an X-linked disorder characterized by progressive skeletal muscle weakness leading to respiratory dysfunction and failure late in the disease course. A prospective study of 19 DMD patients demonstrated either a PaCO2 ≥ 45 mmHg or a forced expiratory value in 1 s (FEV1) < 40 % of predicted value to be indicative of nocturnal hypoventilation as determined by PSG [52]. PSG studies in patients with DMD have demonstrated increased occurrence of nocturnal hypoventilation , sleep fragmentation, and OSA. Hypoventilation is significantly worsened in REM sleep, likely due to combined respiratory muscle weakness and restrictive lung physiology often exacerbated by kyphoscoliosis [53, 54]. Noninvasive positive pressure ventilation (NPPV) use in DMD patients has shown to reduce daytime PaCO2 values, prolong survival, increase likelihood of tracheostomy decannulation, and decrease daytime ventilation requirements [52, 55, 56].
Assessment of Respiratory Muscle Impairment
Early recognition of respiratory muscle impairment and subsequent risk for SRBDs is a challenging task in the neuromuscular patient population. While PSG is the gold standard for identification of SRBDs, it is costly and often logistically difficult to complete in this patient population. There has been extensive research to identify home- or office-based clinical predictors to be used in place of or adjunctive to PSG. Modalities investigated have included symptom-based questionnaires, serologic laboratory analysis, nocturnal pulse oximetry (NPO), nocturnal carbon dioxide monitoring, as well as pulmonary function testing (PFT). To date, no single modality has proven effective as a solitary tool. Individual consideration of each patient’s clinical picture and combined testing data should drive the decision of when and how to initiate treatment.
The utility of symptom-based patient questionnaires in predicting SRBDs was discussed earlier in this text.
Laboratory Analysis
Daytime arterial blood gas (ABG) analysis for detection of hypercarbia and hypoxemia has been extensively evaluated as a screening tool for SRBDs, and in specific disease populations it has shown to be a good screening tool for nocturnal hypoventilation [52]. In general, daytime PaCO2 values > 45 mmHg are viewed as sensitive measures of nocturnal hypoventilation [52]. However, normal PaCO2 values do not exclude the possibility of nocturnal hypoventilation. In fact, it is established that normal daytime ABG analysis may occur in patients with significant nocturnal hypoxia and hypercarbia. There is subsequently, significant concern that normal daytime ABG analysis may fail to identify patients with significant nocturnal respiratory disturbances. This is of particular consequence given data showing that early initiation of treatment improves quality of life and survival in certain disease populations [48–50].
Nocturnal Pulse Oximetry (NPO):
NPO is a relatively inexpensive and practical test that has been used extensively in neuromuscular patients to screen for SRBDs. Baseline oxygen saturation levels as well as duration and frequency of desaturation events have been utilized as rough estimates of hypoventilation and possibly OSA. While NPO has demonstrated some efficacy in the detection of nocturnal hypoventilation in specific disease populations such as ALS [57], it is possible to fail to identify patients with hypoventilation due to the absence of direct measurement of hypercarbia. Thus, while commonly used, NPO alone has shown to be a limited tool for diagnosis and monitoring of hypoventilation [58].
Nocturnal Carbon Dioxide Monitoring
Use of nocturnal carbon dioxide monitoring via transcutaneous and end-tidal sensors has also been evaluated for the detection of early nocturnal hypoventilation . While not a direct measurement of PaCO2, transcutaneous and end-tidal sensors have been validated in clinic-based settings [59, 60]. Recent technological advancements have increased the availability and quality of transcutaneous monitoring, and retrospective studies of home-based monitoring have demonstrated utility as a screening tool in conjunction with NPO [58, 59].
Pulmonary Function Testing (PFT):
PFT including office-based spirometry and measures of respiratory effort has undergone extensive evaluation as a diagnostic indicator of nocturnal SRBDs. The literature on this topic is vast and often confusing. Patients with neuromuscular disorders frequently demonstrate restrictive physiology with diminished FVC in relation to forced expiratory volume in 1 s. The FEV1/FVC ratio is thus commonly preserved with values approaching 1 or greater. Respiratory muscle weakness is commonly seen in this patient population and is often quantified via maximum inspiratory and expiratory pressures (MIP and MEP) as well as sniff nasal inspiratory pressure (SNIP).
Disease specific guidelines, including ALS practice parameters, have previously recommended use of FVC values to identify respiratory muscle weakness, risk for nocturnal hypoventilation, and subsequent need for treatment. Despite several studies suggesting that FVC values alone are inadequate predictors for respiratory muscle weakness and survival [57, 61–63], various absolute cutoffs for FVC in multiple disease populations have been proposed ranging from 25 to 75 % of predicted values. Both the consensus guidelines from the American College of Physicians (ACP) and the ALS practice parameters from the American Academy of Neurology recommend initiation of NPPV for FVC values < 50 % of predicted [22, 64]. Dynamic assessment of FVC has also been investigated by utilizing differences in measurements of supine and upright FVC values. When diaphragmatic function is normal, the difference between upright and supine FVC values (∆FVC), ranges from 0 to 7.5 % [65]. ∆FVC values in patients with diaphragmatic weakness are significantly larger and may reach differences up to 50 % [66, 67]. As with absolute FVC values, there is no definitive ∆FVC value shown to be an isolated predictor of SRBDs across this patient population.
Assessment of respiratory muscle weakness via evaluation of MIP and MEP has also been thoroughly evaluated. MIP values are traditionally viewed as indicative of inspiratory muscle function predominately driven by the diaphragm , while MEP values are considered reflective of accessory expiratory muscle function. In healthy middle aged adults mean (+/− SD) values of MEP and MIP are 111 +/− 25 cmH2O and 79 +/− 19 cmH2O in females and 192 +/− 42 cmH2O and 117 +/− 25 cmH2O in males, respectively [68]. MIP has been suggested as a more sensitive and earlier indicator of respiratory muscle weakness in ALS patients, with data demonstrating deficiencies in MIP to occur 4–6.5 months earlier than deficiencies in FVC [69].
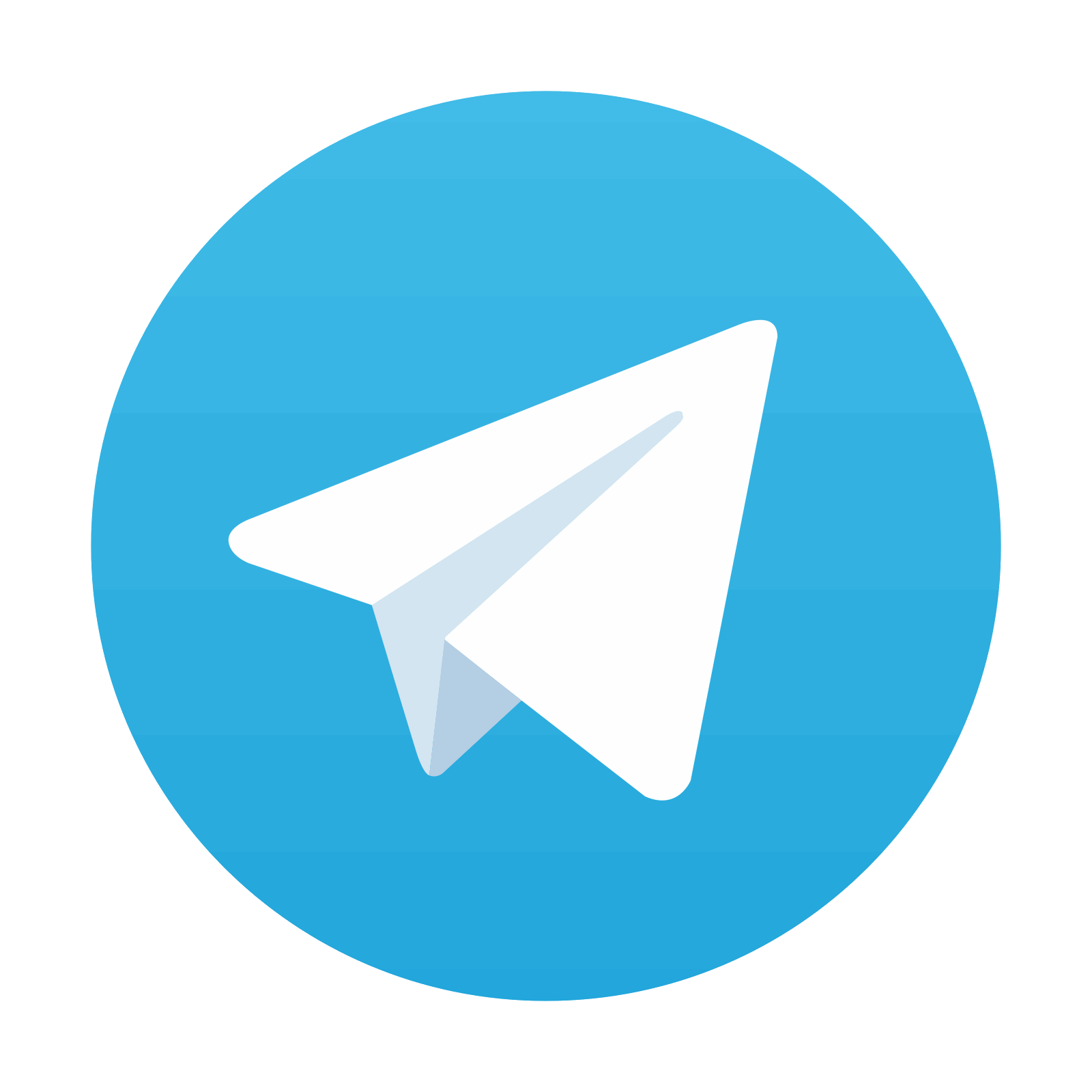
Stay updated, free articles. Join our Telegram channel

Full access? Get Clinical Tree
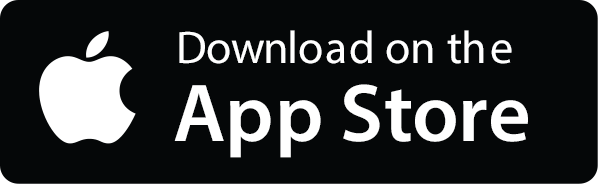
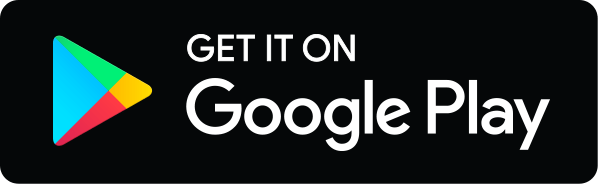