Fig. 35.1
Time course of platelets, fibrinogen, D-dimer % change from baseline between ML change-outs (black vertical lines) during a prolonged VV ECMO treatment, showing “circuit coagulation activation”
A significant technological improvement in MLs has been achieved in recent years, with the transition from silicon non-microporous membrane MLs to microporous hollow-fiber MLs. The latter allows highly efficient gas exchange, reduction in transmembrane pressures, and low priming volume, but plasma leakage through microporous fibers frequently occurs, reducing gas transfer rate. More recently polymethylpentene hollow-fiber MLs have eliminated this problem [4], allowing more longer treatments, reducing the need for circuit change-out. Anyway, even with polymethylpentene MLs, progressive clot formation with partial to total occlusion of blood path and loss of gas-exchanging surface is still an unresolved problem (Fig. 35.2) [2, 6].
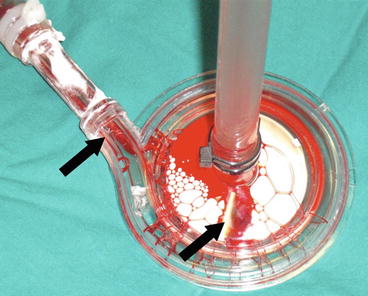
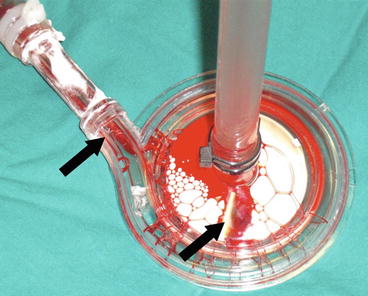
Fig. 35.2
Black arrows showing clots in the pump head after a circuit change-out
35.2.2 Circuit/Pump Failure
Though more resistant materials and more biologically compatible inner circuit coatings have been developed, hemocompatibility is still limited, and careful monitoring of the ECMO system is required. Each part of the circuit must be inspected frequently in search of blood clots and deformities of tubing, especially where blood flow is more turbulent as at fittings, stopcocks, and cannulae. Expected complications may be different depending on the pump technology employed and on patient population (adult vs. pediatric); the most popular technologies are the roller pump, actually more diffused for children [5], and the centrifugal pump. The overall complication rate is not significantly different [8], with a significative difference in hemolysis (but not in in-hospital survival) in favor of roller pumps among children [5]. Roller pump specific complications are raceway or circuit break and, consequently, air embolism; centrifugal pumps instead are specifically exposed to pump head/pump engine decoupling, consequently stopping treatment and thrombosis. Alternative technologies such as nonocclusive and miniaturized pumps have been developed [8]. Some centres use two pumps/MLs running in parallel: in case of failure of an ECMO system, the second ECMO allows a full support to the patient. Other centers have a primed ML circuit always ready to be implemented [8]. Ideally whole circuit or ML change-out should be performed electively to decrease morbidity due to circulatory lack of assistance (VA ECMO) or oxygenation/CO2 removal (VV ECMO) during change-out procedure. Up to now circuits are changed according to clinical nonstandardized criteria. Major clinical criteria may be summarized in:
1.
Circuit coagulation activation
2.
Gross thrombosis of circuit components
3.
Technical failure of the circuit/ML
35.3 Monitoring Techniques
35.3.1 Blood Flow and RPM
In order to monitor energy/mechanical failure of the ECMO pump, rotation per minute (RPM, available both for roller and centrifugal pumps) is always continuously displayed and alarmed. Monitoring RPM is of particular importance on roller pumps, RPM being directly related to blood flow (when occlusion is accurately set and circuit and raceway are intact); when a centrifugal pump is employed, for a set RPM, blood flow is mainly driven by flow resistance of the ECMO circuit (cannulae, tubing, ML) and by patient fluid status: therefore, in case of low-volume state and high RPM, blood flow may abruptly fall, eventually leading to suctioning of venous wall and/or ECMO treatment interruption. This occurrence often leads to blood–pump head decoupling and requires a special emergency procedure to restart ECMO. Close flow monitoring and alarming are thus needed. According to different technologies, companies developed specially modified fittings where flowmeters may be applied on the circuit.
35.3.2 Anticoagulation
As seen above ECMO circuits still require adjunctive anticoagulation to avoid circuit component thrombosis. Adequate anticoagulation level during ECMO is the balance between circuit/patient thrombosis and patient bleeding; finding the optimal level could be challenging as it is not absolute and varies according to circuit and patient status [9] (i.e., preexisting coagulation disorders, ECMO circuit and treatment duration, sepsis). As bleeding and thrombosis are still the principal causes of morbidity and mortality during ECMO [7], strict anticoagulation monitoring is essential.
ECMO anticoagulation is generally obtained with unfractionated heparin (UFH), which is the drug of choice because of a favorable pharmacological profile: in fact it has a parenteral, fast-onset, rapidly reversible effect and is an economical and a widely diffused drug. Despite this, heparin is not ideal, as it has a non-predictable anticoagulant effect, thus requiring close monitoring and frequent variations in dosage. Moreover, anticoagulant effect could not be accurately monitored, and consequently each test requires careful interpretation (see below), sometimes making clinical management difficult [7, 10]. Unfractionated heparin acts by binding with antithrombin III (ATIII); hence, as hepatic ATIII production may be impaired, ATIII level must be frequently monitored and kept constant. Finally, it is associated with heparin-induced thrombocytopenia (HIT) [11, 12].
Heparin anticoagulation can be monitored at bedside with activated clotting time (ACT), point-of-care activated partial thromboplastin time (aPTT), and viscoelastic tests (VET) or can be monitored with routine (aPTT, D-dimers) or special (heparin plasma level – antiXa activity) coagulation lab tests.
aPTT is the standard test to measure heparin anticoagulation; it measures plasma coagulation pathway in-vitro at 37°C temperature. Accordingly, interactions between platelets and other blood cells with soluble factors are not tested, so aPTT test reflects only a small fraction of the thrombin produced during the coagulation process [13].
ACT [14] is a widely diffused test among ECMO centers [9], being classically employed in the cardiopulmonary bypass setting, where high dose of heparin and point-of-care test are required. ACT can also be used for lower dose of heparin: a sample of fresh whole blood is inserted into a warmed cuvette where coagulation is activated by a negatively charged activator (celite or kaolin). ACT measures the seconds needed for the blood to start clotting. Anticoagulation is kept to obtain a value between 180 and 220 s. It is affected by sepsis, D-dimers, platelet dysfunction, thrombocytopenia, hypofibrinogenemia, and hypothermia.
Correlation between ACT and aPTT is poor [9, 14]; therefore, in clinical decision making, factors influencing both tests must be considered.
Some centers employ viscoelastic test as an adjunct to anticoagulant therapy monitoring, being a test extremely sensitive of heparin activity variation. Nevertheless, experience in this application of thromboelastography/thromboelastometry is still limited [7].
This is the anticoagulation monitoring protocol that we follow in our centre:
1.
Platelets
(a)
Platelet levels are checked every 12–24 h.
(b)
Platelets are administered when lower than 50,000 units/mm3.
2.
ATIII
(a)
Plasmatic ATIII level checked daily until stable value with (or without) replacement and then every 2–3 days.
(b)
Replace as needed to obtain a value >100 %. Dosage is decided according to the formula: unit to be administered = weight (kg) × [ATIII desired (%) − ATIII measured (%)]/1.4.
3.
ACT
(a)
Checked after 1 and 3 h after heparin boluses and then every 6–8 h. Target result is decided by integration of ACT with other coagulation tests.
4.
Standard coagulation tests
(a)
aPTT, fibrinogen, and D-dimers checked three times a day. aPTT value is kept between 50 and 60 s (ideally aPTT 1.5–2 times the patient’s baseline), and FNG above 200 mg/dL.
(b)
Ionized calcium is obtained with ABG analysis and is kept within physiological range.
5.
Thromboelastography
(a)
Upon clinical decision and simultaneously with standard coagulation tests to address baseline coagulation (whole kaolin + heparinase TEG tracing shape) and R time value in the kaolin tracing.
35.3.3 Pressures
Circuit pressure monitoring may be mounted onto the ECMO circuit at different sites, representing an easy and effective alarming and information system about both roller pump and centrifugal pump ECMO performances. To monitor pressures and to draw blood, manufacturers provide preassembled or spare fittings with a side port (Luer-lock connectors). Monitoring sites vary through institutions according to the age of the ECMO patient [4] and to local practices and protocols. Commonly used monitored sites are:
Get Clinical Tree app for offline access
1.
Two sites on either side of the ML (pre-ML and post-ML), providing information about variation in pressure drop and blood flow resistances through the oxygenator. An increase in pressure drop suggests ML thrombosis (Fig. 35.3) [2]. Resistance of blood flowing through the oxygenator may be computed according to the following formula: R = (pin-pout)/BF, where pressures are expressed in millimeters of mercury and BF in liters per minute (R = mmHg/L/min). Each manufacturer provides product-specific performance charts comparing resistances at different blood flows.
