Molecular Carcinogenesis and Chemoprevention of Lung Cancer
Fadlo Khuri
Nico Van Zandwijk
Lung cancer is by far the leading cause of cancer-related death, worldwide and in the United States.1 Despite substantial progress in the treatment of lung cancer, which correlates with improvements in surgery, radiation oncology, and chemotherapy, 5-year survival has improved from 7% to only 16% over the last 2 decades. Although median survival in all stages of disease has improved somewhat, the low likelihood of obtaining a cure even with optimal modern therapy is largely because of the clinical presentation of this disease in its advanced stages The late diagnosis is almost certainly related to a covert carcinogenesis phase that often evolves over 10 to 20 years and requires multiple molecular genetic changes to facilitate the development of invasive lung cancer.2,3 Thus, there exists a strong rationale for intervening in those stages of lung carcinogenesis that could result in arrest or delay of disease progression to the stage of frank cancer. Therefore, the strategies that are likely to be successful will likely optimally target both better biological understanding of lung cancer-mediated field carcinogenesis, and the development of reliable biomarkers that can accurately assess the likelihood of efficacy and toxicity across target populations. Ultimately, strategies involving screening and early detection, biomarker-based chemoprevention, smoking cessation, and better biological understanding of the phenomenon of lung carcinogenesis are all likely to be coupled with individualized risk stratification in the development of modern approaches to prevent this dreaded disease and reduce the societal burden of lung cancer.
LUNG CANCER EPIDEMIOLOGY
In most cases, lung cancer is strongly associated with direct consumption of tobacco products.4 In fact, 87% of all lung cancers are detected in individuals who are active or former smokers, with an additional 6% to 7% composed of partners of smokers or their offspring (see Chapter 2). The second most common known cause of lung cancer is radon, which is associated with a significant increase in lung cancer risk, and with other risk factors including asbestos exposure. Nonetheless, despite the high degree of certainty that currently exists over the link between tobacco smoke and lung cancer, the understanding of these facts took several decades to clarify.
LUNG FIELD CARCINOGENESIS
The original description of field carcinogenesis, or field cancerization as it was originally called, dates back to Slaughter and coworkers,5 who showed that multiple foci of epithelial hyperplasia, hyperkeratinization, atypia, dysplasia, and carcinoma in situ occurred in otherwise normal-appearing epithelium adjacent to cancers of the oral pharynx. This empiric evidence strongly suggested that carcinogen exposure has widespread effects throughout the entire carcinogen-damaged epithelial field. These diffuse histological changes suggested that the development of malignancy in the proximal upper aerodigestive tract is a result of the dose of carcinogen impacting exposed cell populations spread throughout this area. Auerbach and colleagues6 observed and demonstrated the same pattern of heterogeneous, multifocal histological changes in the bronchial epithelium of smokers, many of whom have lung cancer. Thus, the concept of field cancerization or carcinogenesis was coined to describe the diffuse damage done by chronic exposure to carcinogens. Field carcinogenesis also forms the basis for the observation that individuals who survive a first cancer are often likely to develop a second malignancy in the region of the condemned epithelium.7,8,9
As these findings have been validated over the last several decades, increasing evidence has come to bear explaining the molecular genetic abnormalities that lead ultimately to the stepwise development of clonal invasion-competent cancer of the airway. Among the earliest findings in the aerodigestive tract of chronic smokers are loss of heterozygosity in chromosomes 3p, 9p, and 17p2,10,11,12,13,14 (see Chapter 6). Clonal genetic abnormalities seen in normal histologic regions of the airway and not necessarily in areas that had detectable changes were
further evidence that damage was scattered throughout the entire field (see Chapter 19). It also brought to bear the limitations of modern histologic techniques in detecting the extent of the damage induced by tobacco smoke in the airway. The importance of these losses of hererozygosity are crystallized in the fact that, for example, the short arm of chromosome 3, which is often the earliest loss and is demonstrated on occasion even in early hyperplasia,10 results in the loss of a region rich in tumor suppressor genes. There is also evidence for losses of the short arms of chromosomes 9 and 17, resulting in loss of tumor suppressor genes in p16 and p53, both of which are important to the cell’s ability to repair DNA damage done by the tobacco carcinogen. p53 in particular, acts as a transcription factor in the control of G1 arrest and apoptosis, or programmed cell death, thereby allowing the cell to repair existing DNA damage or make the determinant decision to push a cell into the apoptotic process once it is too far damaged for repair. p16, found on chromosome 9p, negatively controls cyclin-dependent kinases (CDK)-cyclin activity by overexpression of cyclin-D1. By inhibiting cyclin-D1/CDK, the damaged cell is also prevented from entering into mitosis and proliferating with damaged DNA (see also Chapter 14).
further evidence that damage was scattered throughout the entire field (see Chapter 19). It also brought to bear the limitations of modern histologic techniques in detecting the extent of the damage induced by tobacco smoke in the airway. The importance of these losses of hererozygosity are crystallized in the fact that, for example, the short arm of chromosome 3, which is often the earliest loss and is demonstrated on occasion even in early hyperplasia,10 results in the loss of a region rich in tumor suppressor genes. There is also evidence for losses of the short arms of chromosomes 9 and 17, resulting in loss of tumor suppressor genes in p16 and p53, both of which are important to the cell’s ability to repair DNA damage done by the tobacco carcinogen. p53 in particular, acts as a transcription factor in the control of G1 arrest and apoptosis, or programmed cell death, thereby allowing the cell to repair existing DNA damage or make the determinant decision to push a cell into the apoptotic process once it is too far damaged for repair. p16, found on chromosome 9p, negatively controls cyclin-dependent kinases (CDK)-cyclin activity by overexpression of cyclin-D1. By inhibiting cyclin-D1/CDK, the damaged cell is also prevented from entering into mitosis and proliferating with damaged DNA (see also Chapter 14).
Increasing evidence shows that the loss of these and other tumor suppressor genes in lung cancer is augmented by the activation of several critical proto-oncogenes. For example, RAS, c-MYC, and the epidermal growth factor receptors (EGFRs), are all tumor-promoting genes that are activated progressively during lung carcinogenesis. K-ras mutations are particularly common in adenocarcinomas of the lung, with some studies showing 30% of lung adenocarcinomas in smokers containing K-ras mutations.15 The EGFR is frequently amplified in non-small cell lung cancers (NSCLCs) and in fact in lung carcinogenesis16 (see Chapter 49). Mutations have been detected in the EGFR tyrosine kinase binding domain conferring sensitivity to small molecule EGFR-tyrosine kinase inhibitors (EGFR-TKIs).17,18 Interestingly, both K-ras mutations and EGFR mutations have been found in the airways of smokers and nonsmokers in regions distant from the primary tumor, suggesting that both play important roles in field carcinogenesis. Thus, modern molecular tools can help us better understand the process of field carcinogenesis and, as we will discuss, implement the development of targeted agents that can arrest or inhibit this otherwise inexorable progression.
Epigenetic Abnormalities in the Airway Loss of function of tumor suppressor genes and greater function of oncogenes through mutations or gene activations are not the only mechanisms by which carcinogenesis is augmented in lung cancer patients. A growing body of evidence suggests that gene silencing through epigenetic means can be crucial in lung carcinogenesis (see Chapter 7). For example, in NSCLC, some tumor suppressor genes such as RASSF1A, also located in the tumor suppressor gene-rich chromosome 3p, encodes a protein that heterodimerizes with Nore-1, an important RAS effector with pro-apoptotic effect.19 Evidence from several groups suggests that in NSCLC, RASSF1A can be frequently inactivated by hypermethylation.20,21 Another important gene that can be inactivated by epigenetic means is the retinoic acid receptor-β (RAR-β), which also maps to chromosome 3p. RAR-β is a nuclear retinoid receptor with vitamin A-dependent transcriptional activity.22 RAR-β is a gene that is shown to be gradually lost in lung carcinogenesis,23 and this can be regulated either by loss or by hypermethylation.24,25 Interestingly, the maintenance of RAR-β in mature tumors is a factor for poor prognosis26 and it is differentially regulated in current versus former smokers. For example, a recent retrospective study on methylation status and occurrence of second primary tumors (SPTs) in completely resected NSCLC patients revealed a strong association between RAR-β2 hypermethylation in the development of SPTs only in former smokers.27 In current smokers, hypermethylation was associated with a protective effect, pointing to the critical value of context with regard to smoking status in understanding the biological effects of retinoid receptors in lung cancer.27,28
Lung Cancer Susceptibility and Risk Stratification Although there is little doubt that exposure to tobacco smoke is the major risk factor for the development of lung cancer, there is also progressive evidence that the risk of developing lung cancer, even among the heaviest smokers, varies widely according to genetic and perhaps even dietary factors. This is reinforced by the fact that 85% of heavy smokers will not develop lung cancer, implicating important differences in lung cancer susceptibility across the population in the likelihood of developing overt disease. However, there are significant competing risks for death of heavy smokers, including cardiovascular disease, chronic obstructive pulmonary disease, and other malignancies including those of the upper airway, bladder, cervix, kidney, and pancreas. Evidence exists, however, to show that every other smoker dies of a smoking-related cause.29 Despite this, accumulating evidence suggests that genetic and epigenetic factors are critical in modulating individual susceptibility to lung cancer or other consequences of tobacco exposure.30 The various carcinogens from cigarette smoke, benzpyrine and 4-(methylnitrosoamino)-1-(3-pyridil)-1 butanone (NNK) require metabolic activation before they can exert their full carcinogenic effects. Various activation pathways compete with detoxification pathways and the balance between the two is critical in modulating cancer risk. Cytochrome P450s serve as carcinogen-metabolizing enzymes, whereas glutathione transferases serve as detoxification enzymes. Both sets of these important genes are known to have significant polymorphisms correlating with variations in lung cancer risk (see Chapter 4).
Other important modulators of risk in lung cancer include diet and gender. Dietary factors play an important role as epigenetic modulators of lung cancer susceptibility. In several case-controlled studies, defective detoxification and defective repair of genetic damage have been shown to be associated with increased individual susceptibility to lung cancer.31,32,33,34 However, certain food constituents appear to afford a significant degree of protection to individuals with limitations to their detoxification capacity.35 This relationship between diet and lung cancer has been extensively explored and used as the basis
for the development of novel approaches to the prevention of lung cancer. For example, large studies suggest that diets that have a high intake of fruits and vegetables are associated with a reduced risk of lung cancer.36 Further evidence suggests that a Mediterranean diet is associated with a substantial reduction in several cancers, including lung cancer.37
for the development of novel approaches to the prevention of lung cancer. For example, large studies suggest that diets that have a high intake of fruits and vegetables are associated with a reduced risk of lung cancer.36 Further evidence suggests that a Mediterranean diet is associated with a substantial reduction in several cancers, including lung cancer.37
TABLE 21.1 Levels of Prevention in Lung and Aerodigestive Cancer | ||||||||||||
---|---|---|---|---|---|---|---|---|---|---|---|---|
|
Basis of Clinical Strategies in Lung Cancer Prevention As several dietary adjustments and micronutrients have been associated with lower risks of lung cancer, including vitamin E, selenium, isothiocyanates, and BMT-polyphenols, several large randomized trials have sought to delineate which compounds in complex human diets are most responsible for the protective effects seen.36,38 Initially, the greatest degree of evidence existed around the potency of carotenoids and retinoids and the broad epidemiologic indications implicating them in reduction of cancer risk in general, and lung cancer risk in particular.39,40 In fact, the original definition of chemoprevention coined by Michael Sporn as “attempts to reverse, suppress, and prevent carcinogenic progression to overt cancer”41 is based on his and other’s experimental work showing that vitamin A analogues are capable of reversing or preventing epithelial carcinogenesis in mouse models of cancer.
Consequently, the identification of populations at risk for development of this disease followed suit. For example, target populations in many of the early prevention studies included broad populations of individuals at risk because of their exposure to tobacco and/or asbestos. Others sought to develop strategies for uranium miners, also a population believed to be at enhanced risk for developing lung cancer. Thus, with several decades of broad epidemiologic and dietary investigation, augmented by important experimental systems showing several classes of compounds, especially the retinoids and carotenoids, were capable of reversing cell damage, a strong drive to develop this approach in human populations was born. The trials focused on broad patient populations, including those of individuals at even higher risk, in other words, individuals with known premalignancy of the airway, or those patients who had already developed a primary tobacco-related cancer, and thus were at the greatest risk for developing a second primary tumor.
Clinical Chemoprevention Trials Laudable as smoking cessation efforts have been, it is clear that lung cancers are developing in former smokers.42,43 Thus, the design of lung cancer trials has taken the approach to develop strategies based on the different populations considered for each intervention. Risk categories were different across these populations, and thus the categories of chemoprevention were defined as primary, secondary, and tertiary prevention (Table 21.1). Primary prevention involved intervening in patient populations at increased risk but with no histological or clinical evidence of cancer. The end point here was reduction in incidence of lung cancer, and reduction in death from lung cancer. Secondary prevention involved attempts in individuals with evidence of lung premalignancy to prevent progression of that premalignancy or, ideally, to reverse it to an earlier stage of carcinogenesis. Finally, studies in the highest risk population were undertaken in those individuals who had already developed tobacco-related malignancy. Here, the goal was to prevent the development of SPTs. As expected, many of the early trials focused on using retinoids and carotenoids as the chemoprevention agents of choice, given that the weight of epidemiological and experimental evidence for these compounds was significantly greater than that for other classes of compounds.
Primary Chemoprevention Studies Several large randomized studies were undertaken in populations deemed to be at increased risk of lung cancer caused by exposure to tobacco, asbestos, or their occupation as uranium miners. Three major studies, the α-tocopherol/β-carotene (ATBC), β-carotene and retinol efficacy trial (CARET), and the U.S. Physician Study, were carried out using increasingly high doses of the carotenoid, β-carotene (Table 21.2). None of the three studies showed any reduction of lung cancer risk utilizing these compounds, and two of these studies, the ATBC and CARET studies, had surprising findings, both showing significant increases in lung cancer risk associated with supplementation with β-carotene. Both of these studies, which were 2 plus 2 factorial studies, also involved a second agent as an intervention. In these cases neither the second agent nor vitamin A itself was found to be associated with an increased risk of lung cancer, but neither were they protective against lung cancer incidence. Interestingly, both of these studies showed that the risk was increased only in populations
that were heavy smokers, and particularly so in those individuals who had evidence of pulmonary asbestosis, as measured by manifestation of pleural plaques on chest radiograph.
that were heavy smokers, and particularly so in those individuals who had evidence of pulmonary asbestosis, as measured by manifestation of pleural plaques on chest radiograph.
TABLE 21.2 Primary Randomized Chemoprevention Trials in Lung | ||||||||||||||||||||
---|---|---|---|---|---|---|---|---|---|---|---|---|---|---|---|---|---|---|---|---|
|
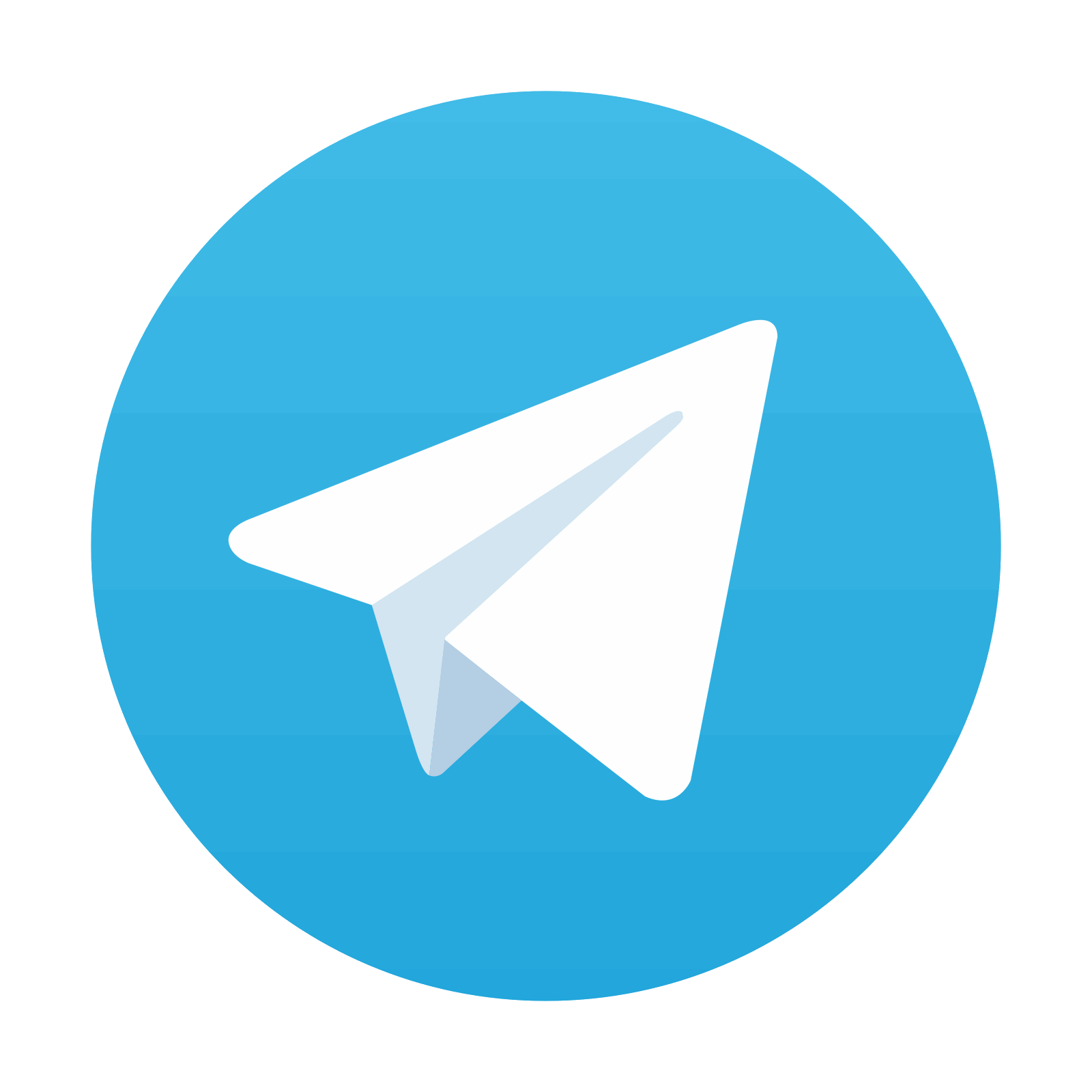
Stay updated, free articles. Join our Telegram channel

Full access? Get Clinical Tree
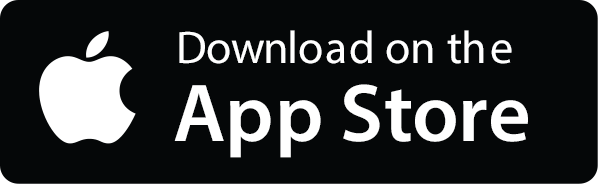
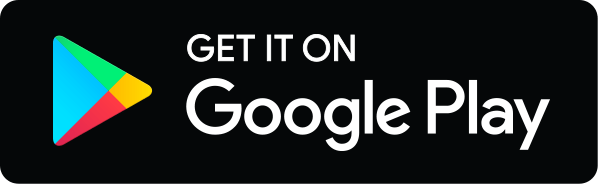