CHAPTER 129 Management of Single Ventricle and Cavopulmonary Connections
TERMINOLOGY AND ANATOMY
In this chapter, the term single ventricle refers to congenital cardiac malformations that lack two completely well developed ventricles, and in which functionally there is only a single ventricular chamber that supports both the pulmonary and systemic circulations. A truly morphologic univentricular heart is rare; more often, there is an additional rudimentary chamber. Over the years, a number of classifications and terminologies for these hearts have been suggested. The Congenital Heart Surgery Nomenclature and Database Project minimum dataset categorized single ventricles into seven broad categories1: hearts with common inlet atrioventricular connection (double-inlet right ventricle and double-inlet left ventricle), hearts with absence of one atrioventricular connection (tricuspid atresia and mitral atresia), hearts with common atrioventricular valve and only one well-developed ventricle (unbalanced common atrioventricular canal defect), hearts with only one fully developed ventricle and heterotaxia syndrome (single-ventricle heterotaxia syndrome), and finally other rare forms of univentricular hearts that do not fit in one of the above categories. A more comprehensive classification of the Congenital Heart Surgery Nomenclature and Database Project subclassifies these categories of single ventricle into four hierarchies spanning over three printed pages.2 Tricuspid atresia is the most common type of single ventricle, with an incidence of 1% to 3% of all congenital heart lesions.
The management of hypoplastic left heart syndrome, a common form of univentricular heart with a dominant right ventricle and a rudimentary left ventricle, is not discussed in this chapter. Chapter 28 is dedicated to this topic.
SURGICAL PREPARATION FOR THE FONTAN CIRCULATION
Inadequate Pulmonary Blood Flow
In the neonatal and early infantile period, when pulmonary vascular resistance is still high, if the child is ductal dependent or has inadequate systemic oxygen saturations, a systemic-to-pulmonary-artery shunt is performed, usually a modified Blalock-Taussig shunt.3 Although this was classically performed through a thoracotomy approach, a sternotomy is now commonly used because it allows concomitant ductal ligation and correction of stenosis of the proximal left pulmonary artery if present.4,5 Despite decades of experience with the modified Blalock-Taussig shunt, a persistent early and intermediate morbidity and mortality remains with this procedure.6
Excessive Pulmonary Blood Flow
In the case of excessive pulmonary blood flow, it is imperative to limit the pulmonary blood flow, usually with a pulmonary artery band, to protect the patient from developing pulmonary vascular disease and ventricular dysfunction caused by chronic volume overload.7 Adequate tightness of the band can be difficult to achieve, and a band that is too loose initially may result in unprotected pulmonary arteries until the child grows into the band. The resultant pulmonary vascular disease may affect the ultimate suitability of the child for the Fontan procedure. Other complications of pulmonary artery banding include distortion of the pulmonary arteries, especially if the band migrates, and erosion of the band. Because of the fixed diameter of the pulmonary band, as the child grows, there will eventually be inadequate pulmonary blood flow with resultant cyanosis, necessitating further surgical intervention. Often in infants with unobstructed pulmonaryt blood flow and single-ventricle anatomy, a concomitant coarctation needs to be repaired at the time of pulmonary artery banding.
To avoid the complications of pulmonary artery banding in the neonate or infant with excessive pulmonary blood flow and single-ventricle physiology, Bradley and colleagues have suggested the strategy of pulmonary artery division with placement of a systemic-to-pulmonary-artery shunt with excellent clinical results.8
Systemic Outflow Obstruction (Subaortic Stenosis)
Some children with single ventricles present in infancy or later develop systemic ventricular outflow tract obstruction (subaortic stenosis). The resultant myocardial hypertrophy can adversely affect the suitability for a Fontan procedure. Patients at risk are those in whom the aorta arises above a small outlet chamber, such as in tricuspid atresia with double-inlet left ventricle, rudimentary right ventricle, and transposition of the great arteries, particularly if the ventricular septal defect (VSD) (sometimes referred to as a bulboventricular foramen) is small or if there is coexisting aortic arch obstruction.9 A modified Damus-Stansel-Kaye procedure should be performed to establish unobstructed systemic arterial outflow. This involves transection of both great arteries, anastomosis of the facing aortic and pulmonary walls, and connection of the distal aorta to the perimeter of the reconstructed proximal great artery. Depending on the pulmonary vascular status, blood flow to the central pulmonary arteries can be reestablished via a systemic-to-pulmonary-artery shunt, a bidirectional cavopulmonary anastomosis, or the Fontan operation.10 The Damus-Stansel-Kaye procedure can be performed as a primary procedure11 or after a previous pulmonary artery band.7,12,13 Alternatively, a Norwood strategy can be followed in these patients (see Chapter 28).14,15 Some have advocated direct relief of the systemic outflow obstruction by surgical enlargement of the VSD (bulboventricular foramen) or resection of subaortic stenosis.7,16,17
Obstructed Pulmonary Venous Return
A particularly unfavorable subset of patients with single ventricles is those with total anomalous pulmonary venous connection.18–20 Even with successful correction of the pulmonary venous return, these patients can have persistent pulmonary artery hypertension, probably related to fetal pulmonary vein and artery abnormalities,20 that precludes the application of the Fontan procedure. To a lesser extent, children with a restrictive atrial communication and an abnormal left atrioventricular valve (as in mitral atresia) are at risk for the development of pulmonary artery hypertension.21 Once this condition is recognized, one of the following should be performed: opening of the atrial septum by percutaneous atrial septostomy or stent placement in the catheterization laboratory, or surgical atrial septectomy.
BIDIRECTIONAL CAVOPULMONARY ANASTOMOSIS
The original cavopulmonary shunt was the Glenn procedure (anastomosis of the divided right pulmonary artery to the superior vena cava with ligation of the proximal superior vena cava).22 The advantages of a venous over an arterial shunt in the palliation of cyanotic heart disease are twofold. First, the venous blood that enters the pulmonary artery is much more desaturated, and therefore a higher uptake of oxygen per milliliter of blood is possible. Second, systemic venous return is diverted to the lungs, thus reducing the volume load on the single ventricle. The original Glenn anastomosis fell into disfavor because it could not be used in neonates with elevated pulmonary vascular resistance.23 There were also reports of the development of ipsilateral pulmonary arteriovenous malformations.24 However, it was noted that patients with a previous classical Glenn anastomosis fared better with a subsequent Fontan procedure.25 For patients considered at high risk for the Fontan procedure, a bidirectional cavopulmonary anastomosis (the divided superior vena cava anastomosed to the pulmonary arteries supplying both lungs) could be used successfully as an alternative to the Fontan or as a staging procedure for the Fontan (Fig. 129-1).26,27 The hemi-Fontan modification of the cavopulmonary shunt involves patch augmentation of the central pulmonary arteries and a connection between the right-atrium-to-superior-vena-cava junction and the pulmonary arteries. Norwood and Jacobs reported improved survival with the Fontan operation in children with hypoplastic left heart syndrome who had undergone an intermediate hemi-Fontan procedure.28 Subsequent studies have shown significantly improved outcomes in patients with a Fontan procedure who were staged with a bidirectional cavopulmonary anastomosis compared with those who were not staged.21,29 The bidirectional cavopulmonary anastomosis can be performed safely in children younger than 6 months30 and is now routinely performed electively between 4 and 6 months of age, even in children without prior surgical palliation.
Additional Sources of Pulmonary Blood Flow
Whether an additional source of pulmonary blood flow (such as a patent ductus arteriosus, a patent right ventricular outflow tract or a tight pulmonary artery band, or a systemic-to-pulmonary artery shunt) should be taken down at the time of the cavopulmonary artery shunt remains open to discussion. Comparisons of patients with and without sources of additional pulmonary blood flow after a bidirectional cavopulmonary anastomosis have shown no deleterious effect at the time of the eventual Fontan procedure.31–33 There is evidence that leaving an additional source of pulmonary blood flow after the bidirectional cavopulmonary anastomosis can enhance pulmonary arterial growth.32,34 Some patients cannot tolerate this increased pulmonary blood flow, which manifests as pleural effusions and superior vena cava syndrome. They can be managed by catheter-based techniques to occlude these sources of additional pulmonary blood flow.35
Some have argued that a bidirectional Glenn anastomosis with an additional source of pulmonary blood flow can serve as definitive palliation without a subsequent Fontan procedure, especially in high-risk cases.36,37 A recent multi-institutional paper from Italy reviewed 246 patients with a mean age of 4.7 ± 6.2 years (range, 12 months to 30 years) who underwent a bidirectional cavopulmonary anastomosis, leaving intact the antegrade accessory pulmonary blood flow.31 On intermediate follow-up of 4.2 ± 2.8 years, 173 patients (70.3%) have not required a completion Fontan procedure and have a mean resting arterial oxygen saturation of 87% ± 4%. The actuarial freedom from a Fontan procedure at 7 years was 70.2% for the entire cohort. These results suggest that this strategy provided sustained palliation for selected patients with a single-ventricle circulation, and they may indicate that the Fontan route is not necessarily the universal palliation for all single-ventricle circulations.
Bilateral Bidirectional Cavopulmonary Anastomoses
Approximately 15% of children with single ventricles undergoing a bidirectional cavopulmonary anastomosis will have a persistent left superior vena cava draining to the coronary sinus.38,39 Although an earlier report from the Hospital for Sick Children in Toronto suggested that the presence of a second superior vena cava was a risk factor for thrombosis and failure to progress to the Fontan procedure,39 a more recent report from our institution did not identify any adverse clinical outcomes in patients undergoing bilateral bidirectional cavopulmonary anastomoses compared with those with a unilateral bidirectional cavopulmonary anastomosis.38
Kawashima Operation and Pulmonary Arteriovenous Malformations
Some children with single-ventricle anatomy, particularly those with heterotaxy syndrome, have an interrupted inferior vena cava with azygos continuation to the right superior vena cava or hemiazygos continuation to a persistent left superior vena cava. After initial palliation in infancy as necessary, with pulmonary artery banding or a systemic-to-pulmonary-artery shunt determined by the status of the pulmonary blood flow, definitive palliation can be accomplished with a Kawashima operation.40 This consists of a bidirectional cavopulmonary anastomosis (bilateral in the case of a persistent left superior vena cava) without the division of the azygos (or hemiazygos) continuation. In effect, this routes all of the systemic venous return except hepatic and mesenteric flow to the pulmonary arteries. A significant percentage of these patients will develop pulmonary arteriovenous malformations (AVMs) in both lungs with resultant cyanosis. This is analogous to the ipsilateral pulmonary AVMs observed with the classic Glenn anastomosis.24 There seems to be some factor in the hepatic venous effluent that, if not circulated to the lung, results in the development of these AVMs.41 Rerouting the hepatic and mesenteric venous blood flow to the pulmonary arteries by completing the Fontan procedure has been shown to resolve this problem for most patients.42–44
Glenn Anastomosis versus Hemi-Fontan Procedure
As an intermediate stage to the Fontan procedure, either a bidirectional Glenn anastomosis or a hemi-Fontan procedure can be chosen. Although there are advocates for both operations, there are few studies comparing the two operations in similar groups of patients. The hemi-Fontan procedure, as described initially by Norwood and Jacobs,28 involves patch augmentation of the central pulmonary arteries and a connection between the right-atrium-to-superior-vena-cava junction and the pulmonary arteries with elimination of alternative sources of pulmonary blood flow. Although it is a more extensive operation, it simplifies the eventual Fontan procedure, particularly if a lateral tunnel Fontan is planned.45 It also has the potential advantage of allowing nonsurgical completion of the Fontan circulation in the cardiac catheterization laboratory,46 although this nonoperative Fontan completion can also be used with the bidirectional Glenn anastomosis.47 Sinus node dysfunction appears to be more common early after the hemi-Fontan procedure than the bidirectional Glenn anastomosis, but this was no longer significant at the time of hospital discharge.48 Using magnetic resonance imaging reconstructions and computational and experimental fluid dynamics methodologies, it appears that the bidirectional Glenn anastomosis is more energy efficient than the hemi-Fontan procedure.49
THE 1.5-VENTRICLE REPAIR
In children with a marginal pulmonary ventricle, the standard surgical options are a Fontan procedure or a high-risk biventricular repair, as is commonly seen for patients with pulmonary atresia with intact ventricular septum.50 To reduce the risk of biventricular repair and to combat the long-term attrition related to the “Fontan state,” Billingsley and colleagues proposed recruiting the hypoplastic pulmonary ventricle to manage part of the systemic venous return by closing the atrial communication, enlarging the right ventricular outflow tract, and adding a cavopulmonary anastomosis so that the small right ventricle is tasked with pumping only the venous return from the inferior vena cava and not the entire cardiac output.51 The concept of a 1.5-ventricle repair has since been extended to other abnormalities52–54 such as unbalanced atrioventricular septal defects and straddling atrioventricular valves, and to use as an adjunct to the repair of Ebstein’s anomaly.55 Strict criteria to distinguish which patients would benefit from a 1.5-ventricle repair as opposed to a biventricular repair or a Fontan procedure are not well defined.56
SELECTION CRITERIA FOR THE FONTAN PROCEDURE
The initial selection criteria for the procedure, described by Fontan and colleagues for patients with tricuspid atresia and known as the “ten commandments,” were stringent (Box 126-1).57 With increasing experience, the criteria have been relaxed; however, because of the unique physiology that accompanies the Fontan circulation, proper patient selection remains crucial.
Box 129–1 Selecting Patients with Tricuspid Atresia for the Fontan Procedure: The “Ten Commandments”
The age of patients undergoing the Fontan operation has been progressively lowered as experience has been gained. Increased pressure and volume load of the single ventricle, such as occurs after systemic-to-pulmonary-artery shunts, is a risk factor for subsequent failure of the Fontan operation, and therefore early ventricular unloading with a bidirectional Glenn anastomosis has been advocated. In short-term studies, regression of ventricular mass was observed in children less than 3 years old who underwent a bidirectional Glenn shunt, but not when the procedure was performed when the child was 10 years or older.58 Similarly, younger age at the time of bidirectional Glenn shunt or Fontan surgery was also associated with better exercise performance than when surgery was delayed until a later age.59 In contrast to Fontan’s initial restriction of a minimum age greater than 4 years, recent data show that for patients with a dominant left ventricle (e.g., with tricuspid atresia), with an average follow-up of greater than 7.5 years, postoperative exercise capacity as well as cardiac index and ventricular ejection fraction were significantly better in children who had a Fontan completion at an age less than 3 years compared with a group who were older than 3 years at the time of the Fontan operation.60 A multivariable analysis of risk factors after the Fontan operation in 406 patients did not identify young age as a risk factor for unfavorable outcome.61 Pizarro and colleagues demonstrated in 107 children that Fontan completion can be performed safely in children at a median age of 13 months.62 It remains to be seen if a very early Fontan procedure has a better long-term outcome than one performed at a later age, in particular in patients with well-balanced circulations. Certainly, current strategies of early unloading of the single ventricle with an intermediate bidirectional cavopulmonary anastomosis or hemi-Fontan procedure has allowed successful Fontan completion at a younger age.
Preoperative sinus rhythm is not an absolute requirement for a successful Fontan procedure,63,64 although patients without preoperative sinus rhythm are more likely to have heterotaxy syndrome, which is a predictor of post-Fontan rhythm disturbances.65,66 The requirement in Fontan’s “ten commandments” for normal right atrial volume or normal caval drainage no longer seems to be of concern. On the other hand, unobstructed pulmonary venous drainage is an absolute necessity (see Obstructed Pulmonary Venous Return, earlier).
Pulmonary vasculature and ventricular function remain the most important selection criteria for successful outcome after the Fontan operation.61 Values should be low for pulmonary arteriolar resistance (<4 units/m2)67 and mean pulmonary artery pressure (<15 mm Hg).61,66 Assessment of the pulmonary vascular bed can be very difficult, especially in the presence of accessory sources of pulmonary blood flow such as aortopulmonary collateral vessels or surgical shunts.
Small size of the central pulmonary arteries has been considered a predictor of poor outcome after the Fontan procedure (death or takedown). Various attempts have been made to standardize these measurements. The McGoon ratio, originally used in patients with pulmonary atresia and VSD,68 is obtained from the sum of the diameters of the left and right pulmonary arteries immediately before they branch, divided by the diameter of the descending aorta just above the diaphragm. In a retrospective study, Fontan and colleagues69 found that the risk of early death or Fontan takedown rose sharply when the McGoon ratio was less than 1.8. The Nakata pulmonary artery index70 is derived from the sum of the diameters of the left and right pulmonary arteries (measured just before the origin of the upper lobe branches) divided by the body surface area. Patients with unfavorable outcome after a modified Fontan for tricuspid atresia were shown to have a lower pulmonary artery index than those with good results (185 ± 47 versus 276 ± 83 mm2/m2),71 but this was not confirmed by others.72,73 The usefulness of these indices has been questioned because they do not take into account the compliance and maturity of the pulmonary vascular system, or of the peripheral and intraparenchymal pulmonary arteries, or any distortion of the central pulmonary arteries that may have occurred because of previous shunts or bands. Furthermore, many of these studies implicating small pulmonary artery size as a risk factor for poor Fontan outcomes were performed before current strategies of earlier staged Fontan procedures using total cavopulmonary connections.72
Adequate ventricular function remains a prime determinant for a successful Fontan circulation.61 The incidence of common causes of impaired systemic ventricular performance seen in the past can be reduced. For example, early elimination of volume overload by an intermediate bidirectional cavopulmonary connection or hemi-Fontan procedure can preserve ventricular function.45,74 Also, aggressive treatment of systemic ventricular outflow obstruction (see Systemic Outflow Obstruction, earlier) can reduce deleterious ventricular hypertrophy in these patients with resultant improved ventricular function at the time of the Fontan procedure. Also, some feel that the presence of systemic-to-pulmonary collateral artery connections can increase the volume load of the single ventricle with resultant impaired ventricular function or increased workload on the heart.75–78 The impact of these collaterals can be reduced in the cardiac catheterization laboratory by coil occlusion, although some groups question its usefulness.79–82
Atrioventricular valve insufficiency remains a risk factor for the Fontan procedure,83 particularly in children with heterotaxy syndrome.18,66 Because this can cause volume overload of the single ventricle with the potential for impaired ventricular function, it is important to address this surgically when possible. Repair of the incompetent systemic atrioventricular valve can be performed reliably at the time of the bidirectional cavopulmonary anastomosis or at the time of the Fontan.84–86 If necessary, atrioventricular valve replacement is an option.87
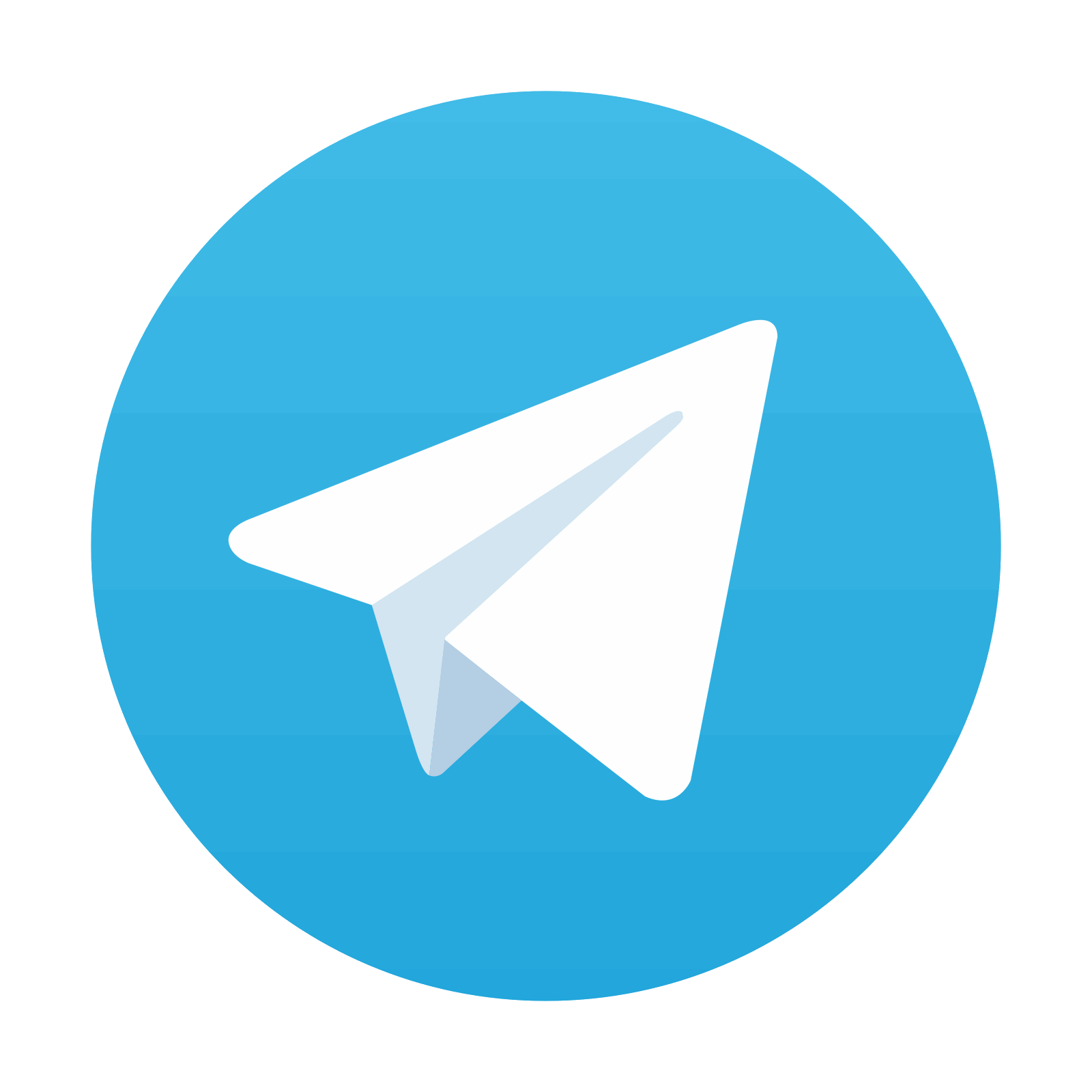