Chapter 24 Management of Renovascular Disease
Historical Background
Renovascular Hypertension
Goldblatt defined a causal relationship between renovascular disease and hypertension through his innovative work published in 1934,1 but Bright of Guy’s Hospital, London, first called attention to a potential association between hypertension and renal disease 100 years earlier.2 Bright observed that patients with “dropsy” and albuminuria during life had shrunken kidneys and an enlarged heart (cardiac hypertrophy) at autopsy. He suggested that the altered quality of the blood so affected the small circulation as to render greater action necessary to force the blood through the terminal divisions of the vascular system.
Although Bright failed to recognize the relationship between increased blood pressure and cardiac hypertrophy, his observations stimulated many theories. Among them was Traube’s speculation that elevated blood pressure led to increased myocardial work and subsequent hypertrophy.3 Based on Bright’s observations and subsequent hypotheses, several investigators described experimental models intended to re-create the clinical lesions observed in the kidney and heart. In 1879, Growitz and Israel4 produced acute occlusion of one renal artery and performed contralateral nephrectomy to decrease functioning renal mass. Although these investigators created what they thought was cardiac hypertrophy in some animals, elevated blood pressures occurred in none.
Lewinski might have predated Goldblatt’s observations had his experiments included blood pressure measurements.5 In 1880, he reported that 6 of 25 dogs developed cardiac hypertrophy after partial constriction of the renal arteries. In 1905, Katzenstein6 created hypertension in dogs by producing partial occlusion of the renal arteries, although complete occlusion after torsion of the renal pedicle did not produce elevated blood pressures. Katzenstein demonstrated that the elevated pressures returned to normal when constricting rubber bands were removed. However, he mistakenly concluded that the blood pressure changes were not related to a chemically mediated mechanism. In 1898, Tigerstedt and Bergman published the landmark description of a renal pressor substance in rabbits, a crude extract they termed renin.7 Although their work was confirmed in 1911 by Senator,8 these and other investigators did not consider renin central to the pathogenesis of hypertension.
In 1934, Goldblatt1 demonstrated that constriction of the renal artery produced atrophy of the kidney and hypertension in the dog. As a clinical pathologist, Goldblatt noticed that extensive vascular disease was often present at autopsy in patients with hypertension and was frequently severe in the renal arteries. In his own words: “Contrary, therefore, to what I had been taught, I began to suspect that the vascular disease comes first and, when it involves the kidneys, the resultant impairment of the renal circulation probably, in some way, causes elevation of the blood pressure.”1 Goldblatt’s elegant experiments introduced a new era by demonstrating that renal artery stenosis could produce a form of hypertension corrected by nephrectomy.
In 1938, Leadbetter and Burkland9 described the first successful treatment of this correctable form of hypertension. They cured a 5-year-old child with severe hypertension by removal of an ischemic ectopic kidney. The photomicrographs published from that renal artery specimen were the first documentation of a renovascular origin of hypertension. In subsequent years, numerous patients were treated by nephrectomy, based on the findings of hypertension and a small kidney on intravenous pyelogram. Smith reviewed 575 such cases in 1956 and found that only 26% of patients were cured of hypertension by nephrectomy.10 This led him to suggest that nephrectomy should be limited to strictly urologic indications.
In 1954, Freeman performed an aortic and bilateral renal artery thromboendarterectomy in a hypertensive patient, which resulted in resolution of the hypertension.11 This first cure of hypertension by renal revascularization, in combination with the widespread use of aortography, was followed by enthusiastic reports describing blood pressure benefit after renal revascularization.12–15 Nevertheless, by 1960 it became apparent that renal revascularization in hypertensive patients with renal artery stenosis was associated with a beneficial blood pressure response in fewer than half of individuals. These clinical results fostered general pessimism regarding the value of operative renal artery reconstruction for the treatment of hypertension.
Contemporary operative management of renovascular hypertension began with the introduction of tests of functional significance. Split renal function by Howard and Connor,16 Stamey and associates,17 Page and Helmes,18 and others19–21 identified the role of the renin-angiotensin system in blood pressure control, thus describing the pathophysiology of renovascular hypertension. After accurate assays for plasma renin activity became available, physicians could accurately predict which renal artery lesion was producing renovascular hypertension.
Renovascular Renal Insufficiency
Until the current era, the pathophysiology and management of renovascular disease focused solely on hypertension; however, contemporary reports have emphasized the relationship between renovascular disease and renal insufficiency.22–30
The term ischemic nephropathy has been adopted to recognize this relationship. By definition, ischemic nephropathy describes the presence of severe occlusive disease of the extraparenchymal renal artery in combination with excretory renal insufficiency. In 1962, Morris and associates reported on eight azotemic patients with global renal ischemia who experienced improved blood pressure and renal function after renal revascularization.31 Novick, Libertino, and Dean and their groups found a similar beneficial functional response when bilateral renal lesions were corrected in azotemic patients.24,25,27,28 These early reports and the reports that followed suggested that ischemic nephropathy could mediate renal insufficiency that was rapidly progressive, contributing to end-stage renal disease. In this chapter, diagnostic studies and methods of management for renovascular disease (RVD), renovascular hypertension (RVH), and ischemic nephropathy are reviewed.
Pathology
Occlusive lesions of the renal artery can be divided into two main categories: atherosclerosis and fibromuscular dysplasia. Atherosclerosis of the renal artery is not unique. The pathogenesis parallels atherosclerotic lesions elsewhere, with cholesterol-rich lipid deposition and intimal thickening. Later, this atheroma may undergo central degeneration and even calcification. Atheromas typically occur at or near the renal artery ostium (Figure 24-1). This ostial lesion reflects aortic atheroma that spills over into the renal artery orifice. Most commonly, these lesions are found on the left and account for approximately 70% of patients with RVH. Like atherosclerosis elsewhere, angiographic findings that are pathognomonic for a hemodynamically significant lesion include post-stenotic dilatation and the presence of collateral vessels. Often there is simultaneous angiographic involvement of the abdominal aorta and its bifurcation. Most commonly, the renal artery lesion is only one manifestation of generalized atherosclerosis.
Based on the angiographic appearance of fibromuscular disease, several methods of categorization have been suggested. To establish a uniform terminology, Harrison and McCormack32 combined their experience and developed a classification of these lesions correlating the histologic and angiographic appearance. Depending on the layer predominantly involved, lesions may be categorized as intimal, medial, or adventitial (Figure 24-2). Clinically, however, it may be difficult to segregate individual lesions into one of these categories. The most common variety of fibromuscular dysplasia is medial fibroplasia (85%) with mural microaneurysms (Figure 24-3). Less commonly, the dysplastic lesions may appear as a single mural stenosis (see Figure 24-2), which is consistent with intimal fibroplasia (5%). Perimedial dysplasia (10%) demonstrates the same gender predilection and arterial septa as medial fibroplasia; however, mural microaneurysms are absent.
Pathophysiology
Renin-Angiotensin-Aldosterone System
It is well accepted that RVH is caused by increased activity of the renin-angiotensin system produced initially by hypersecretion of renin from the juxtaglomerular apparatus of the ischemic kidney. However, as hypertension evolves into a chronic stage, adaptive cardiovascular changes may become an essential mechanism for the maintenance of elevated blood pressure and peripheral vascular resistance. The effect of hypertension on precapillary resistant vessels triggers a myogenic response that is evidenced by the combination of hypertrophy and hyperplasia of the vascular smooth muscle.33,34 This augments vascular reactivity to pressor agents. There is also evidence that renin may be trapped in structural elements of the vascular wall.35 Local production of angiotensin II by tissue renin-angiotensin systems may contribute to the remodeling of resistance vessels.36 Angiotensin-converting enzyme exists in the plasma membrane of vascular endothelial and smooth muscle cells. Thus, the necessary components for the production of angiotensin II may be found in both vascular and cardiac tissue. In the chronic phases of the hypertension process, hypersecretion of renin from the ischemic kidney may be less important than increased production of vascular angiotensin II as the mechanism that sustains the elevation in arterial pressure.
The pathophysiology of renovascular renal insufficiency (i.e., ischemic nephropathy) is incompletely understood.37 The earliest clinical reports suggested a “glomerular filtration failure” based on hypoperfusion of the kidney,38 but the molecular basis for ischemic nephropathy is poorly characterized. Like RVH, the renin-angiotensin system likely contributes to ischemic nephropathy through its paracrine effects—intrarenal angiotensin peptides increase efferent arteriolar tone.39 In the presence of a pressure-reducing renal artery lesion, this paracrine effect increases glomerular capillary pressure to support glomerular filtration. In contrast to these positive effects, angiotensin peptides have also been shown to promote tubulointerstitial injury in the presence of a renal artery lesion.40 This observation is supported by the induction of transforming growth factor-β and interstitial platelet-derived growth factor-β, which are associated with increased extracellular matrix and interstitial fibrosis.41–44 Disruption of the tubular cell cytoskeleton and the loss of tubular membrane polarity have also been suggested. Besides these potentially reversible contributors to excretory renal insufficiency, an atherosclerotic renovascular lesion can also be a source of atheroemboli.45 The inability to distinguish potentially reversible ischemic nephropathy from irreversible renal parenchymal disease has enormous clinical importance. Recovery of renal function after renovascular intervention has proved to be the strongest predictor of dialysis-free survival.23,26
Prevalence of Renovascular Hypertension and Ischemic Nephropathy
RVH is generally thought to account for 5% to 10% of the hypertensive population. Tucker suggested an even lower prevalence.46 Likewise, Shapiro and colleagues47 suggested that the identification and successful operative treatment of RVH in patients older than 50 years are so unlikely that diagnostic investigation for a correctable cause in that group should be undertaken only when hypertension is severe and uncontrollable. Estimates of the prevalence of hypertension in the United States from all causes range from 60 to 80 million people, and hypertension may be present in 25% to 30% of the adult population.
The actual contribution of RVD to hypertension or renal insufficiency has been uncertain because the population-based prevalence of RVD was unknown. Past prevalence estimates of RVD were extrapolated from case series, autopsy examinations, or angiography obtained to evaluate diseases of the aorta or peripheral circulation.31,48–54
Recently, the population-based prevalence of RVD has been estimated for participants in the Cardiovascular Health Study (CHS) sponsored by the National Heart, Lung, and Blood Institute. The CHS is a longitudinal, prospective, population-based study of coronary heart disease and stroke in elderly men and women. This study showed that hemodynamically significant RVD was present in 6.8% of this elderly, free-living cohort.55 Multivariate analysis demonstrated that increasing participant age (p = 0.028; odds ratio [OR], 1.44; 95% confidence interval [CI], 1.03 to 1.73) and increasing systolic blood pressure at baseline (p = 0.007; OR, 1.44; 95% CI, 1.10-1.87) were significantly and independently associated with the presence of RVD. Moreover, renal insufficiency was associated with RVD, but only when renal artery disease coexisted with significant hypertension. Contrary to historical assumptions, RVD demonstrated no significant relationships with gender or ethnicity.55
Like in the general population, the incidence of RVH is undoubtedly low when all patients with hypertension are considered. Because RVH tends to produce relatively severe hypertension, its prevalence in the large population of mildly hypertensive patients (diastolic blood pressure <105 mm Hg) is probably negligible. In contrast, however, it is a frequent cause of hypertension in the smaller group of patients with severe hypertension. In our experience, the presence of severe hypertension at the two extremes of life carries the highest probability of its being RVH. Our review of the causes of hypertension in 74 children admitted for diagnostic evaluation over a 5-year period showed that 78% of the children younger than 5 years had a correctable renin-dependent cause (Table 24-1).56 In 1996, our center screened 629 hypertensive adults older than 50 years for RVD (Table 24-2). Overall, 25% of subjects demonstrated significant renal artery disease. However, 52% of those older than 60 years whose diastolic pressure was greater than 110 mm Hg had significant renal artery stenosis or occlusion. When serum creatinine was elevated in conjunction with this age and blood pressure, 71% of subjects demonstrated hemodynamically significant RVD.
Characteristics of Renovascular Hypertension
Because of the small proportion of RVH among the entire hypertensive population, many reports have focused on the value of demographic factors, physical findings, and screening tests to discriminate between essential hypertension and RVH. Most frequently cited as discriminate factors suggesting the presence of RVH and a need for further study are recent onset of hypertension, young age, lack of family history of hypertension, and presence of an abdominal bruit. The most complete study comparing the clinical characteristics of patients with RVH to those with essential hypertension was the Cooperative Study of Renovascular Hypertension.57 In that study, the prevalence of certain clinical characteristics in 339 patients with essential hypertension was compared with their prevalence in 175 patients with RVH secondary to atherosclerotic lesions (91 patients) and fibromuscular dysplasia (84 patients). Although the prevalence of several characteristics was significantly different in RVH compared with essential hypertension, none of the characteristics had sufficient discriminant value to be used to exclude patients from further diagnostic investigation for RVH. Certainly, the finding of an epigastric bruit in a young white female with malignant hypertension is strongly suggestive of a renovascular origin of the hypertension. The absence of such criteria, however, does not exclude the presence of RVH, and such criteria should not be used to eliminate patients from further diagnostic study.
In a review of the first 200 patients with RVH treated in our center, 64% had family histories of hypertension, 46% had no audible abdominal bruit, and ages ranged from 5 to 80 years (mean, 56 years).58 Because RVH can be secondary to any of several diseases affecting the renal artery, and because each of these diseases has its own clinical characteristics, the use of demographic or physical findings such as age, abdominal bruit, and duration of hypertension to exclude patients from study inappropriately excludes some patients with RVH from further evaluation. Therefore, the decision to undertake diagnostic study should be based on the severity of hypertension. Mild hypertension has a minimal chance of being renovascular in origin. In contrast, the more severe the hypertension, the higher the probability that it is from a correctable cause. With this in mind, we evaluate all adult patients with diastolic blood pressures greater than 105 mm Hg who would be considered for intervention to evaluate for a renovascular lesion as a correctable origin of hypertension. Children are evaluated when their blood pressure exceeds the 95th percentile for height and age.59
Natural History of Atherosclerotic Renovascular Disease
The rationale provided for renal artery intervention frequently cites the presumed natural history of the atherosclerotic renal artery lesion. Much of the information regarding atherosclerotic renovascular disease and its natural history has been extrapolated from angiographic case series and ultrasound examinations from retrospective reviews or from prospective studies in select hypertensive patients. Although the quality of these studies and their interpretation vary widely, most commonly, anatomic progression of atherosclerotic renovascular disease has been considered a certainty, one that is associated with inevitable decline in kidney size and kidney function. This “natural history” of atherosclerotic renovascular disease has been cited to support intervention for disease when encountered.60
Prospective angiographic clinical studies of atherosclerotic renovascular disease are summarized in Table 24-3. Forty years ago, Dean61 reported on patients with renovascular hypertension randomized to medical management or open surgical repair. Forty-one patients were randomized to medical management with high grade, atherosclerotic renal artery stenosis, hypertension, and positive renal vein renin assay or split renal function studies. Over an average of 44 months, 17 patients crossed over to the surgical arm. Each of these patients had controlled hypertension, but 15 demonstrated declining renal function as demonstrated by 10% loss in renal length, a 100% increase serum creatinine level, or 50% reduction in measured glomerular filtration rate. Among medically treated patients, 54% demonstrated a 50% decline in glomerular filtration rate; however, 13% demonstrated improved renal function when measured serially. Overall, the decline in renal function was variable with 97% of patients experiencing less than 50% decline in glomerular filtration.
TABLE 24-3 Prospective Angiographic Natural History Studies of Atherosclerotic Renal Artery Stenosis
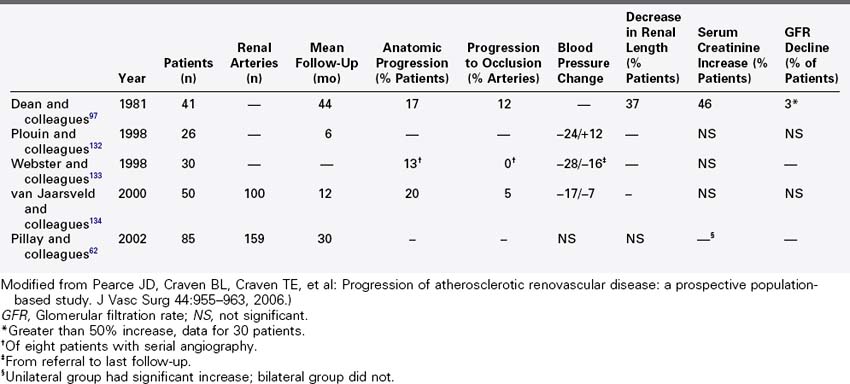
A recent prospective study by Pillay and colleagues62 described the change in blood pressure and serum creatinine among patients with atherosclerotic renovascular disease. Ninety-eight patients in this multicenter, nonrandomized observational study were noted to have greater than 50% renal artery stenosis during aortography to evaluate peripheral vascular disease. On a minimum duration of 2 years of follow-up, 64 patients with unilateral renal stenosis and 21 patients with bilateral disease were managed medically. Twelve patients with bilateral disease underwent percutaneous intervention or open operative repair. The overall 2-year estimated mortality was 32%. Mortality was equivalent for patients treated with renal artery intervention and for those treated medically. There was no change in median blood pressure, number of anti-hypertensives, or change in renal size in follow-up among survivors. A small but statistically significant increase in serum creatinine was observed in patients who underwent renal artery intervention. Patients with bilateral renovascular disease treated medically had stable serum creatinine over 2 year follow-up. This study lacked a specific measure of glomerular filtration; however, it demonstrated stable length and stable serum creatinine with controlled hypertension in medically managed patients who had anatomic disease but no clinical indication for renovascular hypertension.
Prospective duplex ultrasound studies are summarized in Table 24-4. A series of consecutive reports described prospective duplex studies performed at the University of Washington. These authors first reported on serial duplex examinations performed on 80 patients with hypertension. Renal arteries were classified according to four categories: normal, stenosis less than 60%, stenosis greater than 60%, or renal artery occlusion. The rate of progression to greater than 60% stenosis during 3 years of follow-up was 8% for renal arteries that were initially classified as normal and 43% initially classified as having less than 60% diameter reducing stenosis. Incident renal artery occlusions were observed only in arteries previously categorized as having 60% diameter reducing stenosis. The 3-year risk for occlusion among the entire group was 7%. Lesion progression was associated with increasing patient age, increasing systolic blood pressure, smoking, female sex, and poorly controlled hypertension.
TABLE 24-4 Prospective Duplex Sonography Natural History Studies of Atherosclerotic Renal Artery Stenosis
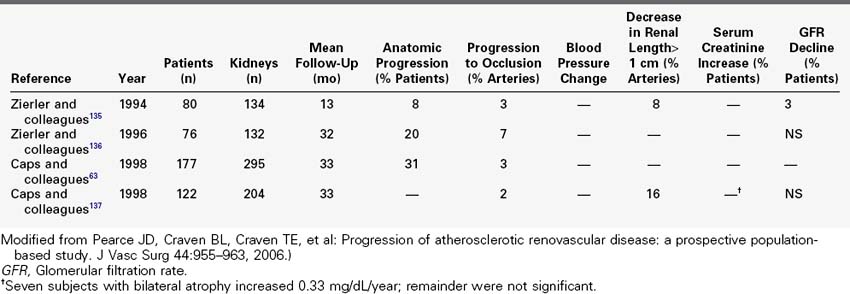
A most informative prospective study of atherosclerotic renovascular disease among hypertensive patients utilizing renal duplex was provided by Capps and colleagues,63 who described 5-year follow-up for 170 patients with 295 kidneys. Disease progression was defined by 100 centimeter per second increase in renal artery peak systolic velocity or progression to renal artery occlusion. By these criteria, disease progression was detected in 31% of renal arteries; 3% of arteries progressed to occlusion and all of these were considered to be diseased at baseline. A model to predict the 2-year calculated risk of progression included diabetes and systolic blood pressure greater than 160 mm Hg.
The data reviewed in the reports above are frequently cited as rationale for intervention for atherosclerotic renovascular disease discovered incidentally during assessment for cardiac or other vascular diseases. However, more recent prospective duplex studies suggest otherwise, at least in patients without severe associated hypertension. Davis and colleagues64 reported on 119 participants in the Cardiovascular Health Study with 235 kidneys followed over an 8-year period. None of these subjects demonstrated severe hypertension. Controlling for within subject correlation, the overall estimated change in renovascular disease among all 235 kidneys was 14% (95% CI, 9.2%-21.4%) with hemodynamically significant renovascular disease in 4% (95% CI, 1.9%-8.2%).64 No hemodynamically significant stenosis at baseline progressed to renal artery occlusion on follow-up. Increased blood pressure and decrease in renal length were not associated with renovascular disease at baseline examination. These findings cast doubt on the value of renal artery intervention in the absence of renovascular hypertension or renal insufficiency.
Diagnostic Evaluation
Screening Studies
Captopril Renal Scanning
To understand the basis of captopril renal scanning, one must consider some of the features of renal physiology and the importance of the renin-angiotensin system in the maintenance of homeostasis. Glomerular filtration is governed partially by the relative tone of the afferent and efferent arterioles. During periods of reduced blood pressure recognized by the juxtaglomerular apparatus (e.g., proximal renal artery stenosis), increased renin is released, which ultimately leads to an increase in the level of intrarenal angiotensin II. Angiotensin II acts predominantly to constrict the efferent arteriole to maintain renal glomerular perfusion pressure and filtration. When an angiotensin I–converting enzyme inhibitor such as captopril is given in this circumstance, an acute reduction in the amount of angiotensin II occurs. This leads to a reduction in constriction of the efferent arteriole and a decrease in the glomerular filtration rate (GFR). Captopril renal scanning is composed of a baseline renogram and a repeat renogram obtained after a dose of captopril. A test is considered positive when a normal baseline scan becomes abnormal after captopril administration—that is, when the time to peak activity increases to more than 11 minutes or when a normal glomerular filtration ratio between the two sides increases to greater than 1.5 : 1 (Figure 24-4). In experienced hands, this study is reported to be highly reliable.65 Unfortunately, it is less reliable when significant parenchymal disease is present. For that reason, we do not rely on its results to eliminate RVD in azotemic patients.
Renal Duplex Sonography
Through continued improvements in probe design and duplex sonographic technology, imaging and Doppler shift interrogation of deep abdominal vasculature have been introduced as screening methods to identify and quantify visceral and renal artery occlusive disease. We have evaluated the role of duplex sonography as an initial surface screening test, as an intraoperative study to confirm the technical success of reconstructive procedures, and as a postoperative surveillance method to follow progression of disease and stability of reconstructions.59,66–69
Technically successful studies, defined as a complete main renal artery interrogation from aortic origin to renal hilum, can be obtained in almost 95% of cases. These results are ensured by proper patient preparation and method of examination. Details of the conduct of the procedure are covered elsewhere.66
The criteria for identifying renal artery stenosis by renal duplex sonography are given in Table 24-5. Assuming that renal artery peak systolic velocity (PSV) varies with the degree of renal artery stenosis and aortic PSV (i.e., inflow), most authors have advocated using the ratio of renal artery PSV to aortic PSV (the renal-to-aortic ratio) to define critical renal artery stenosis.70–73 In contrast, we have found no relationship between renal artery PSV and aortic PSV in the presence or absence of disease (Figure 24-5). Focal renal artery PSV of 1.8 m/s or more in combination with distal post-stenotic turbulence correlates highly with the angiographic presence of diameter-reducing renal artery stenosis of 60% or greater.66 In 122 kidneys with single renal arteries and renal angiography for comparison, renal duplex sonography correctly identified 67 of 68 kidneys with normal arteries or less than 60% renal artery stenosis, and 35 of 39 kidneys with 60% to 99% renal artery stenosis. All 15 renal artery occlusions were correctly identified by failure to obtain a Doppler-shifted signal from an imaged renal artery. Using this method and these criteria for interpretation, renal duplex sonography was 93% sensitive and 98% specific.
TABLE 24-5 Doppler Velocity Criteria for Significant Stenosis and Occlusion
Defect | Criteria |
---|---|
<60% diameter-reducing RA defect | RA-PSV from entire RA < 1.8 m/s |
≥60% diameter-reducing RA defect | Focal RA-PSV ≥ 1.8 m/s and distal turbulent velocity waveform |
Occlusion | Doppler-shifted signal from RA B-scan image |
Inadequate study for interpratation | Failure to obtain Doppler samples interpretation from entire main RA |
RA, Renal artery; RA-PSV, renal artery peak systolic velocity.
From Hansen KJ, Tribble RW, Reaves SW, et al: Renal duplex sonography: evaluation of clinical utility. J Vasc Surg 12:227–236, 1990; and Motew SJ, Cherr GS, Craven TE, et al: Renal duplex sonography: main renal artery versus hilar analysis. J Vasc Surg 32:462–471, 2000.
Renal Angiography
Details of performing angiography to evaluate aortic and branch disease are addressed elsewhere. Currently, intraarterial digital subtraction angiography is used to evaluate the majority of patients. The fact that angiography in patients with severe renal insufficiency, especially those with concomitant diabetes mellitus, can aggravate renal failure is widely recognized. Nevertheless, we believe that this risk is justified in patients with severe or accelerated hypertension and in those with positive renal duplex sonography results. In these circumstances, the potential benefit derived from the identification and correction of a functionally significant renovascular lesion exceeds the risk of contrast exposure. In addition, the use of carbon dioxide and gadolinium as alternatives may reduce the risk of nephrotoxicity.74,75 However, gadolinium should be used with caution in patients with chronic renal insufficiency due to its association with nephrogenic systemic fibrosis.
Because the mortality and the morbidity of contrast nephropathy leading to dialysis dependence are high, measures to protect renal function during angiography should be taken. Conventional contrast agents have iodine incorporated into their structure to absorb x-ray photons, thereby achieving visualization of the vasculature. The nephrotoxicity of such iodinated contrast agents has been recognized for many years. The principal site of contrast-induced nephrotoxicity is the renal tubule from transient regional renal ischemia, whereas the effect on glomerular function appears to be mild.76,77 It was thought that the ionization and high osmolarity of early contrast agents may have contributed to their nephrotoxicity. Nonionic contrast agents (e.g., iohexol) are available that provide comparable absorption of x-ray photons, but are significantly less charged than traditional agents. It was hoped that the reduced ionic nature would decrease their nephrotoxicity, but the occurrence of severe adverse renal events did not differ between ionic and nonionic contrast media in a large randomized clinical trial.78
Renal nephrotoxicity after exposure to ionic agents occurs most commonly in patients with preexisting renal insufficiency (3.3 relative risk) alone or in combination with diabetes mellitus, especially juvenile-onset diabetes.78 Other risk factors such as dehydration, volume of contrast used, and simultaneous exposure to other nephrotoxins contribute to the likelihood of acute contrast nephrotoxicity.79 Other risk factors include multiple myeloma and heavy proteinuria. Overall, the incidence of acute renal dysfunction following contrast angiography varies from 0% to 10%, although these estimates are skewed by several studies that included only juvenile diabetics. In one study, hospital-acquired nephropathy occurred in 12% of patients.80 In patients with normal renal function, however, the incidence of contrast nephropathy is only 1% to 2%.81 The effect of diabetes on the risk of acute renal failure following angiography appears to be dependent on the type of diabetes and the magnitude of secondary diabetic nephropathy.
Patients with type 1 diabetes appear to be more susceptible to contrast-induced acute renal failure than do those with type 2 diabetics. Harkonen and Kjellstrand82,83 found that 20 of 26 patients (76%) with a prestudy serum creatinine level greater than 2 mg/dL who underwent excretory urography also developed acute renal failure.83 Weinrauch and associates84 reported that acute renal failure following coronary angiography developed in 12 of 13 patients (92%) with juvenile-onset diabetes and severe diabetic nephropathy. In addition, the cause of chronic renal insufficiency appears to affect recovery from contrast-induced acute renal failure. Whereas both diabetic and nondiabetic patients with renal insufficiency are at increased risk for contrast-induced acute renal failure, diabetics appear to recover less often and are at greater risk of permanent dependence on dialysis as a consequence of contrast-induced acute renal failure.82–84
Specific measures to minimize the risk of contrast-induced acute renal failure remain controversial, and the results from controlled studies are largely inconclusive. Nevertheless, the basic relationship between the use of contrast material and the risk of contrast nephropathy appears to be related to the amount of time the kidney is exposed to the contrast material. For this reason, maximizing urine flow rate during and immediately after angiography and limiting the quantity of contrast agent used are important considerations. Maximal urine flow rate should be achieved by preliminary intravenous hydration of the patient. Studies that examined the optimal preparation of patients with renal insufficiency indicated that hydration with 0.45% saline provides better protection against acute decline in renal function associated with radiocontrast agents than does hydration with 0.45% saline plus mannitol or furosemide.85 Recent evidence suggests that administration of sodium bicarbonate before contrast exposure may significantly reduce renal dysfunction.86
By scavenging reactive oxygen species, acetylcysteine may protect against contrast-induced nephrotoxicity. Tepel and colleagues87 studied patients with chronic renal dysfunction who required nonionic contrast for computed tomography. They documented a significant reduction in serum creatinine with the use of oral acetylcysteine and hydration compared with placebo and hydration.
Although further study is needed to better define the role of acetylcysteine during aortography, we administer two oral doses of acetylcysteine (600 mg) before and after these studies in patients at high risk for contrast nephropathy.68 We routinely admit any patient at risk for contrast nephropathy 12 hours before angiography for intravenous hydration at 1.5 mL/kg per hour. The patient receives two 600-mg oral doses of acetylcysteine immediately before angiography and a bolus of intravenous fluid (3 to 5 mL/kg) with or without sodium bicarbonate. Finally, intravenous hydration is continued for 4 to 6 hours, and two additional doses of acetylcysteine are administered after completion of the study.
Although attempts to calculate a safe upper limit of contrast material have met with some success, no definitive limit currently exists. Even small doses (30 to 60 mL) may induce renal failure in patients with extreme renal insufficiency (GFR ≤ 15 mL/min). Conversely, more than 300 mL of contrast material can be administered safely to other patients with no risk factors for acute renal failure.83 The authors’ practice is to limit the quantity of non-ionized contrast agent to less than 50 to 75 mL in patients with a significant reduction in GFR (<20 to 30 mL/min).88 If additional contrast material is required to complete the vascular evaluation, we postpone further study and approach the total evaluation in a sequential manner. In almost all instances, digital subtraction techniques have been useful in limiting the quantity of contrast material required. A single midstream aortic injection, using 10 to 20 mL of low-osmolar, nonionic contrast material and digital subtraction, provides images approaching the quality of prior conventional cut-film studies.
Adjuncts or alternatives to angiography are appropriate in many instances. In addition to the use of digital subtraction techniques, carbon dioxide gas can be used for angiography with minimal renal risk.89 Because it offers limited detail, carbon dioxide angiography is often used to identify the site of disease, which is then better defined with conventional contrast agents. Other alternatives to conventional angiography that reduce or eliminate the risk of nephrotoxicity include the use of gadolinium as a contrast agent for angiography,74,75 magnetic resonance angiography,90 and abdominal ultrasonography with visceral or renal artery duplex sonography.
Finally, high-dose loop diuretics, angiotensin-converting enzyme inhibitors, and angiotensin II receptor antagonists are held for at least 72 hours before aortic reconstruction or exposure to arterial contrast agents. Selective β-blockers and calcium channel blockers are substituted when necessary.91
Both aortography and selective renal angiography using multiple projections may be necessary to adequately examine the entire renal artery. The proximal third of the left renal artery usually courses anteriorly, the middle third transversely, and the distal third posteriorly, whereas the right renal artery arises from the anterior aorta and then pursues a more consistent posterior course. Lesions in the renal artery that are coursing anteriorly or posteriorly are frequently not seen or may appear insignificant in an anteroposterior aortogram. Oblique aortography or oblique selective renal angiography projects these portions of the vessels in profile and reveals the stenosis. Figure 24-6 illustrates how the delicate septal lesions of fibromuscular dysplasia may be unrecognizable or appear insignificant in the anteroposterior projection, whereas the true severity is demonstrated in the oblique projection.
Functional Studies
Two tests—renal vein renin assays and split renal function studies—have proved valuable in confirming the functional significance of renal artery stenosis. Neither has great value, however, when severe bilateral disease or disease in the renal artery supplying a single kidney is present. In these circumstances, the decision for operation is based on the severity of hypertension and the degree of renal insufficiency. Furthermore, urologically performed split renal function studies are no longer done in any center studying patients with RVH. The reader is referred to earlier texts describing their use.92,93
Renal Vein Renin Assays
The effect of antihypertensive medications and unrestricted sodium intake on renin release, and thereby the RVRA, is widely recognized. Many antihypertensive medications, especially those that function through β-adrenergic blockade, suppress renin output and can lead to false nonlateralization of the RVRA. Before presuming that no drug is interfering with the release of renin, all such medications must be withheld for at least 5 days—preferably 2 weeks—before the RVRA. Similar effects on renin levels are seen when sodium intake is not restricted. For this reason, the patient must be on no more than a 2-g sodium diet for at least 2 weeks before the study. The preparation of patients for RVRA is summarized in Box 24-1.
Box 24-1
Patient Preparation for Renal Vein Renin Assay
1. Chronic salt restriction (2-g sodium diet daily)
2. Discontinuance of all antihypertensive drugs except diuretics and calcium channel for at least 5 days before the study
3. Oral furosemide (40 mg) diuresis the night before the study
4. Nothing by mouth for 8 hours before the study
5. Strictly flat bed rest for 4 hours before and during the study
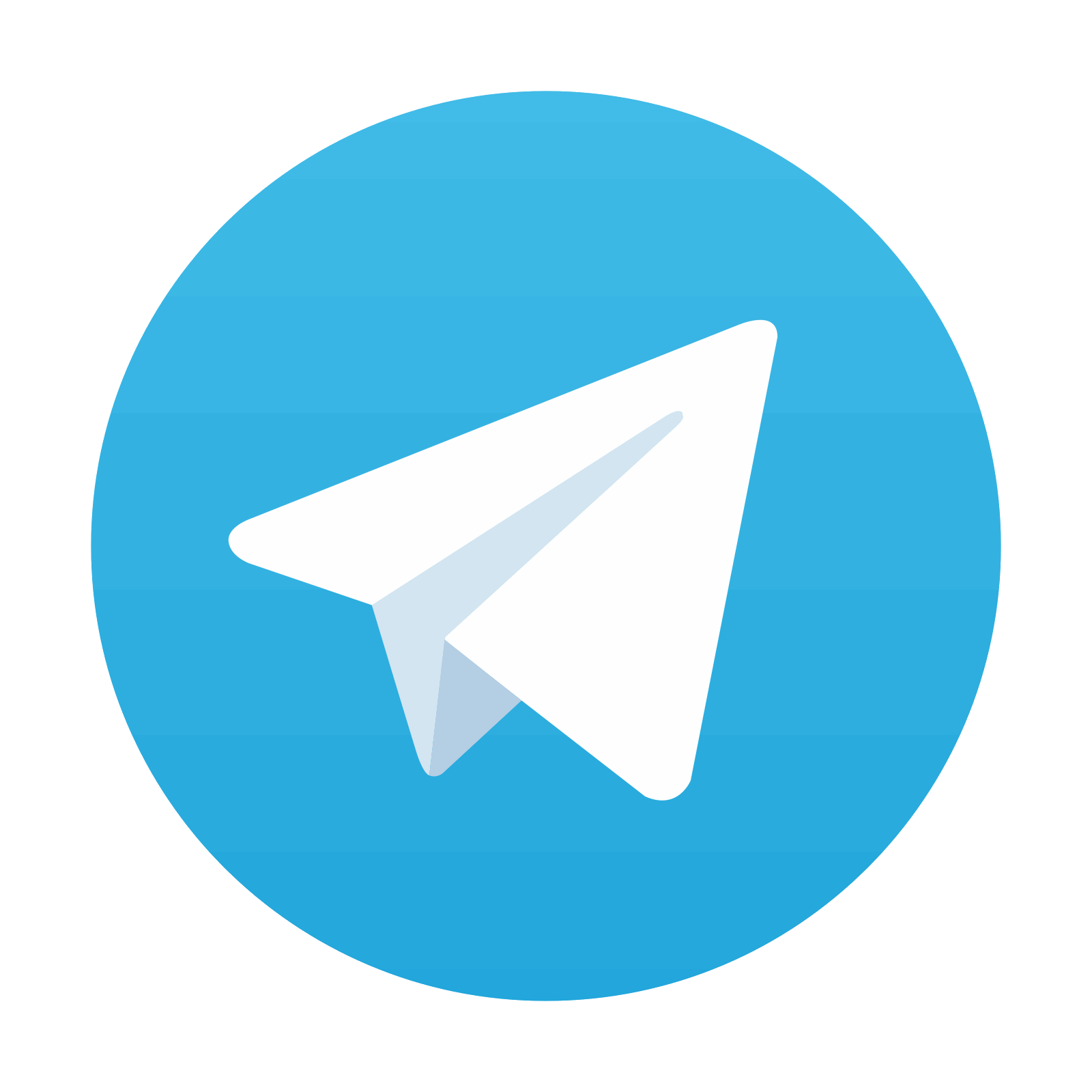
Stay updated, free articles. Join our Telegram channel

Full access? Get Clinical Tree
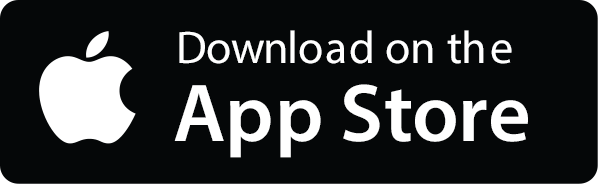
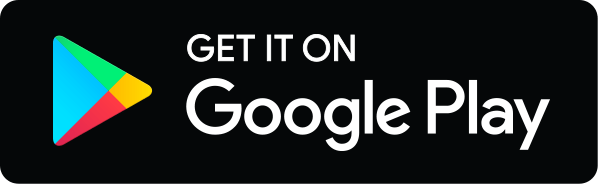