Management of Pneumothorax and Bullous Disease
Stephen D. Cassivi
Claude Deschamps
The pleural space is a potential space during normal conditions with the visceral pleura directly in apposition to the parietal pleura. Pneumothorax is defined as the presence of air in the pleural space and can be due to a number of causes. The etiology and volume of the pneumothorax and the resultant intrapleural pressure and condition of the underlying lung play a role in determining the clinical severity.
This chapter outlines the anatomy and basic physiology of the pleural space. The pathophysiology of the various etiologies of pneumothoraces is discussed, as are the diagnosis and management options. Special attention is directed to the particular condition of bullous disease.

The pleural space is lined by the visceral and parietal pleurae (Fig. 12.1). The visceral pleura is a thin layer (usually one cell thick) intimately covering the outer surface of the lung. It adheres to the underlying alveolar walls of the lung parenchyma via connective tissue made up of elastic fibers. There is, therefore, no true cleavage plane between the visceral pleura and the lung parenchyma that it envelops. The visceral pleura has no somatic innervation.
The parietal pleura is a more complex serous membrane. It lines the inside of the chest wall, the diaphragm, and the mediastinum and is attached to these by a fibrous and connective tissue layer known as the endothoracic fascia. Between the endothoracic fascia and the parietal pleura is a dissection plane that allows the parietal pleura to be stripped off of the chest wall and other structures. It is thickest and most substantial along the chest wall, overlying the ribs, and thinnest as it covers the mediastinal structures and beneath the sternum. The parietal pleura is innervated by somatic, sympathetic, and parasympathetic nerve fibers via the intercostal nerves.
PHYSIOLOGY
The physiology of the pleural space is relatively straightforward, although dynamic. Functional residual capacity is the measure of lung volume with the patient at rest after normal exhalation. In this state, the elastic and retractive nature of the chest wall and lung pull the parietal and visceral pleurae away from one another, thus creating a negative intrapleural pressure usually in the range of -2 to -5 cm H2O. During inspiration, the outward chest wall and diaphragmatic forces counteracting the normal elastic recoil of the lung parenchyma can create intrapleural pressures of -20 to -35 cm H2O. Gravity also exerts an influence on this negative intrapleural pressure. In the upright position, the apex has a greater negative intrapleural pressure than the base of the lung in the region of the costophrenic sulci (0.25 cm H2O/cm of height). This phenomenon may contribute to some degree to creating increased distention of alveoli in the apex and a greater predisposition to spontaneous pneumothoraces by rupture of apical blebs.
As a consequence of having more oxygen consumed than carbon dioxide produced during the respiratory cycle (respiratory quotient <1), there is a resultant partial pressure gradient between the gases in the venous blood and those of the arterial system and pleural space. This gradient, usually between 54 and 72 cm H2O, ensures against spontaneous gas formation in the pleural space as long as the intrapleural pressures do not become less than -72 cm H2O. On a more practical level, this also explains how intrapleural air, as in the case of a pneumothorax, can be gradually reabsorbed by diffusion into the venous circulation.
Pleural gases can also be affected by barometric pressure. Whereas the relative proportions of gases do not change with variation in atmospheric pressure, there can be a significant change in the volume of these gases. Boyle’s law states that at constant temperature, for a given mass of gas, pressure p multiplied by volume V is a constant:
pV = c
Thus, the volume change in a gas is inversely proportional to the change in atmospheric pressure. This has a number of clinically significant consequences when considering pneumothoraces. First, although a person with a pneumothorax being transported by airplane is likely to be in a pressurized cabin, one can expect the barometric pressure to decrease, with a resultant proportional increase in the volume of their pneumothorax if there is not a path of egress for the intrapleural gas such as provided by a chest tube. Second, a clinician whose practice is located at a higher altitude (with lower atmospheric pressure) can expect a slower resolution of pneumothoraces by resorptive diffusion alone than that seen by a colleague practicing closer to sea level.
ETIOLOGY AND PATHOPHYSIOLOGY
Primary spontaneous pneumothorax is the most common cause of pneumothoraces and has an estimated overall incidence of 5 to 10 cases per 100,000 per year. It occurs predominantly in young, healthy men, with an incidence in this group as high as 1 in 500 per year, and is due in most cases to rupture of apical subpleural blebs in otherwise normal lungs (Fig. 12.2). The pathogenesis of these apical blebs is unclear, although it is postulated that higher transpulmonary pressures at the apex lead to greater alveolar distending pressures. The resultant rupture of alveoli traps air between the internal and external elastic membranes of the visceral pleura. It is noted that these types of pneumothoraces most often occur in tall, thin individuals, many of whom are smokers. The lifetime risk of developing a pneumothorax in an otherwise healthy man is estimated at 0.1%, whereas it is as high as 12% in one who is a smoker. There may also be an association with connective
tissue disorders such as Marfan syndrome. A familial form of spontaneous pneumothorax has been described with autosomal-dominant inheritance and incomplete penetrance.
tissue disorders such as Marfan syndrome. A familial form of spontaneous pneumothorax has been described with autosomal-dominant inheritance and incomplete penetrance.
![]() Fig. 12.2. Photomicrograph of apical resection for recurrent primary spontaneous pneumothoraces demonstrating subpleural bleb. |
Spontaneous pneumothorax can also occur as a result of underlying lung disease, in which case it is referred to as a secondary spontaneous pneumothorax. There are various pulmonary disease states leading to secondary pneumothoraces (Table 12.1). Most cases are related to bullous-type disease, with hyperinflation leading ultimately to rupture and subsequent pulmonary parenchymal collapse. Diseases characterized by cystic lesions in the pulmonary parenchyma such as cystic fibrosis and lymphangioleiomyomatosis can also lead to spontaneous rupture and pneumothorax. Malignant
pulmonary nodules, both primary and metastatic, usually located subpleurally, can rupture as they expand and result in a spontaneous pneumothorax.
pulmonary nodules, both primary and metastatic, usually located subpleurally, can rupture as they expand and result in a spontaneous pneumothorax.
Table 12.1 Etiologies of Pneumothorax | |||||||||||||||||||||||||||||||||||||||||||||||||||||||||||||||||||||||||
---|---|---|---|---|---|---|---|---|---|---|---|---|---|---|---|---|---|---|---|---|---|---|---|---|---|---|---|---|---|---|---|---|---|---|---|---|---|---|---|---|---|---|---|---|---|---|---|---|---|---|---|---|---|---|---|---|---|---|---|---|---|---|---|---|---|---|---|---|---|---|---|---|---|
|
Spontaneous pneumothoraces that occur and recur in relation to the menstrual cycle are referred to as catamenial pneumothoraces. These episodes occur within 72 hours before or after the onset of menstruation. Three distinct mechanisms have been proposed based on metastatic, hormonal, and anatomic models. The metastatic model hypothesizes migration of endometrial tissue via the peritoneal cavity through transdiaphragmatic lymphatic channels, via diaphragmatic fenestrations, or hematogenously into the pleural space. An alternative theory suggests that endometrial tissue may be deposited in the chest cavity during embryonal development. Endometrial deposits have been identified in the pleural space in 13% to 62.5% of these cases. Hormonally regulated monthly sloughing of endometrial tissue is believed to result in pleural irritation, causing chest pain, and subpleural breaches, causing air leaks. The hormonal hypothesis suggests that high serum levels of prostaglandin F2 at ovulation leads to vasospasm, associated ischemia with tissue injury, and alveolar rupture. The anatomic model for catamenial pneumothorax is based on the influx of air into the pleural space from the peritoneal cavity via diaphragmatic fenestrations (Fig. 12.3).
Infectious diseases of the lung in their many forms may result in secondary spontaneous pneumothorax. This is most notable in the immunocompromised host such as individuals infected with the human immunodeficiency virus (HIV) and those with manifestations of acquired immunodeficiency syndrome. Pneumothorax occurs in 1% to 2% of hospitalized patients with HIV and is associated with 34% mortality. The most common etiologic agent in these cases is Pneumocystis carinii. Other virulent or opportunistic infections are also causally related to the occurrence of spontaneous pneumothoraces, including most recently severe acute respiratory syndrome (SARS).
Pneumothoraces may also be incited by iatrogenic or traumatic events. Penetration of the pleural space by a needle during a diagnostic or therapeutic procedure, whether deliberate or accidental, may result in a so-called acquired pneumothorax. This is usually due to a breach or laceration of the visceral pleura. This can occur during procedures such as transthoracic needle aspiration, central line placement in the neck, and thoracentesis. In the latter procedure, recent studies have shown that the incidence of iatrogenic pneumothorax can be significantly reduced by using ultrasound guidance.
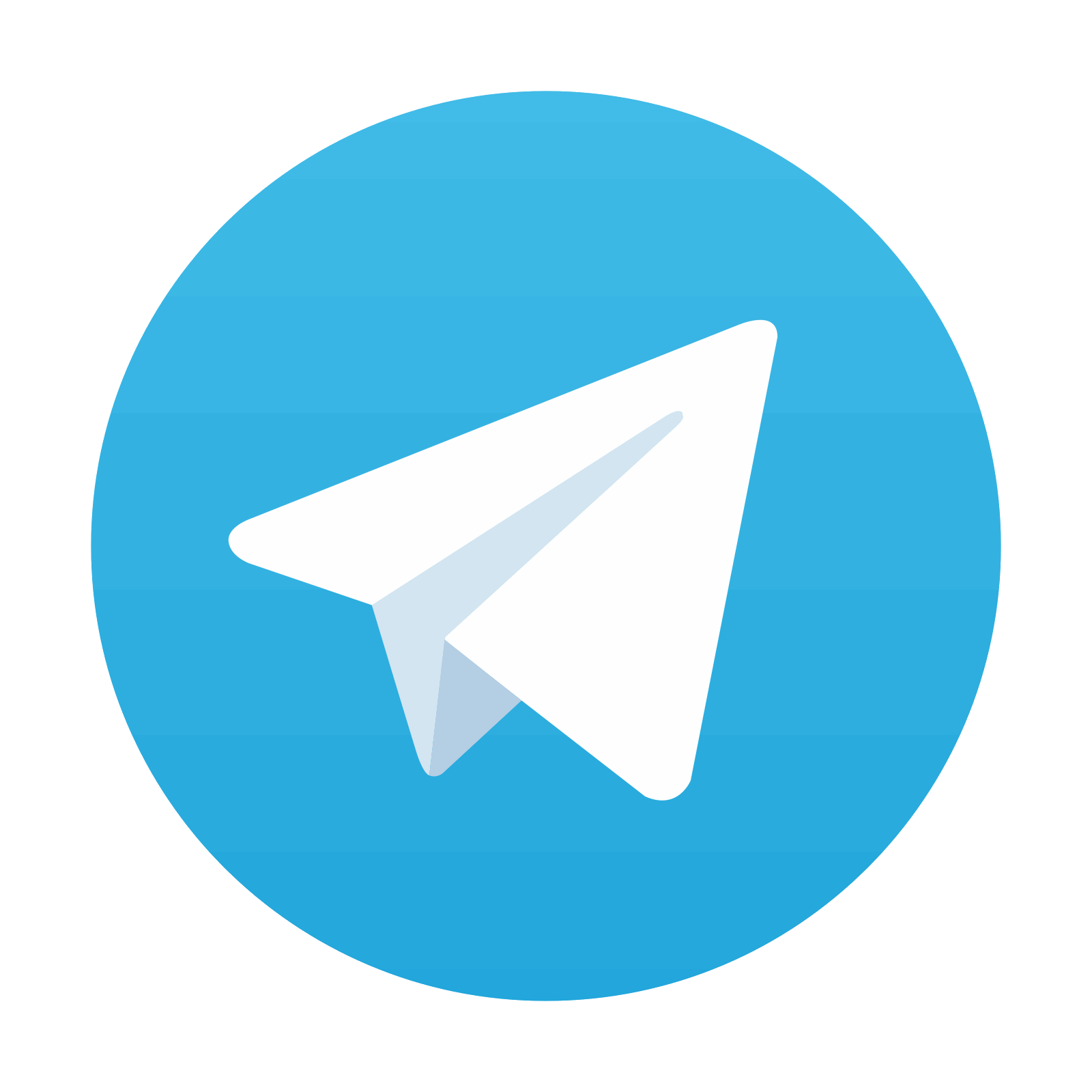
Stay updated, free articles. Join our Telegram channel

Full access? Get Clinical Tree
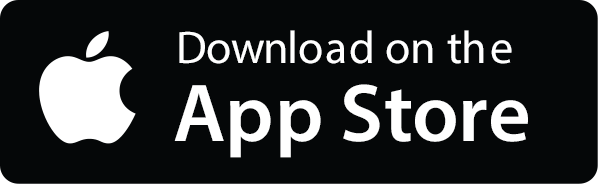
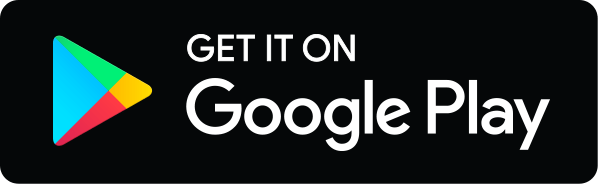