Introduction
Lymphangioleiomyomatosis (LAM) is a rare neoplastic disease characterized by a genetic association with the neurocutaneous syndrome, tuberous sclerosis complex (TSC), marked female gender predominance, cystic destruction of the lung, and progressive respiratory failure. Symptoms and signs include dyspnea on exertion, recurrent pneumothorax, abdominal tumors including renal angiomyolipomas (AMLs) and lymphangiomyomas, and chylous fluid accumulation in the chest and abdomen.
LAM was first reported in a patient with TSC who presented with bilateral spontaneous pneumothorax in 1918 and in a patient without TSC in 1937. In the 1950s through the late 1960s, there were solitary case reports or small case series that fostered a varied and confusing pathologic nomenclature in the literature. Cornog and Enterline, for example, described six cases of “lymphangioleiomyoma” in 1966 and reviewed 14 prior cases in the literature with similar pathology that had been termed “lymphangioma,” “lymphangiomyoma,” “lymphangiopericytoma,” “leiomyomatosis,” “lymphangiomatosis malformation,” and “intrathoracic angiomatous hyperplasia.” Since the late 1970s, larger case studies have better defined the natural history, pathologic classification, and clinical presentation of LAM. Moreover, in the past 2 decades, synergistic interactions between pulmonary investigators, tuberous sclerosis geneticists, Drosophila biologists, and patient advocacy groups have resulted in truly remarkable progress in our understanding of the molecular and cellular basis of LAM, as well as the development of a targeted therapy.
Epidemiology
LAM is seen both in the setting of tuberous sclerosis complex (TSC-LAM) and in a sporadic form in patients who do not have germline mutations in TSC genes or the clinical syndrome of TSC (sporadic LAM, or S-LAM) ( Table 69-1 ). TSC is an autosomal dominant genetic disorder associated with development of hamartomas and dysplastic lesions in several organs, including cortical tubers, giant cell astrocytomas, and subependymal nodules in the central nervous system; angiofibromas, shagreen patches, and ash leaf macules of the skin; and AMLs and cysts of the kidney. The cystic lung changes seen in patients with TSC are typically presumed to be due to LAM but, because the lung is not routinely biopsied in this population, other etiologies are also possible. There have been a number of case reports of biopsy-proven LAM in women with TSC, as well as in a few in men with TSC. In cross-sectional studies from tertiary referral centers, TSC-LAM has been reported in about 10% of men and 30% of women with TSC ; although a recent screening study suggested that age-dependent development of cystic change in women with TSC results in an estimated LAM prevalence of up to 80% by age 40. Symptomatic TSC-LAM is almost entirely restricted to women. In S-LAM, in contrast, lesions of the skin and brain are absent, and manifestations that accompany the pulmonary cystic changes include only renal and extrarenal AMLs and smooth muscle infiltration and obstruction of the lymphatic system. A clinical presentation consistent with S-LAM, including biopsy documentation and negative germ line TSC mutation analysis, has been reported in a single male without clinical evidence of TSC.
TSC-LAM | S-LAM | |
---|---|---|
Estimated no. patients worldwide | 150,000 | 10,000-30,000 |
Reported in males | + | + (one) |
Reported in children | + | + |
Ascertainment | Screening, dyspnea, pneumothorax | Dyspnea, pneumothorax, chylothorax, incidentally on CT |
Germline TSC mutations | + | − |
Inheritable | + | − |
TSC1/TSC2 mutations reported | 33%/66% | 0%/100% |
Renal angiomyolipomas | 93% | 33% |
Bilateral 81% | Bilateral 19% | |
Multiple 60% | Multiple 6% | |
Hepatic angiomyolipomas | 33% | 2% |
Lymphangiomyomas | 9% | 29% |
MMPH | + (~12%) | Very rare, if ever (~1%) |
CNS/skin/eye/cardiac lesions | + | − |
Retroperitoneal, thoracic adenopathy | Rare | + |
Dyspnea | Less common | More common |
Chylothorax | Uncommon | 33% |
Pneumothorax | Less common | 66% |
Respiratory failure | Less common | More common |
Careful epidemiologic analyses of the ethnic and racial distribution of LAM have not been performed. Although the biases introduced by the rarity of the illness, the protean presentation, and the disproportionate reporting of patients with LAM in large series from the United States, Europe, and Asia might suggest a predilection of the disease for whites and Asians, it is likely that all races are affected and that countries and populations with the greatest access to health care resources are overrepresented in these studies.
Harknett and colleagues determined that LAM was diagnosed in 3.4 to 7.8 women per million across seven different countries. The authors did not stratify patients by the presence or absence of TSC, but it is likely that both S-LAM and TSC-LAM populations were included in the estimate. Extrapolation of this prevalence range to the 3.4 billion women on earth results in a predicted global LAM population ranging from 11,600 to 26,500 diagnosed patients.
LAM often presents in a protean manner and it is likely that the disease is severalfold more common than these numbers suggest. Considering that the live birth prevalence of TSC is estimated to be 1/10,000 to 1/12,500 persons and that TSC affects both genders equally, the estimated worldwide prevalence of TSC is approximately 1.1 million to 1.5 million people (assuming a world population of 7 billion people), with roughly half of them being women. If one estimates that cystic pulmonary changes consistent with LAM are found in one third of women with TSC, the global prevalence of TSC-LAM is about 150,000 to 200,000 women, or upwards of 60 TSC-LAM cases per million women in the general population.
Although screening of TSC populations identifies a subset of LAM patients who have a range of cystic change in the lung from very mild to severe, the degree of cystic profusion is often mild or moderate, and pulmonary manifestations appear to reach clinical significance in only about 5% to 10% of patients with TSC-LAM. TSC-LAM is certainly underrepresented in large trials, registries, and databases: patients with TSC-LAM made up only about 14.7% of the 230 patients who enrolled in the National Heart, Lung, and Blood Institute (NHLBI) LAM Registry and 9.5% of patients who are listed in the LAM Foundation database (Sally Lamb, personal communication, The LAM Foundation, Cincinnati). Although TSC-LAM is predicted to be 10-fold more common than S-LAM, most patients with LAM (85% to 90%) who seek medical attention with adult pulmonary specialists have S-LAM. This observation is consistent with the notion that TSC-LAM identified through screening is often a milder disease than S-LAM, which typically reaches attention through progressive dyspnea or pneumothorax/chylothorax. It is also possible that other TSC comorbidities may overshadow the pulmonary manifestations of TSC-LAM and hinder TSC-afflicted patients from seeking medical care for their lung disease.
Genetic and Molecular Basis of Tuberous Sclerosis Complex and Lymphangioleiomyomatosis
Inheritance
Approximately two thirds of new TSC cases arise from de novo mutations, which happen during embryogenesis. Familial TSC, which results from inheritance of germline mutations, accounts for one third of new cases. There have been several reports of familial TSC-LAM, but mother-daughter transmission of S-LAM has never been reported.
Molecular Pathogenesis
Our understanding of the genetic and molecular basis of TSC and LAM has increased substantially since the late 1990s. The most significant findings have included that LAM is a neoplastic and metastatic process, that LAM is caused by mutations in TSC genes, and that dysregulation of the Akt signaling pathway plays a central role in LAM cell growth, motility, and survival.
Tumor Suppressor Proteins Control Cell Growth
Clinicians have long recognized that the cystic lung disease in women with TSC is pathologically indistinguishable from that in women with S-LAM. However, the demonstration that S-LAM and TSC-LAM are genetically linked was reported only relatively recently. The lag period in our understanding may have been due, in part, to the failure to appreciate that a nonfamilial disease could share a common genetic basis with a heritable disease. There are several precedents for this phenomenon among the class of diseases known as tumor suppressor syndromes (including neurofibromatosis and von Hippel-Lindau syndrome), of which TSC is a member.
Tumor suppressor proteins regulate orderly cell growth and differentiation by sensing the surrounding environment, transmitting signals to the nucleus, and directly affecting transcription, translation, survival, or cell division. In the classic “two-hit” paradigm, a mutant copy of a tumor suppressor gene is inherited from one parent and a tumor or dysplastic lesion develops when the second “good” parental copy of the tumor suppressor gene is inactivated through a random, somatic mutation. This first (inherited) hit, often a point mutation or a small insertion or deletion, results in heterozygosity for the allele (i.e., one good copy and one mutant copy). The second mutational event is usually a large deletion, resulting in the loss of a chromosomal segment and loss of heterozygosity (LOH) (two mutant copies). A polymerase chain reaction (PCR) technique can be used to detect LOH for the allele caused by the deletion. When both copies of a tumor suppressor gene contain critical mutations, the protein produced by the gene becomes defective or deficient, protein function is lost, and cell growth, survival, and synthetic function become dysregulated.
The Tuberous Sclerosis Complex Proteins Regulate Signaling through the Akt Pathway
TSC is caused by inactivating mutations in either of the two known TSC loci, TSC1 on chromosome 9q34 or TSC2 on chromosome 16p13. The proteins encoded by TSC1 and TSC2 are hamartin and tuberin, respectively ( Fig. 69-1 ).

Hamartin has no informative homologies with other proteins in the National Center for Biotechnology Information database, whereas tuberin has a domain with GTPase-activating protein (GAP) homology.
Until recently, little was known about the function of TSC proteins other than that they modulate cell growth. In 1999, it was reported that the enlarged eye cell phenotype of the mutant fruit fly, Gigas, was the result of genetic inactivation of the fly homologue for tuberin. The loss of tuberin results in a defect in cell cycle control, which causes cells to repeat S phase without entering M phase. Several laboratories subsequently reported that tuberin and hamartin regulate signaling through the PI3K/PKB(Akt)/S6K pathway, which controls cellular size and proliferation.
Our understanding of the function and positioning of the TSC proteins in the Akt/S6K pathway has been a rapidly evolving process, and the available data to date are modeled in Figure 69-1 . Tuberin and hamartin associate into a complex that functions as a master regulator for the kinase, mTOR, or mammalian target of rapamycin (sirolimus), through an intermediate G protein called Rheb. The intact tuberin/hamartin complex acts as a GAP for Rheb, maintaining the protein in an inactivated, nonphosphorylated state (Rheb-GDP). Phosphorylation of tuberin by Akt inactivates the GAP activity of the tuberin/hamartin complex, increases the abundance of Rheb-GTP, drives association of mTOR with raptor (and mLST8 and PRAS40, not shown) to form the mTORC1 complex, and results in phosphorylation and activation of downstream kinases mTOR, S6K, and the initiation factor 4E-binding protein (4E-BP1). The association of mTOR with raptor (and mSIN1 and mLST8, not shown) to form mTORC2 results in activation of ROCK and rho kinase, cytoskeletal rearrangement, and cell movement.
Genetic mutations that result in the absence or dysfunction of tuberin or hamartin, such as is seen in patients with tuberous sclerosis and LAM, ultimately cause constitutive activation (phosphorylation) of S6 and eIF4E, two proteins that are intimately involved in the regulation of protein translation, as well as Rho kinase, which regulates intracellular trafficking, cell adhesion, and cell movement. The end result is the inappropriate stimulation of protein synthesis, cell motility, and cell growth. Multiple mTOR effectors and mTOR itself were found to be highly phosphorylated in tumors of rats, mice, and humans with mutations in TSC genes. Sirolimus, a microbial product that inhibits mTOR activity, extinguishes phosphoS6 staining and causes widespread apoptosis in the rat renal tumors.
Tuberous Sclerosis Complex Mutations Are Found in the Lung and Kidney Lesions of Patients with Sporadic Lymphangioleiomyomatosis
Tuberous sclerosis patients have germline mutations in TSC genes, but patients with S-LAM do not. Smolarek and coworkers first implicated TSC genes in the pathogenesis of S-LAM by finding that LOH for TSC2 was present in the renal AMLs and lymph nodes of patients with S-LAM. Proof that the loss of tuberin function was responsible for lung disease in LAM was provided by Carsillo and colleagues, who demonstrated the presence of missense and protein truncating TSC2 mutations associated with LOH in the abnormal lung and kidney tissue of patients with S-LAM. Samples taken from the normal regions of lung and the kidney in those patients did not exhibit TSC mutations. These findings were subsequently confirmed in 21 patients by Sato and associates, A single patient with S-LAM caused by TSC1 mutations has been described to date. These data indicate that somatic mutations in either TSC2 or TSC1 alleles after conception cause S-LAM and result in defects or deficiency in tuberin or hamartin. TSC1 and TSC2 mutations have both been described in TSC-LAM, although TSC2 mutations are more common.
Genetic Evidence Suggests That Lymphangioleiomyomatosis Is A Metastatic Neoplasm
Cornog and Enterline were the first to suggest that, despite their benign appearance, cells that make up the LAM lesion represent a clonal, neoplastic proliferation of smooth muscle cells. The demonstration of LOH in the lung, kidney, and lymph node lesions in patients with LAM indicated that Cornog and Enterline’s suspicions regarding neoplasia and clonality were correct. Carsillo and colleagues further demonstrated that the mutations present in the cells of the kidney tumor and lung lesion of a given individual with S-LAM were identical, suggesting that they arise from a common precursor.
A model for LAM has been proposed in which the lung is infiltrated as a consequence of benign metastasis of LAM cells from a remote and, as yet unidentified, source such as the bone marrow, the lymphatic system, the uterus, or AMLs. Reports of recurrence of LAM in the donor lung of patients with LAM who had undergone lung transplantation are also consistent with this metastatic theory ; in two cases, the source of lung metastases were proven to arise from the recipient by genetic and molecular techniques. These data contradicted earlier reports that the recurrent lesions were derived from donor cells, but in retrospect, the initial studies were limited by the spatial resolution that can be achieved with the immunohistochemical techniques used to colocalize cellular markers. Cells containing LOH for TSC genes have been isolated from the blood of patients with LAM, consistent with dissemination through the systemic circulation.
Other rare diseases that result from metastases of benign smooth muscle cells in women include leiomyomatosis peritonealis disseminata, intravenous leiomyomatosis, and benign metastasizing leiomyomatosis. The metastatic theory of LAM suggests possible novel approaches for treatment based on preemptive removal of the source, once its identity is known.
Role of Lymphangiogenesis and Lymphatic Spread In Dissemination of Lymphangioleiomyomatosis
Approximately 30% of patients with LAM have axial abdominal or thoracic lymphadenopathy. In some cases, LAM is restricted to the retroperitoneum, abdomen, or pelvis and is associated with normal lung parenchyma or only a very few lung cysts, consistent with regional spread from a subdiaphragmatic source. Clusters of cells in the chylous pleural fluid of patients with LAM were first described by Valensi in 1973. Later, Itami and coworkers demonstrated that the clusters originated in the dilated lymphatic system and were composed of alpha smooth muscle actin–positive spindle cells enveloped by a single layer of endothelial cells. They suggested that LAM cell clusters could be used diagnostically to obviate the need for biopsy in patients with chylous manifestations of LAM. Data from Japan provide additional evidence that a likely source and mechanism of spread of LAM may be through the lymphatic circulation. In a small autopsy series, Kumasaka and colleagues described the presence of LAM cell clusters enveloped by lymphatic endothelial cells budding from the walls of lymphatic vessels and in the lumen of lymphatic channels and the thoracic duct.
Induction of lymphangiogenesis appears to play an important role in this process, based on abundant expression of lymphatic endothelial markers such as podoplanin (D2-40), LYVE-1, vascular endothelial growth factor (VEGF) receptor 3 (VEGFR-3), VEGF-C, and VEGF-D. Several laboratories have reported that VEGF-D is elevated more than threefold to eightfold in the serum of patients with LAM, a finding that is useful for distinguishing LAM from other cystic lung diseases and for predicting response to therapy. LAM clusters are found in the lymphatic lumen, in regions between axial lymph nodes (retroperitoneal, mediastinal, and left venous angle) that are infiltrated with LAM cells, and in chylous fluids of patients with chylous ascites and chylothorax. LAM cell clusters enter the venous circulation at the insertion of the thoracic duct into the junction of the left internal jugular and subclavian veins and disseminate throughout the pulmonary capillary bed. LAM cell clusters impacted in the lung vasculature proliferate and invade, perhaps through a novel mechanism called “invasion-independent metastasis.”
Role of Estrogen
Estrogen-containing drugs have been implicated in the worsening of LAM. Estrogen may play a role in LAM cell migration, infiltration, proliferation, or secretion of destructive proteases. Estrogen regulates the transcription of many genes, and there is some evidence that estrogen can modulate signaling through the Akt pathway. Estrogen-mediated activation of the ERK pathway promotes activation of the late response gene FRA1 , which is associated with epithelial mesenchymal transition. Akt activation, in turn, enhances the efficiency of FRA1 translation through the phosphorylation of the S6K1-dependent eucaryotic translation factor, 4b. Estrogen was shown to promote pulmonary metastases and enhanced survival of TSC2-deficient cells in mice. Collectively, these data suggest that targeting the E2-ERK pathway in combination with the mTORC1 pathway may be an effective combination therapy strategy for LAM.
Mechanisms of Matrix Remodeling in Lymphangioleiomyomatosis
Cystic destruction of the lung parenchyma causes progressive decline in lung function in patients with LAM, and proteases are believed to play a major role in the process.
In addition, multiple growth factors are abundant in LAM, which induce proliferation, suppress apoptosis and support fibrosis. Targeting the systems that promote proteolytic and fibroproliferative responses are possible therapeutic strategies for the future.
The spectrum of metalloproteinases that are aberrantly expressed in LAM has not been fully characterized, but matrix metalloproteinase (MMP)-2 is the most abundant of those studied to date. Overexpression of MMP-1, MMP-9, MT1-MMP, and MMP-14 has also been reported, and serum levels of MMP-9 are elevated in patients with LAM. MMP-1, MMP-2, and MMP-9 are able to degrade elastin, consistent with the loss and degradation of elastic fibers seen in LAM. Zhe and associates have proposed that increased expression of serum response factor may lead to MMP activation and down-regulation of tissue inhibitor of metalloproteinase (TIMP-3) in LAM cells, leading to an imbalance of proteases and protease inhibitors. Abundant expression of cathepsin K has been reported in LAM, suggesting a possible pathogenic role of this protein in tissue destruction.
Basic fibroblast growth factor (bFGF) is a potent mitogenic factor for smooth muscle cells, myofibroblasts, and fibroblasts. LAM lesions have also been reported to contain abundant tryptase-positive mast cells, which express bFGF and receptors Bek (FGFR-2) and Flg (FGFR-1), as well as chymases, renin, angiotensin-converting enzyme, and angiotensin receptors type I and type II. Insulin-like growth factors (IGFs) are involved in normal pulmonary development and in the pathogenesis of smooth muscle cell proliferation. IGF-I, IGF-II, IGF-I receptor, IGF-binding protein (IGFBP)-1, IGFBP-2, IGFBP-4, IGFBP-5, and IGFBP-6 are expressed in lung tissue of patients with LAM. Carelli and coworkers showed that TSC2 −/− human smooth muscle cells derived from AMLs express the apoptosis inhibitor protein survivin when exposed to IGF-1. Transforming growth factor-β1, a profibrotic cytokine, is enriched in highly cellular areas of the LAM lesion.
Clinical Features
LAM usually presents during the reproductive years, with a mean age at the onset of symptoms in the early to mid-30s. Although new diagnoses of LAM have been reported in patients as young as 12 years, documented cases of the disease before menarche are rare. A number of reports in the literature describe women in whom the diagnosis was made after menopause and in the ninth decade of life. LAM does not appear to be smoking-related, because the majority of patients in the largest case series were nonsmokers.
Because LAM is rare and the symptoms are often nonspecific, the diagnosis is often delayed, with a mean interval between the onset of symptoms and diagnosis of 5 to 6 years. Initial incorrect diagnoses in these series typically included bronchial asthma, emphysema, chronic bronchitis, chronic obstructive pulmonary disease, sarcoidosis, diffuse panbronchiolitis (in Asia), and even idiopathic interstitial pneumonias including idiopathic pulmonary fibrosis.
In the patients reported by Urban and associates, exertional dyspnea and spontaneous pneumothorax were the initial symptoms in 49% and 46% of patients, respectively. During the course of the illness, dyspnea developed in 87% of patients, and pneumothorax developed in 68% of patients. The first pneumothorax precedes the diagnosis of LAM in 82% of patients; in fact, most patients have two pneumothoraces before the diagnosis is made. In patients with LAM who have a pneumothorax, the likelihood of an ipsilateral or contralateral recurrence is greater than 70%. Pneumothorax most likely results from the rupture of subpleural blebs, but whether the event is triggered by progressive degradation of connective tissue matrix or airflow obstruction and overdistention of distal air spaces, or both, is unclear.
Other signs and symptoms of LAM, which may be present at the onset or may develop during the course of the disease, include fatigue, cough, hemoptysis (rarely massive), chest pain, and symptoms related to reflux of chylous fluid into pleural (33% of patients) and extrapleural locations, such as the peritoneum (chylous ascites) or pericardium (chylopericardium), airway (chyloptysis), and genitourinary tract (chyluria and chylous metrorrhea). Fistulous communication in the gut can result in protein-losing enteropathy. Chylous pulmonary parenchymal congestion was recently described in patients with LAM and should be considered in the differential of subacute worsening of oxygenation or shortness of breath. It is likely that the chylous complications of LAM result from obstruction of lymphatic channels due to infiltration by smooth muscle cells.
AMLs, unusual hamartomas composed of fat, smooth muscle, and abnormal blood vessels, may arise in virtually any location in the chest and abdomen but are most common in the kidney ( eFig. 69-1 ). About 29% to 33% of patients with S-LAM have renal AMLs, compared with about 88% to 93% of patients with TSC or TSC-LAM. AMLs are more commonly unilateral and solitary in patients with S-LAM, and more commonly bilateral and multiple in patients with TSC-LAM (see eFig. 69-1 ). Blood vessels in AMLs are often tortuous and aneurysmal and are composed of cells with normal genotype and cells with TSC mutations. Spontaneous hemorrhage into AMLs may produce severe flank or abdominal pain, acute hypotension, and/or anemia, occasionally in association with circulatory collapse. Renal cysts are seen occasionally in patients with LAM; in TSC-LAM, polycystic kidney disease may develop due to a contiguous gene deletion of PKD1 which is immediately adjacent to TSC2 on chromosome 16.
Lymphangiomyomas are tumor-like masses characterized by smooth muscle (LAM cells) and endothelial proliferation of lymphatic vessels and lymph nodes in the mediastinum, retroperitoneum, and lung. Not uncommonly, LAM presents as retroperitoneal masses, including cystic lymphangiomyomas ( eFig. 69-2 ) or lymph nodes with hypodense centers, which mimic necrotizing lymphomas, ovarian or renal cancers, or other malignancies.
There have been case reports of exacerbation of LAM associated with birth control pill use or during pregnancy. In a group of 69 patients with sporadic LAM, pulmonary symptoms began during pregnancy in 9 of the 46 patients (20%) with a history of pregnancy before or at the time of diagnosis. In this same group, a marked exacerbation of previously diagnosed pulmonary LAM was observed in two (14%) patients during pregnancy. The effect of pregnancy or exogenous estrogen use on LAM has not been systematically studied.
Physical Examination
The physical examination in LAM is often nonspecific. Lung auscultation is usually uninformative but may reveal rhonchi or wheezing in some patients. Crackles are unusual and should raise suspicion for chylous pulmonary congestion. Clubbing is not a feature of LAM, despite being reported in 3% and 5% of patients in two larger case series. Physical examination may also reveal evidence of pleural effusion, ascites, or pneumothorax, if present. Careful dermatologic, ocular, and dental surveys should be performed for evidence of TSC, including facial angiofibromas, subungual fibromas, palpable dysplastic cutaneous lesions called shagreen patches, hypomelanotic macules including those with ash leaf or confetti configurations, retinal hamartomas, and dental pitting.
Imaging Studies
The chest radiograph in patients may appear relatively normal ( eFig. 69-3 ), even late in the disease, or may suggest hyperinflation only. As the disease progresses, the chest radiograph often demonstrates diffuse, bilateral, and symmetric reticulonodular opacities ( eFig. 69-4 ), cysts ( eFig. 69-5 ), bullae, or a “honeycomb” or somewhat “pseudofibrotic” appearance ( eFig. 69-6 ). Pleural effusion and pneumothorax ( eFig. 69-7 ) may also be apparent ( Fig. 69-2 ). Preservation of lung volumes in the presence of increased interstitial markings is a radiographic hallmark of LAM that helps distinguish it from most other interstitial lung diseases (see eFigs. 69-3A, 69-4, 69-5A, and 69-6A ), in which alveolar septal and interstitial expansion tend to increase the lung’s elastic recoil properties and decrease lung volumes.

The high-resolution computed tomography (HRCT) chest scan is much more sensitive than the chest radiograph in detecting cystic parenchymal disease and is almost always abnormal at the time of diagnosis, even when the chest radiograph and pulmonary function assessments are normal. The typical CT shows diffuse round, bilateral, thin-walled cysts of varying sizes ranging from 1 to 45 mm in diameter (see Fig. 69-2 ; see eFigs. 69-1C, 69-2C, 69-3B through D, 69-5B, 69-6B, and 69-7B ). The numbers of cysts varies in LAM from a few (see eFig. 69-7B ) to almost complete replacement of the normal lung tissue (see eFig. 69-5B ). The profusion of cysts tends to be milder in patients with TSC-LAM than S-LAM, perhaps explained in part by ascertainment of patients with TSC-LAM earlier in the disease process by screening.
The morphology of the cysts is useful in differentiating LAM from other cystic lung diseases ( Fig. 69-3 ). In emphysema , dilated airspaces often have internal structure, such as septae or a “centrilobular dot” consistent with a vessel, features virtually never seen in LAM. In addition, in emphysema, the borders of the lucent spaces are difficult to discern, whereas in LAM, cyst walls are thin but easily perceptible. Langerhans cell histiocytosis cysts (see eFig. 54-37 ) have thick walls and the cystic spaces are bizarre in shape. Birt-Hogg-Dubé (BHD) cysts tend to predominate in the lower lobes, while LAM cysts are distributed diffusely. Lymphocytic interstitial pneumonia cysts can closely resemble those of LAM, but are often larger, more varied in size, and bounded by eccentric vessels. Blinded expert radiologists can correctly identify LAM among a pool of CT scans consisting of a variety of other cystic lung diseases about 72% of the time. Pleural effusions are seen on CT in 12% of patients with S-LAM and 6% of patients with TSC-LAM. Other CT features include linear densities (29%), hilar or mediastinal lymphadenopathy (9%), pneumothorax, lymphangiomyomata (see eFig. 69-2 ), and thoracic duct dilation. Ground-glass opacities (12%) suggest the presence of interstitial edema due to lymphatic congestion ( Fig. 69-4 ).






In patients with TSC, nodular densities on HRCT may represent multifocal micronodular pneumocyte hyperplasia (MMPH) made up of clusters of hyperplastic type II pneumocytes ( Fig. 69-5 ; see eFig. 56-2 ). MMPH may be present in males or females with TSC in the presence or absence of LAM, but not in patients with S-LAM. MMPH is not typically associated with physiologic or prognostic consequences, but one case of respiratory failure due to MMPH has been reported.

Chu and associates reported that ventilation-perfusion scans were abnormal in 34 of 35 women with LAM. The most common abnormality was nonspecific diffuse heterogeneity, usually grossly matched. These authors also described an “unusual,” “speckling pattern” on the perfusion images in 74% of patients, consisting of “small, often peripheral collections of radioisotope.”
LAM and AML lesions do not typically exhibit increased uptake of 18 F-fluorodeoxyglucose on PET scanning. Other neoplasms (or sources of inflammation) should therefore be considered in known or suspected LAM cases in which FDG-PET results are positive.
Abnormalities on abdominal imaging, such as renal AML (see eFig. 69-1A and B ) and enlarged lymphatic structures, are also common in LAM ( Figs. 69-6 and 69-7 ). Fat density within a renal mass is pathognomonic of AMLs (see eFig. 69-1A and B ). AMLs are more prevalent and more frequently bilateral and large in patients with TSC-LAM than in patients with S-LAM, and AML size correlates with the prevalence of pulmonary cysts in patients with TSC. Avila and associates reported the results of CT imaging in 256 patients with S-LAM and 67 patients with TSC-LAM who were referred to the National Institutes of Health. Renal AMLs were present in 32% of patients with S-LAM and 93% of patients with TSC-LAM. Hepatic AMLs (see eFig. 69-1A ) were present in 2% of patients with S-LAM and 33% of patients with TSC-LAM. Ascites was uncommon, seen in fewer than 10% of patients with LAM. Abdominal lymphangiomyomas (see eFig. 69-2B ), often containing both cystic and solid components, were seen in 29% of patients with S-LAM and 9% of patients with TSC-LAM.


Central nervous system abnormalities, such as cortical or subependymal tubers and astrocytomas, are common in patients with TSC, including those with TSC-LAM, but are not found in women with S-LAM. Moss and associates reported that women with S-LAM and TSC-LAM may have an increased incidence of meningioma, but the significance of that finding has been challenged.
Pulmonary Function Testing
Pulmonary function testing in patients with LAM may be normal or may reveal obstructive, restrictive, or mixed patterns, with obstructive physiology being the most common abnormality. Quality controlled lung function data were collected prospectively by the NHLBI Registry, a 5-year study of patients with LAM in centers around the United States. Spirometry revealed obstructive changes in about 57% of patients and normal results in 34%. Restriction, defined as a total lung capacity less than the lower limit of normal, was seen in 11%. Hyperinflation was unusual, present in about 6%. The average residual volume was 125% of predicted when measured by plethysmography, but was only 103% of predicted determined with gas dilution methods, suggesting significant air trapping in noncommunicating airspaces. Approximately 25% of patients with obstructive physiology may demonstrate bronchodilator responsiveness. The obstructive physiologic defect in LAM is primarily attributable to airflow obstruction and, to a lesser degree, to a modest increase in lung compliance.
The most common finding on initial pulmonary function testing in various case series was abnormal gas transfer, as assessed by the diffusing capacity for carbon monoxide (D l co ), described in 82% to 97% of patients. It is not unusual for D l co to be reduced out of proportion to forced expiratory volume in 1 second (FEV 1 ). Reduction in D l co and increase in residual volume are generally considered to be the earliest physiologic manifestations of LAM.
Crausman and colleagues studied the mechanism of exercise limitation in 16 patients with LAM. They concluded that poor exercise performance was primarily due to airflow obstruction and increased dead-space ventilation caused by pulmonary vascular disease or extensive cystic change. Cardiopulmonary exercise testing in a much larger cohort of patients with LAM revealed a reduced maximal oxygen consumption ( ) and anaerobic threshold in 217 patients. Exercise-induced hypoxemia was found even in patients who did not have resting abnormalities in FEV 1 and D l co . In most patients, exercise was thought to be ventilation limited, owing to airflow obstruction and increased dead-space ventilation.
Disease progression is usually accompanied by a progressive obstructive ventilatory defect, and decline in FEV 1 is the most commonly used parameter to monitor disease progression. Although resting pulmonary hypertension appears to be unusual in LAM, pulmonary arterial pressure often rises with low levels of exercise, related in part to hypoxemia. Zafar and colleagues reported an increase in intraparenchymal shunts in dyspneic patients with LAM, which may contribute to resting and exercise hypoxemia.
Taveira-DaSilva and coworkers correlated lung function testing with lung histology in 74 patients. They reported that a positive bronchodilator response was associated with a predominantly solid pattern of lung lesions as opposed to a cystic pattern. They also found that the D l co correlated with the lung histology score, a quantification of the extent of involvement with cystic lesions and LAM cells. FEV 1 and D l co were the best predictors of .
Pathology
Grossly, LAM lungs are enlarged and diffusely cystic, with dilated air spaces as large as several centimeters in diameter ( Fig. 69-8 ). Microscopic examination of the lung reveals foci of smooth muscle cell infiltration of the lung parenchyma, airways, lymphatics, and blood vessels associated with areas of thin-walled cystic change. LAM lesions often contain an abundance of lymphatic channels, forming an anastomosing meshwork of slitlike spaces lined by endothelial cells. LAM cells generally expand interstitial spaces without violating tissue planes but have been observed to invade the airways, the pulmonary artery, the diaphragm, aorta, and retroperitoneal fat, to destroy bronchial cartilage and arteriolar walls, and to occlude the lumen of pulmonary arterioles.

There are two major cell morphologies in the LAM lesion: small spindle-shaped cells and cuboidal epithelioid cells. LAM cells stain positively for smooth muscle actin, vimentin, desmin, and, often, estrogen and progesterone receptors ( Fig. 69-9 ). The cuboidal cells within LAM lesions also react with a monoclonal antibody called HMB-45, developed against the premelanosomal protein gp100, an enzyme in the melanogenesis pathway. This immunohistochemical marker is very useful diagnostically, because other smooth muscle–predominant lesions in the lung do not react with the antibody. The spindle-shaped cells of the LAM lesion are more frequently proliferating cell nuclear antigen-positive than the cuboidal cells, consistent with a proliferative phenotype. Compared with cigar-shaped normal smooth muscle cells, spindle-shaped LAM cells contain less abundant cytoplasm and are less eosinophilic. Estrogen and progesterone receptors are also present in LAM lesions, but not in adjacent normal lung tissue. LAM lesions express lymphatic markers LYVE-1, PROX1, podoplanin and VEGFR-3. The smooth muscle–like cells of AMLs are morphologically and immunohistochemically similar to LAM cells, including reactivity with antibodies directed against actin, desmin, vimentin, and HMB-45 as well as estrogen and progesterone receptors. Unlike the dilated air spaces in emphysema, the cystic spaces found in LAM may be partially lined with hyperplastic type II cells.

Diagnosis
The diagnosis of LAM usually follows CT scanning of the chest, often triggered by one of four presentations: chronic dyspnea on exertion, new onset or recurrent pneumothoraces, chylous effusion, or incidental cysts found on scans of the chest, heart, or abdomen done for other reasons. When based on dyspnea, diagnoses such as obstructive pulmonary diseases (e.g., asthma or chronic bronchitis) are often erroneously made first, resulting in delays in the diagnosis of LAM for up to several years. The time to diagnosis is typically shorter following pneumothorax or chylous effusion, but most patients have more than one pneumothorax before the diagnosis is made. Cardiac and abdominal CT scans performed for other indications can detect cystic change in the lung and lead to performance of a dedicated chest CT and a diagnosis of LAM.
Clinical Diagnosis
In the proper clinical context, a confident, clinical diagnosis of LAM may be established based on radiographic findings. For instance, classic LAM cystic changes on HRCT of the lung along with a chylothorax documented by thoracentesis or CT evidence of fat-containing renal masses consistent with AMLs establishes the diagnosis with a high degree of certainty. There are rare exceptions, however, because lymphomas can present with pulmonary cystic change and chylothorax, and Birt-Hogg-Dubé (BHD) syndrome (see Fig. 69-3 ) can present with pulmonary cystic change, and AMLs, albeit rarely. Pulmonary cystic change in a nonsmoking woman with TSC is consistent with LAM and does not usually require biopsy. In smoking patients with or without TSC, the diagnoses of pulmonary emphysema and Langerhans cell histiocytosis (see Fig. 69-3 ; see eFig. 54-37 ) must be seriously considered. Serologies for Sjögren syndrome antibody A (SS-A) and B (SS-B) should be obtained to investigate follicular bronchiolitis and lymphocytic interstitial pneumonitis; alpha 1 antitrypsin should be measured to exclude emphysema caused by antitrypsin deficiency. Serum VEGF-D is elevated threefold to eightfold in patients with LAM, but not in patients with pulmonary emphysema, Langerhans cell histiocytosis, lymphangiomatosis, or BHD and is useful in differentiating LAM from these disorders. A serum level of VEGF-D of at least 800 pg/mL in a patient with typical cystic change on HRCT establishes the diagnosis of LAM with a sensitivity and specificity of 60% and 100%, respectively.
To aid in diagnosis of LAM, a dedicated CT of the abdomen may be obtained to screen for AMLs, lymphangiomyomas, uterine masses, and chylous ascites. Renal AMLs can be identified radiographically based on the presence of fat (see eFigs. 69-1A and B ); biopsy may be required to rule out renal cell carcinoma in the rare cases in which renal masses are solid appearing and do not contain fat.
Differential Diagnosis
The primary differential diagnoses in patients with a smoking history include Langerhans cell histiocytosis and pulmonary emphysema. The morphology of the cysts can be helpful in differentiating these disorders from LAM; in emphysema (see Fig. 69-3 ), the cysts are devoid of distinct walls and, in Langerhans cell histiocytosis (see Fig. 69-3 ; see eFig. 54-37 ), the cysts are thicker walled, mid and upper lung zone predominant, and more irregularly shaped. Diffuse subcentimeter nodular changes are often present in Langerhans cell histiocytosis (see eFig. 54-38 ), but can also be seen in TSC-LAM, where they usually represent MMPH. Other cystic lung diseases that can mimic LAM and that should also be considered include Sjögren syndrome, Castleman disease, follicular bronchiolitis and lymphocytic interstitial pneumonitis (see Fig. 69-3 ), Pneumocystis jirovecii pneumonia (see eFigs. 90-14 and 90-15 ), recurrent respiratory papillomatosis (see eFig. 54-29 , eFig. 54-30 , eFig. 54-31 , eFig. 54-32 ), hyper IgE syndrome, hypersensitivity pneumonitis ( eFig. 69-8 ), amyloidosis (see eFig. 54-35 ), lymphoma, light chain deposition disease, and bronchopulmonary dysplasia/barotrauma. Note that a few scattered thin-walled cysts may normally be encountered on HRCT studies, particularly in older individuals. Recently, diffuse thin-walled cysts seen on HRCT have been described in a small number of patients with various small airway obstructive disorders, including asthma. Thin-walled cystic lung lesions can also be associated with metastatic genitourinary neoplasms ( eFig. 69-9 ) including endometrial stromal cell sarcoma, low-grade leiomyosarcomas and angiosarcomas ( eFig. 69-10 ), cystic fibrohistiocytic tumor, and cystic benign metastasizing leiomyoma ( eFig. 69-11 ).
BHD syndrome can be difficult to distinguish from LAM. BHD is a rare tumor suppressor syndrome associated with spontaneous pneumothorax, skin lesions, bland, peripheral and subpleural pulmonary cysts devoid of smooth muscle infiltration (see Fig. 69-3 ; Fig. 69-10 ), and inherited renal neoplasms. Mutations in the folliculin gene ( FLCN ) cause defects or deficiency in a protein of unknown function called folliculin and result in BHD syndromes of renal tumors, lung cysts, and skin lesions, or familial spontaneous pneumothorax. It appears that BHD is also associated with aberrant signaling through the Akt pathway, but the loss of regulation is upstream of mTOR.

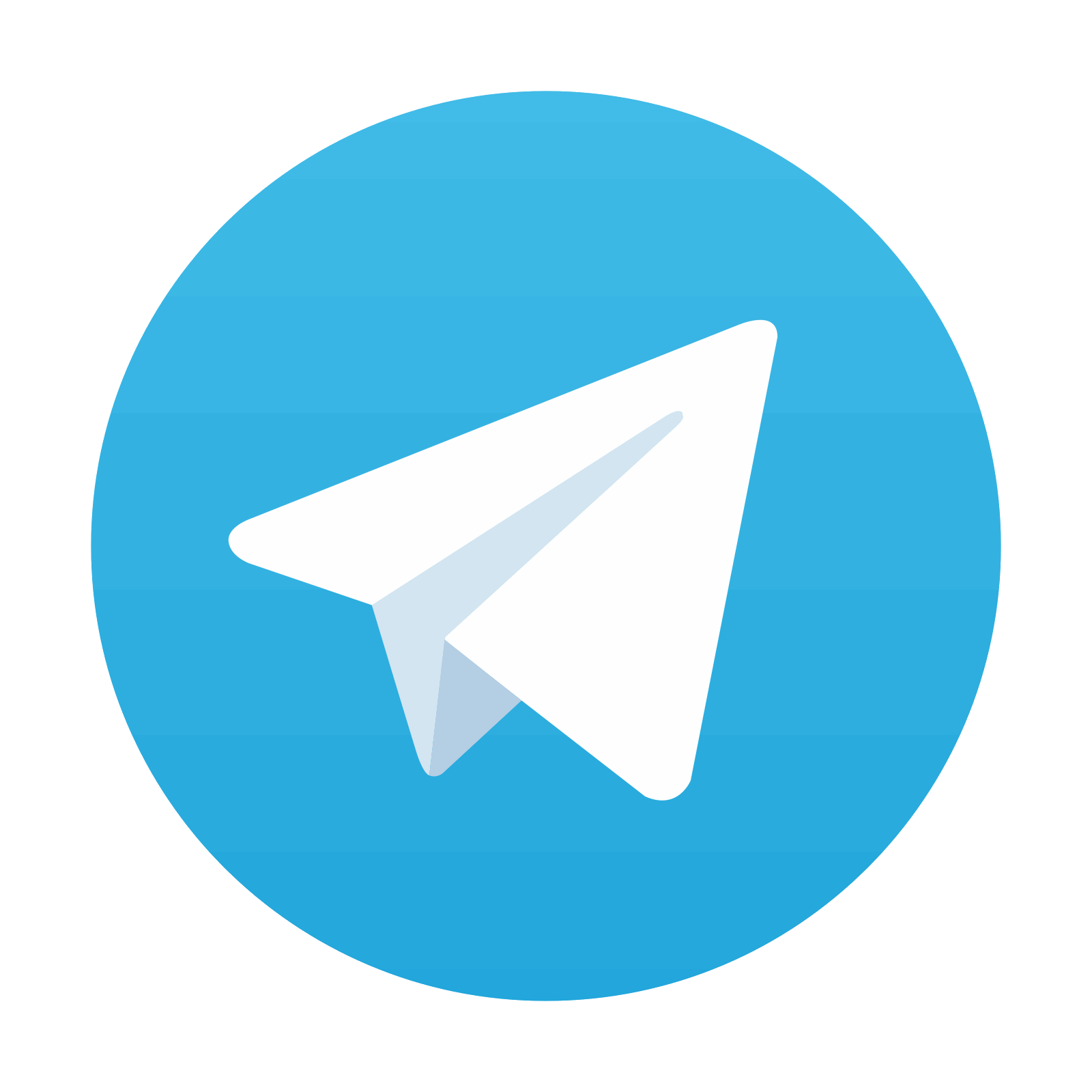
Stay updated, free articles. Join our Telegram channel

Full access? Get Clinical Tree
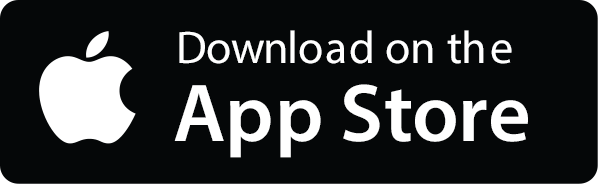
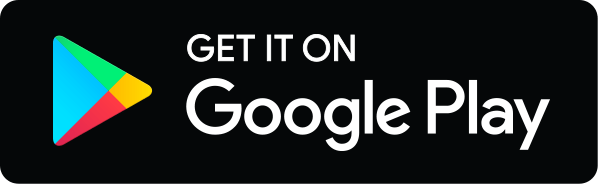
