Lung Transplantation
The last 30 years have seen the development and promulgation of lung transplantation as a robust therapy for advanced lung diseases for which no other medical therapy is effective. At best lung transplantation is immediately lifesaving and capable of offering selected recipients extended and good quality survival. However, there are many problems that beset the graft and may lead to chronic lung allograft dysfunction (CLAD). Principal among these is chronic rejection (CR), manifest as fibrotic luminal occlusion of small airways (bronchiolitis obliterans) for which the term bronchiolitis obliterans syndrome (BOS) was coined to allow a uniformity of description and grading of severity throughout the world.1,2 As the field of lung transplantation has matured, a community of dedicated lung transplant clinicians and scientists has formed, working together to develop a number of position papers, guidelines and clinical trials to guide practice. Lung transplantation has expanded its reach in dramatic fashion, with the edge of the diaspora that commenced in the modern era at Stanford University Medical Center touching most nations and many conditions once thought unsuitable for transplantation. It has been used as a successful therapeutic intervention for a wide variety of end-stage pulmonary parenchymal and vascular diseases. There is no doubt that advances in recipient and donor selection, surgical technique, and postoperative management have improved early survival as documented in serial iterations of the annual registry of the International Society for Heart and Lung Transplantation (ISHLT); late survival is slowly improving as well.4
Novel areas of interest in the lung transplantation world include the use of so-called marginal donors to assist in overcoming the relative shortage of donor organs and the development of donation after circulatory death protocols that hold great promise to provide outcomes at least equivalent if not better than donation after brain death.5 Ex vivo lung perfusion may facilitate organ salvage and promote optimal outcomes.6,7 So may our increasing understanding of antibody-mediated rejection (AMR), another area where knowledge is changing rapidly, generate superior outcomes in the future.8,9
HISTORY
Pioneering efforts in experimental lung transplantation were undertaken in the 1940s and 1950s. In Russia in 1946, Demikhov, working without the benefit of cardiopulmonary bypass, performed a variety of experiments involving transplantation of pulmonary lobes and heart–lung blocs in dogs.10 These experiments demonstrated the technical feasibility of such procedures but warned of the physiologic challenges of the control of breathing reflexes. Dogs have a well-developed Hering–Breuer inflation inhibition reflex, so they fail to breathe adequately after reimplantation of the heart–lung bloc. Demikhov’s first heart–lung transplant survived 2 hours and only 2 of the 67 transplants he performed over the next 5 years survived more than 5 days. Nakae subsequently demonstrated the fallacy of assuming the homogeneity of pulmonary neural control between species and showed how this reflex was developed differentially between species in an elegant series of experiments in cats, dogs, and monkeys.11 This opened the way for further work on subhuman primates by Shumway and Reitz, who were able to demonstrate extended survival after heart–lung transplantation.12 Subhuman primates were able to override the Hering–Breuer reflex and breathe so the hope of human lung transplantation was rekindled. This physiologic quirk excited a number of the early researchers who studied the physiology of the transplanted lung devoid of neural control mechanisms, cough reflexes, antegrade bronchial blood supply, and normal mucociliary clearance mechanisms.13–20 In addition, an early observation was that in heart–lung transplantation, the heart and the lung individually pursued different functional courses, foreshadowing the differing rates of rejection of the heart and lungs that were seen in human combined heart–lung transplantation performed more than three decades later.21
Human lung transplantation was undertaken first by Hardy22,23 in 1963. The procedure consisted of a left single-lung transplant performed for a carcinoma of the left lung that involved the hilum. The patient survived for 18 days, dying of renal failure and malnutrition. This effort demonstrated that a transplanted lung could function for the short term in a patient and stimulated further clinical and experimental efforts. Between 1963 and 1978, however, at least 38 attempts were made at isolated lung transplantation and only 1 patient survived to hospital discharge, succumbing to sepsis and CR 8 months after transplantation. The remaining patients in this 15-year experience all died postoperatively. The major cause of mortality beyond the first postoperative week in these patients was bronchial dehiscence. In addition, most of the patients were greatly debilitated at the time of the procedure, frequently ventilator dependent or in a state of multisystem and multiorgan failure, hindering their ability to survive. It was appreciated that in many of these patients, the available immunosuppressive regimens, which relied on high-dose corticosteroids, significantly compromised postoperative healing of the bronchus and further potentiated the adverse effects of pre-existing conditions.
The problem of bronchial healing was related to the relative ischemia of the donor bronchus, which followed revascularization of the lung graft without reestablishment of the bronchial circulation. One technical approach to this problem was the development of a procedure for combined heart–lung transplantation, allowing for maintenance of collateral circulation from the coronary circulation to the tracheal anastomosis and mediastinal tissues as well as retrograde perfusion from the pulmonary arterial circulation. Although the operation was performed primarily for patients with end-stage cardiac failure due to pulmonary hypertension, the initial report of successful heart–lung transplantation demonstrated the feasibility of this approach in obtaining healing of the airway and confirmed the ability of the transplanted lung to provide long-term respiratory function. The world’s first recipient of a combined heart–lung transplant died 5 years post transplant from trauma. Postmortem examination revealed no complications of rejection or infection in the transplanted heart or lungs. Subsequently, heart–lung transplantation has been performed for numerous pulmonary parenchymal diseases, including emphysema and bilateral septic lung disease, such as cystic fibrosis, but is now only performed for conditions that cannot be serviced by single- or bilateral-lung transplantation such as some forms of congenital heart disease.
An alternative technique for improving bronchial healing was to optimize the bronchial–pulmonary collateral circulation by limiting the length of the donor bronchus and by providing an alternative vascular supply by extrinsically wrapping the anastomosis with omentum. In addition to these technical measures, the avoidance of high-dose steroid immunosuppressive regimens, made possible by the use of cyclosporine, was shown to improve bronchial anastomotic healing. These advances, combined with the selection of well-conditioned recipients with pulmonary fibrosis, whose pathophysiology favored preferential perfusion and ventilation of the allograft, culminated in the initial clinical success of single-lung transplantation. Further efforts were made to perfect a technique for double-lung transplantation to expand this approach to patients with bilateral septic lung disease. The initial clinical success of an en bloc double-lung transplant procedure was tempered by a significant incidence of tracheal anastomotic complications. However, further modification of the technique, by either direct bronchial revascularization or performance of two sequential single-lung transplantations at the same operative sitting (i.e., bilateral sequential single-lung transplantation), has provided satisfactory results.
It has since been shown that despite initial concerns about the physiology of allograft ventilation, single-lung transplantation is also appropriate for patients with end-stage chronic obstructive pulmonary disease (COPD). Single- and bilateral-lung transplantation have also been successfully applied to patients with primary pulmonary hypertension or Eisenmenger syndrome (with correction of the congenital shunt), for whom combined heart–lung transplantation was initially devised. As the utility of lung transplantation for these pulmonary diseases has been demonstrated, the need for heart–lung transplantation has diminished. There are now relatively few surgeons who have extensive experience with combined heart–lung transplantation while experience with bilateral-lung transplantation continues to grow, driven by demonstrable superior outcomes for most indications.4
RECIPIENT SELECTION
Selection of appropriate candidates is critical to the success of lung transplantation. The numerous factors that are considered when selecting candidates are discussed in detail in the following sections.
GENERAL CONSIDERATIONS
Recipient selection is one area where a remarkable international consensus has been achieved among key societies dedicated to the investigation and management of advanced respiratory diseases.24,25 The current published guidelines represent a conjoint effort of members of the ISHLT, American Thoracic Society, European Respiratory Society, American Society of Transplant Physicians, and the Thoracic Society of Australia and New Zealand.25 Published first in 1998 and revised in 2006, the guidelines are now under further revision as there have been major changes in acceptance of candidates, especially those needing advanced life support and those of more mature age.
In brief, the evaluation of a potential candidate for lung transplantation should include a complete assessment of cardiopulmonary function and the patient’s general health in addition to a thorough evaluation of psychosocial status. A battery of screening tests is required, as well as evaluation by members of the transplant team, including pulmonologists, cardiologists, thoracic surgeons, psychiatrists, and social workers (Table 107-1). Contingent on the patient’s status, this evaluation can be completed in many instances on an outpatient basis. A coordinated review of the results of these studies by the multidisciplinary transplant team serves to assure that the best candidates are accepted as potential transplant recipients. The so-called “best candidates” are those who are deemed to have a superior chance of obtaining both an immediate survival benefit and an enduring quality-of-life benefit. The introduction of the Lung Allocation Score (LAS) within the United States has been an attempt to provide potential lung transplant recipients with a uniform lung donor allocation system designed to achieve at least the first of these broad goals.26 One iconoclastic action of the LAS has been to abolish the “time-accrued” principle of determining who received the next lung transplant. The “time-accrued” principle valued the serendipity of time of listing greater than the acuity of the disease and led directly to an excess mortality on the waiting list and indirectly to overly large waiting lists at some institutions. Within a month of introducing the LAS the number of patients waiting for lung transplantation within the United States halved due to the acknowledgment by transplant centers that many on the list were there simply to accrue time but were not in immediate need of transplantation.
aSeptic lung disease.
bIf >50 years of age, coronary artery disease or LVEF <45%.
cExcluding patients with pulmonary hypertension.
INDICATIONS
Lung transplantation is a treatment of last resort. Potential recipients should be patients with end-stage pulmonary parenchymal, airways, or vascular disease who have a limited life expectancy and for whom no effective alternative therapy is available. Additional considerations include the degree of reduction in activities of daily living resulting from the disease and the patient’s quality of life. The variable rates of progression of the diseases for which lung transplantation is performed and the variety of supportive therapies available dictate the use of disease-specific criteria in assessing the appropriate timing of listing a given patient.
Immunologic study of potential transplant candidates includes assessment of ABO status and preformed anti-HLA antibodies. All patients are currently matched to donors by ABO status, most commonly with ABO-identical donors and less commonly with ABO-compatible donors (this situation arises commonly for blood type AB candidates). Screening for sensitization to HLA antigens is now routine and will be further discussed under the section on AMR. Pregnancy, blood transfusion, or prior transplantation can lead to HLA sensitization.27
CONTRAINDICATIONS
There are few absolute contraindications to lung transplantation. Over the years the impetus has swung from focusing on which patients should be excluded to how to reasonably include patients after appropriate evaluation and management. With newer support technologies, a number of the original exclusion criteria have been relaxed. Absolute contraindications include bone marrow failure and hepatic cirrhosis, the latter to be distinguished from reversible hepatic dysfunction due to right heart failure, which resolves following lung transplantation (Table 107-2). In exceptional circumstances, combined liver and lung transplantation may be contemplated, and in experienced hands this procedure has acceptable long-term outcomes. Active malignancy precluding long-term survival is also an absolute contraindication. One consideration that impacts the determination of who may receive a transplant is the current limited supply of donor lungs. Hence, other significant life-limiting comorbidities also stand as a proscription against lung transplantation.
A host of additional factors may be considered relative contraindications to lung transplantation (Table 107-2). The age of the recipient may be a significant factor in view of the limited number of donor organs and the presumed subclinical organ dysfunction associated with the aging process that increases the potential for postoperative complications. As the latter factor is variable, a “physiologic age” rather than a strict chronologic criterion is appropriate. The type of transplant procedure also influences the significance of age as a contraindication. Many centers consider single-lung transplantation as more suitable for older patients because of its lower risk. Nevertheless, there is a definite creep toward offering transplantation to the well-chosen older recipient for whom it may be the best palliative option. In fact, according to the OPTN/SRTR 2011 report, the largest expansion in the waiting list population for lung transplantation in the United States was over the 65 age group.28 Other contraindications are evidence of psychosocial instability that would preclude compliance with the necessary posttransplant regimens and active use of tobacco products or other substances of addiction during the wait for transplantation. Obesity or cachexia can increase the risk for perioperative morbidity.29–32 The same is true of the continued need for high doses of corticosteroid therapy (more than 20 mg of prednisone, or equivalent dose of another agent, per day).
Respiratory failure requiring mechanical ventilation before transplantation also increases the likelihood of complications.33 Some centers will not consider a new patient for evaluation who is completely ventilator dependent or who has acutely deteriorated and become ventilator dependent. However, this stance is definitely changing and many centers, particularly those in Europe and Australia, have championed the use of a high-urgency status to allow successful transplantation of patients on extracorporeal membrane oxygenation (ECMO).34 Patients who have a chronic need for partial ventilatory assistance or those who have been accepted as transplant candidates and require assisted ventilation because of progression of their native disease are often still considered potential recipients unless multiorgan failure intervenes.35 Prolonged mechanical ventilation results in colonization of the lower respiratory tract with significant microbiologic pathogens and a degree of deconditioning and protein wasting that significantly increases the perioperative risk of transplantation. Nevertheless, despite these theoretical concerns recent outcomes have improved significantly for well-selected patients needing assisted ventilation.
Chronic kidney disease may affect eligibility for lung transplantation. The calcineurin inhibitors (CNIs) universally used as part of posttransplant immunosuppressive regimens are nephrotoxic and invariably cause some degree of renal insufficiency as a complication of therapy. As with irreversible hepatic dysfunction, combined renal and lung transplantation may be considered, but the potential risks of the procedure, particularly in the context of the shortage of donor lungs, require careful consideration. In most patients, severe pre-existing renal insufficiency is a contraindication for lung transplantation.
Severe peripheral vascular disease may be a limiting factor in selecting candidates for a number of reasons including the occasional need for cardiopulmonary support in the perioperative period by either partial cardiopulmonary bypass or ECMO via the femoral or subclavian routes. Peripheral vascular disease is also frequently associated with occult significant coronary or aortic disease, which may greatly increase the morbidity and mortality of the lung transplant procedure and limit quality long-term survival. Finally, transplantation is contraindicated in patients with gangrenous changes in the extremities due to peripheral vascular disease because of the potential for systemic spread of the infectious process during immunosuppression.
Careful consideration is required before patients with pre-existing osteoporosis are accepted for transplantation. In addition, chronic steroid immunosuppressive therapy causes bone loss in all patients and exacerbates the complications of prior osteoporosis. Post operative rib fractures or compression fractures of the vertebrae not only cause severe pain requiring the use of narcotic analgesia but limit cough, mobility, and rehabilitation and can lead directly to sputum retention, pneumonia, and death.
Infectious diseases have a profound effect on the morbidity and mortality of lung transplantation. Colonization of the respiratory tract with potential pathogens in patients with end-stage pulmonary disease requires careful assessment. Most bacterial flora in transplant candidates have a pattern of antibiotic sensitivity that can be identified preoperatively to select an appropriate perioperative antibiotic regimen. For example, lung transplant patients harboring gram-negative bacilli preoperatively were found to be at risk for posttransplant pneumonia, demonstrating the importance of preoperative identification and a plan for eradication of potential pathogens. However, Burkholderia cenocepacia (BCC), a pathogen found in up to 15% of patients with cystic fibrosis in some geographic regions, is often highly resistant to antimicrobials and is a relative contraindication to transplantation unless a suitable pattern of antibiotic sensitivity can be identified before transplantation. Irrespective of the precise genomovar, it is the biologic behavior of the organism within a particular host that determines outcome.36,37 Aspergillus fumigatus and other Aspergillus species are also common pathogens in the sputum of patients with bronchiectasis, sarcoidosis, or COPD and, while not a contraindication to transplant, these organisms can occasionally lead to life-threatening posttransplant infections.38–41 Other pathogens such as Scedosporium species may be associated with fatal dissemination post transplant.38–41 Scedosporium prolificans in particular may be difficult to control and is hard to eradicate.42,43 Lifelong therapy with voriconazole and terbinafine may be effective but carries the risk of accelerated development of skin cancers especially squamous cell cancer of the lip.44 Interestingly, the presence of organisms in donor lungs is not always a predictor of post–lung transplant pneumonia, perhaps reflecting the efficacy of targeted antibiotic therapies or the successful eradication with removal of the diseased lungs.
Viral diseases in a potential lung transplant recipient can also have a significant impact on the outcome of transplantation. Active hepatitis B or C in the lung transplant candidate is associated with increased early and late mortality because of the effect of hepatic dysfunction on perioperative complications and the accelerated progression of these diseases in patients requiring chronic immunosuppression. However, successful lung transplantation has been reported in patients in whom therapy for hepatitis C has been effective.45 Cytomegalovirus (CMV), a DNA-type virus that is incorporated into the host genome, can cause both systemic illness and pneumonitis in immunosuppressed patients. Therefore, the serologic CMV status of the recipient is an important determination to make before transplantation. An early publication in the preprophylaxis era found that CMV infection developed in 54% (32/59) of patients who underwent heart–lung or lung transplantation and survived for more than 30 days, and that CMV infection was more common in patients who had been CMV seropositive prior to transplant (95%) than those who had been seronegative preoperatively (38%).46 While some centers still prefer to match CMV-negative recipients with CMV-negative donors as a strategy for reducing the chance of primary CMV infection, the widespread use of prophylactic ganciclovir and valganciclovir therapy has been shown to significantly reduce the risk of CMV disease in transplant patients. Extended prophylaxis with valganciclovir appears both safe and effective.47–51 However, some strains of CMV are resistant to ganciclovir, hence ongoing surveillance for CMV using PCR assays and transbronchial lung biopsy is advised.52
SPECIFIC DISEASE STATES
In addition to general selection criteria, there are disease-specific considerations that impact decisions about candidate selection and timing of listing.
CHRONIC OBSTRUCTIVE PULMONARY DISEASE
The rate of progression of the underlying disease is an important factor in the timing of the evaluation and selection of potential transplant recipients (Table 107-3). The most common indication for lung transplantation is obstructive lung disease, with COPD accounting for more than one-third of all lung transplants and emphysema due to α1-antitrypsin deficiency accounting for 8% of all lung transplants. Patients with these diseases tend to remain relatively stable for long periods, albeit with a reduced quality of life. It is now apparent that lung transplantation not only provides marked symptomatic and functional palliation for these patients but improved survival as well, depending on the severity of disease at the time of transplant.53 The recently devised BODE index (Table 107-4) that incorporates multiple factors (B, body mass index; O, obstruction as indicated by the FEV1 as % predicted; D, degree of dyspnea; and E, exercise capacity) is a validated survival measure that is more accurate in predicting survival in individuals with COPD than the FEV1 alone.54–56 Accordingly, calculation of the BODE index is now included in the international guidelines for referral and transplantation for COPD (Table 107-3). Prior to the introduction of the LAS, death during the wait for transplantation was rare in these patients, occurring in less than 5% of cases. In addition to reflecting the slow progression of COPD, two additional factors may contribute to their low mortality: their participation in a graduated rehabilitation program while they await transplantation, which may maintain or even increase their functional capacity, and the institution of oxygen therapy, which improves survival in patients with obstructive lung disease.
TABLE 107-3 Disease-Specific Considerations for Lung Transplantation
TABLE 107-4 The BODE Index for COPD
INTERSTITIAL LUNG DISEASES
Interstitial lung diseases are the indication for lung transplantation in 24% of patients who undergo lung transplantation. The most common cause is idiopathic pulmonary fibrosis (IPF), whereas a variety of interstitial lung diseases including sarcoidosis, collagen vascular disease–associated interstitial lung disease, and drug-induced lung disease account for the remainder.
The end-stage fibrotic lung is characterized by severe destruction of gas exchange units, secondary distortion and dilatation of the airways with development of cystic lesions, and replacement of the lung with nondistensible fibrous tissue. The work of breathing in these patients may be increased five times above normal because of the increased elastic load. The vital capacity in patients with pulmonary fibrosis is severely reduced, as is the functional residual capacity and total lung capacity. Dead-space ventilation is increased, and may actually increase further during exercise. A marked reduction in diffusing capacity is invariably present. Although some degree of alveolar hyperventilation is common early in the course of disease, hypercapnia during exercise and later at rest is encountered in the advanced stages. Extensive intrapulmonary shunting of blood flow is seen, resulting in hypoxemia and, in later stages, pulmonary hypertension. Progression of the disease may be variable, but patients often deteriorate precipitously, developing progressive hypoxemia and pulmonary hypertension. Acute exacerbations of IPF are associated with mortality exceeding 50% irrespective of therapy. As a result, the mortality of these patients while they await transplantation is more than 20%. Criteria for considering IPF patients for transplantation include severe dyspnea, forced vital capacity less than 50% of predicted, resting arterial hypoxemia or hypercapnia, and pulmonary hypertension. However, a downhill clinical course is the best individual indication for transplantation. It must be emphasized that there is currently no proven therapy that reverses established IPF.57–59
Two other factors in patients with interstitial lung disease are also important. First, many of these diseases are systemic, and the effects of the disease on extrapulmonary organs may result in a sufficient number of relative contraindications to exclude the patient from consideration for transplantation. The presence of an underlying connective tissue disease should be investigated in appropriate cases, and an individual determination made about the possibility of an enduring benefit from lung transplantation. Second, a number of these diseases, including sarcoidosis and lymphangiomyomatosis, may recur in the lung graft, but typically do not impact posttransplant survival.
SEPTIC LUNG DISEASE
Septic lung disease, including cystic fibrosis and other types of bronchiectasis, accounts for approximately 20% of patients undergoing lung transplantation. Candidates with focal or unilateral disease can sometimes be managed with medical treatment or surgical resection of the affected area. In most patients, however, the disease is bilateral or systemic, and the natural history is one of recurrent infection and progressive respiratory failure. It is important to attempt to establish a cause of the bronchiectasis before transplant evaluation, because of the impact of systemic diseases on management before and after transplantation. Cystic fibrosis can be diagnosed with a sweat test or from genotyping. Serum immunoglobulin levels should be measured and a careful assessment for evidence for a systemic illness – such as rheumatoid arthritis, ulcerative colitis, or immotile cilia syndrome – should be undertaken. Primary infectious causes, such as tuberculosis and allergic bronchopulmonary aspergillosis, should be identified and treated appropriately before consideration of the potential benefit of transplantation can be evaluated fully. Finally, any suggestion of aspiration as a primary or secondary factor demands further investigation, including a barium swallow and esophageal manometry testing to rule out gastroesophageal reflux.
Many of these patients demonstrate significant short-term improvement in response to optimum medical therapy, which includes postural drainage, intravenous and/or inhaled antibiotics, and nutritional supplementation. Once medical therapy has been optimized, however, a pattern of more frequent hospitalizations for exacerbations, continued weight loss, and progressive functional impairment is indicative of a patient who has a limited life span and should be given priority for transplantation. Cystic fibrosis patients with an FEV1 under 30% of the predicted value, a PaO2 under 55 mm Hg, or a PaCO2 greater than 50 mm Hg have a 2-year mortality of 50%; the FEV1 appears to be the most sensitive predictive factor. Any patient with septic lung disease who manifests these criteria should be further evaluated as a potential transplant recipient.
While the patient is awaiting transplantation, close medical follow-up is required, and all components of the patient’s therapeutic regimen should be continued. Serial study of sputum microbiology is important for assessing changes in flora. Aerosolized broad-spectrum antibiotic therapy may reduce the colonizing bacterial load while minimizing the potential for renal toxicity and in some cases it may transiently improve functional capacity. In the event of progressive hemoptysis, bronchial artery embolization can provide adequate short-term control of the bleeding without significantly compromising technical aspects of the transplant procedure. Finally, institution of noninvasive positive pressure ventilation in the patient who is approaching respiratory failure has been shown to stabilize lung function without adversely affecting the outcome of transplantation. Because of the shortage of donor organs and the variability in the progression of the disease some programs had previously developed the approach of live donor lobar transplantation of the lung. This uses bilateral lobar transplants from two live donors, often parents or close relatives, particularly for small recipients with cystic fibrosis. It has proven to achieve outcomes comparable to cadaveric donor transplantation without donor mortality or significant morbidity.60 The use of this procedure in the United States has largely been abandoned as the implementation of the LAS system has provided an alternative means of expediting transplantation for severely ill patients but the procedure has now gained popularity in Japan.61–65
PULMONARY VASCULAR DISEASE
Pulmonary vascular disease, either idiopathic pulmonary arterial hypertension (IPAH) or secondary pulmonary arterial hypertension due to Eisenmenger syndrome, accounts for 4% to 5% of patients requiring lung transplantation and approximately 25% of patients requiring heart–lung transplantation. The criteria for identifying patients who may require transplantation relate to the risks of death due to the underlying disease. On the basis of data from the National Heart, Lung, and Blood Institute registry, it is apparent that an NYHA class III or IV functional status, an elevated central venous pressure, a decreased cardiac index, and an elevated mean pulmonary artery pressure correlate with a poor prognosis. Episodes of near-syncope or syncope, which tend to occur later in the course of the disease, are also associated with mortality. The changing landscape of medical therapy for pulmonary arterial hypertension has had a major impact on the survival of these patient populations and the timing of referral and listing for lung transplantation. A wide variety of effective therapies are now available, including traditional therapies with anticoagulation and high-dose calcium channel blockers as well as newer vasodilator drugs such as sildenafil, endothelin receptor antagonists, and inhaled, subcutaneous and intravenous prostanoids.66 These therapies may significantly modify the natural history of the disease. Therefore, symptomatic patients with pulmonary arterial hypertension who do not respond to medical therapy are the ones best considered for transplantation. Early referral before the development of end-stage organ system failure is critical to success. Although the vast majority of data concerning the natural history of patients with pulmonary arterial hypertension is derived from the IPAH population, similar clinical criteria are applied to those with Eisenmenger syndrome in deciding when to list for lung transplantation. Notably, however, many patients with Eisenmenger physiology are well compensated for many years and transplantation can often be delayed much longer than for patients with IPAH.
The decision as to what procedure a patient with pulmonary vascular disease should undergo has evolved over time. The relative shortage of suitable heart–lung donor blocs combined with a mortality of 20% to 25% among patients with significant pulmonary hypertension awaiting heart–lung transplantation, has led to a shift away from this procedure in favor of bilateral-lung transplantation. The results with bilateral-lung transplantation are similar to those with heart–lung transplantation for pulmonary vascular disease provided that there is no significant left ventricular dysfunction. Of interest, the presence of severe right ventricular systolic dysfunction does not appear to affect the results of lung transplantation; the severe tricuspid regurgitation and pulmonary valvular regurgitation that are present in virtually all patients preoperatively resolve almost immediately and right ventricular function normalizes over a period of weeks to months. Patients with Eisenmenger syndrome who have a shunt defect that can be corrected at the time of transplantation are also candidates for lung transplantation. Thus, heart–lung transplantation is primarily limited to patients with either significant biventricular dysfunction or uncorrectable congenital heart defects.
TRANSPLANT PROCEDURE SELECTION
Except for patients with bilateral septic lung disease or severe pulmonary arterial hypertension, single-lung transplantation is potentially suitable for the majority of end-stage pulmonary diseases that require transplantation (Table 107-5). However, there has been a very definite swing away from single-lung transplantation and toward bilateral-lung transplantation worldwide, based in part on the lower long-term survival outcomes associated with the former procedure, particularly when applied to the COPD population.4,67 The argument that single-lung transplantation is associated with a shorter wait for donor lungs and a lower morbidity and mortality rate after transplantation than other lung transplant procedures performed for the same recipient diagnoses is no longer tenable given improvements in surgical experience and techniques combined with the ramifications of the LAS. The surgical mortality for single-lung transplantation ranges from 3% to 10%, relating to the specific transplant indication, the presence or absence of pulmonary hypertension, and the intraoperative need for cardiopulmonary bypass. The incidence of graft failure within the first 6 weeks following single-lung transplantation approximates 5%.28
Bilateral-lung transplantation is the procedure of choice for patients with septic lung disease, such as cystic fibrosis, and for patients with severe pulmonary arterial hypertension from either primary or secondary causes. Many centers also favor bilateral-lung transplantation for patients with emphysema who are less than 60 to 65 years of age.67 More controversial is the preferential use of bilateral-lung transplantation for patients with fibrotic lung disease in the absence of severe secondary pulmonary hypertension. As currently performed, each lung is sequentially resected and the donor lung implanted; that is, two single-lung transplants are performed sequentially at the same operative sitting. This is in distinction to the original double-lung transplant, which used a double-lung bloc and a tracheal anastomosis. Bilateral-lung transplantation is performed via a bilateral thoracosternotomy incision, median sternotomy, or bilateral anterior thoracotomy incisions. The latter has the decided advantage of avoiding transection of the sternum and thereby preventing any chance of sternal infection and dehiscence, a major complication that can be associated with significant morbidity and occasional mortality. Surgical mortality is now equivalent for bilateral- and single-lung transplantation. Similarly, at most large centers, perioperative rates of acute graft failure and bronchial dehiscence are similar.
Combined heart–lung transplantation has been used successfully for virtually all end-stage pulmonary diseases. However, with the perfection of the techniques of single and bilateral transplantation, and in light of the significant limitations in supply of donor organs, the use of heart–lung transplantation has focused on patients with significant intrinsic left ventricular dysfunction or Eisenmenger syndrome and surgically irreparable shunt defects. The surgical mortality for heart–lung transplantation at large centers is about 10%; typically, it is higher than the surgical mortality for single or bilateral transplantation for similar disease states. Notably, the number of procedures performed worldwide has fallen with the realization that most recipients can be well serviced with lung transplantation alone.
DONOR SELECTION
The most significant factor limiting wider application of lung transplantation is the supply of donor organs.68 Unlike other solid organs used for transplantation, the lung is exposed before brain death to environmental contamination, including both microbiologic pathogens and toxic substances, which may significantly impair its functional capabilities. The microbiologic aspects of this exposure are accentuated by the endotracheal intubation that is a necessary aspect of donor management. In addition, aspiration of oropharyngeal or gastric contents is a common occurrence during the events preceding brain death. Nearly half of all comatose patients develop pneumonia within 1 week of intubation, probably owing to a combination of these factors. Brain death itself may also lead to neurogenic pulmonary edema. In cases of trauma that lead to brain death, significant injury to the thorax may occur, or the volume replacement required for the resuscitation of these patients may limit the suitability of the lungs for subsequent transplantation. In addition to these immediate insults, a history of significant cigarette smoking raises concerns about more chronic damage to donor lungs.
As a result of these factors, only about 25% of cadaveric organ donors have historically proven to be potential lung donors. In recent years concerted efforts to improve donor supply and optimize donor utilization worldwide have proved fruitful, pushing the lung recovery rate up to approximately 50% in some centers. In part, this has been facilitated by an increasing willingness to use donors that were formerly called “marginal donors,” a practice that has proven to be safe in most circumstances. The advent of ex vivo lung perfusion to “recondition” lungs following recovery heralds a most exciting phase in the development of the science of lung transplantation and holds great promise of optimizing donor lungs and allowing salvage of lungs that formerly would not have been considered suitable for organ donation.6,7,69,70
Criteria for lung donation are meant to identify donors with evidence of acceptable gas exchange in the absence of infection of the airways or parenchyma (Table 107-6). A donor age of less than 60 years and a history of smoking for less than 20 to 30 pack-years are important. Both increasing age and prolonged tobacco use are known to correlate directly with anatomic alterations in the pulmonary parenchyma, which, despite preservation of gas exchange function in the donor, may result in impaired graft function in the recipient. Despite these theoretical considerations the ultimate decision to use or not use a particular donor often depends on the status of the potential recipient. It is argued that it is preferable to use a donor that has smoked than miss a transplant opportunity that may equate to a death on the waiting list.
The chest radiograph should reveal normal lung on the side(s) of the lung(s) considered for donation. Unilateral pneumonia or parenchymal trauma does not preclude use of the contralateral lung for transplantation in most circumstances. No major thoracic surgery should have been performed on the side of proposed donation, not only because of potential technical limitations but also because such a history usually suggests either a major anatomic abnormality (e.g., prior lobectomy) or pathology (e.g., malignant neoplasm), which would preclude donation.
Finally, the size of the donor lungs, based on direct measurement or correlated to predicted total lung capacity – as estimated by donor height, age, and gender – is a useful parameter to use when selecting lungs for a particular recipient. The simple expediency of measuring lung heights (apex to base) and transthoracic diameter correlates well with predicted total lung capacity and is a time-honored method of determining lung size. Generally, the donor lungs should be within 25% of the predicted size of the recipient’s lungs. Donor lungs larger than these measurements can be volume reduced at the time of transplantation, whereas donor lungs considerably smaller than these measurements usually should be avoided. As a rule of thumb, donor organs should be smaller than the actual size of the hyperinflated native lungs of the recipient with emphysema so that the optimal length–tension relationships of the respiratory muscles can be reestablished. Conversely the donor for a patient with restrictive physiology should be larger than the actual size of the recipient native lungs so that after remodeling of the chest cavity the new total lung capacity approximates normal.
Adequate oxygenation has been defined as a PaO2 greater than 300 mm Hg on mechanical ventilation, with an FIO2 of 1.0 and positive end-expiratory pressure (PEEP) of at least 5 cm H2O. However, this is both arbitrary and dynamic. It is the trend that is most important and lungs with initial poor gas exchange due to a host of factors such as edema or atelectasis may well be suitable. It is the challenge of the donor retrieval team to analyze closely the operational factors to determine how to maximize the function of any potential donor organ. A “low stretch” ventilator protocol (i.e., tidal volume 6 mL/kg) should be utilized and recruitment maneuvers should be employed to maximize oxygenation. If a unilateral pulmonary process is present, a lower PaO2 may be acceptable because of the possibility of mixing of venous blood from the two lungs at the level of the left atrium. In this circumstance, intraoperative evaluation of unilateral gas exchange by sampling from the ipsilateral pulmonary vein for determination of PO2 can be used to determine that the prospective donor lung is satisfactory.
All lung donors have some evidence of colonization of the lower respiratory tract by potential pathogens owing to the requisite endotracheal intubation, which bypasses the defense mechanisms of the upper airway. A distal tracheitis is uniformly present after 72 hours of intubation. Therefore, a sputum Gram’s stain revealing polymorphonuclear leukocytes or multiple bacterial forms does not necessarily imply invasive infection. For this reason, bronchoscopy is a critical step in the evaluation of any potential lung donor. Bronchoscopy allows inspection of the large airways for the presence of aspirated debris as well as assessment of the character of the secretions and status of the bronchial mucosa. A finding of diffuse bronchial mucosal inflammation is significant, even if only a limited amount of aspirated debris or secretions are present. However, purulent secretions without significant mucosal inflammation in the presence of a clear chest radiograph and preserved gas exchange generally indicate a suitable lung for donation. A potassium hydroxide smear for fungal organisms is also a part of the routine evaluation of the lung donor, although as with the Gram’s stain, the mere presence of fungal organisms, particularly Candida species, does not preclude lung donation. In most cases, the presence of potential pathogens in the donor sputum by either Gram’s stain or fungal smear requires preemptive modification of the recipient’s antimicrobial regimen if such lungs are used for transplantation. At some centers, this treatment is begun by the administration of intravenous or aerosolized antimicrobial therapy to the donor before retrieval of the donor lungs. Prophylaxis for Candida and fungal organisms found in the donor swabs should be prolonged to prevent dehiscence of vascular and airway anastomoses.
The donor evaluation is completed by intraoperative inspection of the pleural space and lung. Occasionally, unsuspected parenchymal trauma is evident in the form of a bloody pleural effusion or pulmonary contusion. The donor lung also should be studied for evidence of unsuspected bullous disease or mass lesions. Excisional biopsy and intraoperative pathologic evaluation of any parenchymal mass lesion should be carried out. Finally, the anesthesiologist should be directed to maintain adequate tidal volumes and PEEP during intraoperative ventilation to preserve optimal function of the donor lung before its removal.
The appropriateness of a potential lung donor always should be interpreted in the context of the recipient’s disease and clinical status. Older patients and patients with a sudden clinical deterioration, such as those who have recently been placed on mechanical ventilation, may all benefit from transplantation with a lung that does not fulfill all the criteria of an optimal donor lung. Most frequently, the criteria relating to cigarette smoking and PaO2 are breached in these circumstances. The results have generally been satisfactory in such recipients, suggesting that the use of “marginal” lung donors may partly address the problem of donor organ shortage.71 It has also been shown that the effect of the functional status of the donor lung is most significant in the first 24 hours after transplantation, and that subsequent graft function depends primarily on factors related to the recipient. In addition, these studies have underscored that patients with pulmonary hypertension, who are the most difficult to manage postoperatively, are best served by transplantation with lungs from optimal donors.
LUNG PRESERVATION
The ideal method of pulmonary preservation has not yet been identified. With current techniques, however, satisfactory graft function can be obtained after ischemic intervals as long as 6 to 8 hours. As with other vascular solid organs used for transplantation, the lung consists of a heterogeneous population of cells, of which the vascular endothelial cell appears to be the most sensitive to ischemia. Ischemic injury to the pulmonary vascular endothelium increases its permeability and results in pulmonary edema, the common end point for assessment of injury in models of pulmonary preservation techniques. Hypothermia is the major method used clinically to limit ischemic injury to these cells. The lung also has some unique biologic and physical characteristics that distinguish it from other solid organ transplants. Although it has an absolute requirement for aerobic metabolism, the lung is capable of using ambient oxygen for the metabolism of glucose, even during the ischemic state. In addition, the effective size of the pulmonary vascular bed and thermal conductivity of the lung can be manipulated by the degree of lung inflation. Current clinical methods of lung preservation make use of these characteristics to optimize graft function following an ischemic interval.
Two techniques are currently being used for lung preservation, core cooling and hypothermic flush perfusion. Extracorporeal core cooling (ECC) is a technique that has been used primarily for procurement of heart–lung donor blocs, commonly in conjunction with multiorgan procurement at abdominal sites. ECC consists of systemic heparinization of the donor and institution of full cardiopulmonary bypass (CPB) by means of a transpericardial approach. The donor is cooled to 15°C (rectal temperature). Ventricular fibrillation typically develops during this maneuver, and the heart is decompressed through the left ventricle. CPB is then discontinued, and the heart–lung bloc is harvested and transported in a cold ischemic state with the lungs inflated. No flush solutions are used, although the lungs are essentially being flushed by cooled autologous blood during the time of CPB. Safe ischemic times of 6 hours or more have been reported with adequate pulmonary function. It is of interest that while oxygenation in lungs preserved by ECC appears to be somewhat less optimal than in those preserved by hypothermic flush techniques, the pulmonary vascular resistance (PVR) upon reperfusion of the lungs following ECC is generally lower than that seen upon reperfusion of lungs obtained by flush techniques.
Hypothermic flush perfusion is the method most commonly used for pulmonary preservation in clinical practice. This technique consists of flushing the pulmonary vasculature with a cold solution after systemic heparinization of the donor, followed by extraction and transport of the lungs inflated with 100% oxygen. Flushing can be performed in antegrade fashion via the pulmonary artery or retrograde fashion via the left atrium or pulmonary veins. Many centers favor the retrograde technique as it appears to be more effective in flushing out clots from the pulmonary arterial circulation thereby improving gas exchange by enhancing ventilation and perfusion matching. A low-potassium dextran solution is used. The ideal pulmonary preservation solution is a topic of much debate.
The state of inflation of the lungs is important in obtaining optimal perfusion of the pulmonary vasculature by the flush solution, to achieve both rapid cooling and direct cellular preservation by the solution itself. Intraoperatively, maintaining a tidal volume similar to that used during the initial donor assessment is important. The addition of PEEP during the procurement procedure maintains functional residual capacity and the desired state of inflation of the donor lungs. PEEP also increases the intra-alveolar release of surfactant, minimizing pulmonary compliance abnormalities after implantation of the donor lungs. Ventilation is continued throughout the period of lung perfusion to maintain the effective size of the pulmonary vascular bed. Although it was once thought that the maintenance of an FIO2 of 1.0 during the procurement was useful, particularly at the time of lung extraction, to provide an oxygen-rich ambient environment for metabolic activity of the lung during the ischemic interval, new information suggests that use of high FIO2 is associated with a higher risk of primary graft dysfunction (PGD). The lungs are extracted and transported in a state of inflation that approximates end-tidal inspiration. Some consideration should be given to the fact that the donor lungs may be transported by aircraft, in which a fall in atmospheric pressure may result in further inflation of the lungs. Overinflation of the donor lungs is to be avoided, as this leads to increased capillary permeability and postimplantation pulmonary edema.
The administration of prostanoids, either prostaglandin E1 (PgE1) or prostacyclin, into the pulmonary circulation before flush perfusion has been shown to improve lung preservation. The mechanism of action of prostanoids includes dilation of the pulmonary vasculature, allowing for better distribution of the flush solution, and decreased leukocyte adhesiveness, which can abrogate the initial events of reperfusion injury. Most commonly in North America, PgE1 is used as a bolus (500 μg) into the pulmonary circulation, with or without the addition of similar amounts of PgE1 directly to the flush solution. The use of prostanoids in combination with intracellular flush solutions has been shown to provide pulmonary preservation equivalent, if not superior, to that with the use of extracellular-type flush solutions alone.
Most flush solutions are administered at a temperature of 4°C, while topical cooling is carried out by filling of the pleural cavity with iced crystalloid solution. After extraction, the lungs are immersed in crystalloid and packed in ice, resulting in a transport temperature of 1° to 4°C. Some studies have shown that lung preservation is superior when a more moderate hypothermia with a temperature of 10°C is used. However, because of the concerns regarding the deleterious effects of flush and storage temperatures greater than 10°C, and the difficulties in maintaining this temperature during the procurement procedure, clinical flush perfusion continues to be performed at the lower temperature ranges.
Experimental work has identified numerous adjuncts to the techniques currently used for pulmonary preservation that have the potential for prolonging ischemic intervals. A significant part of the lung injury seen after ischemia has been shown to be due to the phenomenon of reperfusion, which is initiated by leukocyte adhesion to endothelial cells and the production of oxygen-derived free radicals and peroxides. Measures that diminish this response, in addition to the use of prostanoids, include donor leukocyte depletion, the administration of antibodies to block adhesion molecules, the use of inhaled nitric oxide, and the inclusion of oxygen radical scavengers, such as superoxide dismutase or catalase, to the flush solution. In addition, methods of increasing the resistance of cells to ischemic injury, such as the induction of heat shock proteins, have been shown to be beneficial in other organs and may be of some use in lung preservation. Evidence of the effect of these manipulations on tolerable ischemic intervals in clinical lung transplantation awaits additional study.
TECHNIQUES OF LUNG TRANSPLANTATION
Technical aspects of lung transplantation include both anesthetic and surgical considerations. Single lung, bilateral lung, and heart-lung transplantation are the surgical options currently utilized.
ANESTHETIC MANAGEMENT
Proper perioperative management of the recipient is crucial to obtain the best outcome following lung transplantation.72 Close cooperation and understanding between the anesthesiology and surgical teams are essential. An appreciation of the unique aspects of the physiology of the various types of lung transplant recipient is also important. Patients with COPD have reduced expiratory flow rates, air trapping, and increased lung volumes. Following endotracheal intubation, extreme care should be taken to allow adequate expiratory time for emptying of the lungs, avoiding the cardiovascular instability caused by “pulmonary tamponade” due to progressive air trapping in the lungs and reduction of ventricular filling. Tension pneumothorax due to rupture of bullae can also occur but is relatively uncommon. Pneumothorax is particularly difficult to diagnose in the supine patient and may be missed on fluoroscopy especially if there is an anterior air collection. Patients with restrictive lung disease have progressive fibrosis of the lung tissue, with secondary hypoxemia and progressive pulmonary hypertension. Patients with these diseases have an increased work of breathing and are oxygen dependent and extremely dyspneic before transplantation. Many of these patients have evidence of cor pulmonale at the time of transplantation and cannot tolerate occlusion of the pulmonary artery during implantation of the donor lung without the support of cardiopulmonary bypass. Careful and repeated assessment of filling pressures and cardiac output is required to allow prompt interventions in such patients. Patients with septic lung disease demonstrate primarily the abnormalities in pulmonary function seen in patients with obstructive airway disease. However, these recipients have excessive copious purulent secretions, which can exacerbate air trapping and also contribute to marked V/Q abnormalities – particularly during single-lung ventilation. Careful management of double-lumen endotracheal tubes to avoid contamination of the contralateral lung graft and attention to bronchopulmonary hygiene to avoid obstruction of the lumens of these tubes are needed in these patients. Finally, recipients with pulmonary vascular disease who present for transplantation have marginally compensated cor pulmonale and are extremely dyspneic and anxious. Patients with IPAH become oxygen dependent late in the course of their disease, although oxygen is commonly administered to these patients to lessen the hypoxic contribution to their pulmonary hypertension. For this reason, oxygen therapy should be continued throughout the time of preoperative preparation and line placement to avoid abrupt right heart dysfunction. Because these patients have normal pulmonary mechanics, they generally tolerate mechanical ventilation well. Patients with Eisenmenger syndrome are well adapted to chronic hypoxemia. In these patients, supplemental oxygen does not reverse the hypoxemia and may even worsen arterial hypoxemia by eliciting systemic vasodilatation and increasing right-to-left shunting.
All lung transplant procedures should be performed with CPB available on standby, if not utilized by surgeon choice. There is an argument to avoid CPB in cases of septic lung disease or other situations where there are known extensive intrapleural adhesions, to avoid excessive bleeding complications, but as always it is a balance of experience and safety. No specific preoperative factors can be used to predict the need for CPB—with the exception of severe pulmonary arterial hypertension, for which CPB is requisite for the procedure. For other lung transplant recipients, assessment of the need for CPB is best made intraoperatively by trial clamping of the pulmonary artery, followed by assessment of hemodynamic parameters, oximetry, and if available, ventricular function testing by transesophageal echocardiography. Progressive deterioration in these parameters requires unclamping of the pulmonary artery and an attempt to optimize factors such as preload, inotropic support, PaO2, PaCO2, and PVR. If repeat trial clamping of the pulmonary artery is still not tolerated, plans for CPB are made. Typically, cannulation after systemic heparinization is via the femoral vessels for single-lung transplantation and via a transpericardial approach for patients undergoing bilateral-lung or heart–lung transplantation.
SINGLE-LUNG TRANSPLANTATION
The approach to single-lung transplantation requires an initial decision regarding the side of implantation. Most commonly, the native lung with the least pulmonary function based on preoperative V/Q scans is excised. In some patients, however, specific technical factors, such as a prior pleurodesis, may override this factor. When the function of the two lungs is equal or when the need for CPB is anticipated, the right side is preferred because of the greater ease of surgical exposure and the institution of CPB via the ascending aorta and right atrium. A right-sided approach also facilitates exposure for closure of intracardiac defects in patients with Eisenmenger syndrome. Despite the potential differences in size of the right and left hemithorax, there does not appear to be any long-term difference in outcome following right or left single-lung transplantation.
Most often, exposure for single-lung transplantation is via a generous posterolateral thoracotomy through the fifth intercostal space or the bed of the excised fifth rib. When elective CPB via the right hemithorax is planned, the use of a fourth interspace may facilitate placement of the cannulae. The ipsilateral groin is included in the surgical field in the event that cannulation of the femoral vessels is required for partial CPB. Although the use of femoral sites for cannulation requires repair of the vessels after removal of the cannulae, it does provide a site for additional venous drainage with use of intrathoracic cannulation sites. Femoral cannulation sites also provide access for conversion to ECMO support if acute graft failure occurs immediately after implantation. Occasionally, when the repair of an associated intracardiac defect requires an anterior approach, a median sternotomy may be used for right single-lung transplantation in patients with Eisenmenger syndrome (though single-lung transplantation is rarely used for this indication).
The donor lung is prepared for implantation and then wrapped in sponges soaked with cold crystalloid solution and placed into the hemithorax. The bronchial anastomosis is performed first. Although a variety of techniques have been described, the essential points are to minimize the length of both the donor and recipient bronchi to preserve collateral blood supply and achieve some degree of anastomotic overlap. An end-to-end anastomosis is preferred (Fig. 107-1). Occasionally, it is necessary to telescope the smaller bronchus, most commonly the donor bronchus, into the larger bronchus with either a technique of interrupted sutures or a combination of running sutures on the membranous wall and interrupted sutures on the anterior wall in a figure-eight or horizontal mattress fashion. Polyfilament absorbable suture (e.g., 4-0 polyglactin) or monofilament suture, either absorbable (e.g., polydioxanone) or nonabsorbable (e.g., polypropylene), may be used. The anastomosis is then covered by either local peribronchial tissue or local pedicled flaps of thymic tissue or pericardial fat.
Figure 107-1 Bronchial anastomosis for lung transplantation. A technique of approximation using stay sutures at the junction of the cartilaginous and membranous walls is shown. If the donor and recipient bronchi are size matched a continuous monofilament suture can be utilized. If there is a significant size mismatch alternative techniques are employed to minimize puckering. A running suture is used for the membranous wall, followed by an interrupted suture technique of horizontal mattress sutures on the cartilaginous wall to achieve an end to end anastomosis. Additional anastomotic coverage may be obtained by approximation of peribronchial and mediastinal tissues about the site.
The order of the vascular anastomoses can vary even though the pulmonary artery anastomosis is frequently the more technically difficult to perform. A continuous 5-0 polypropylene suture is used for each anastomosis, leaving the ends untied for deairing upon reperfusion of the lung. For the pulmonary artery anastomosis, the length of the donor and recipient vessels requires careful assessment to avoid kinking. For the left atrial anastomosis, the confluence of the recipient pulmonary veins is incised to create a left atrial cuff. Occasionally, dissection in the interatrial groove is required to allow more proximal placement of the vascular clamp on the recipient left atrium (Fig. 107-2). After completion of these anastomoses, the lung is gently reinflated. Perfusion of the lung graft is then reestablished, initially in an antegrade fashion, evacuating air via the left atrial suture line. The atrial clamp is removed, with the atrial suture line under a fluid level to prevent entrainment of air into the left heart. Ventilation of the donor lung is resumed, and after a few minutes to allow the vascular suture lines to adapt to the distention caused by increased flow, these suture lines are secured. Hemostasis is then obtained, two chest tubes are placed, and the chest is closed in a standard fashion. Following reintubation with a single-lumen tube, flexible bronchoscopy is completed to inspect the bronchial anastomosis and clear the airway of blood or residual secretions.
Figure 107-2 Implantation of the donor lung at the right hilum. The bronchial anastomosis is performed first, followed by the venous then arterial anastomoses. A clamp is placed on the left atrium within the pericardium. After excision of the pulmonary vein stumps, the confluence of the pulmonary veins is incised to create a cuff of left atrium. The atrial anastomosis is performed with a running monofilament suture following approximation with stay sutures superiorly and inferiorly. The pulmonary arterial anastomosis is performed last. A clamp is placed on the proximal pulmonary artery. Usually the anastomosis is proximal to the upper lobe pulmonary artery branch on the right side however if there is a size mismatch the anastomosis may be distal to this branch. On completion of the anastomosis, the sutures are left untied until lung reinflation and antegrade reperfusion is completed to evacuate air from the donor vasculature.
BILATERAL-LUNG TRANSPLANTATION
Although most commonly referred to as bilateral-lung transplantation in the medical literature, the most frequently performed bilateral procedure is more accurately described as bilateral sequential single-lung transplantation. This procedure has a significantly lower incidence of anastomotic complications than the en bloc double-lung procedure that it replaced, and is technically less difficult to perform than en bloc double lung with simultaneous bronchial artery revascularization. The exposure for bilateral-lung transplantation has traditionally been via bilateral anterolateral thoracotomies through the fourth or fifth intercostal space, connected by a transverse sternotomy—the so-called clamshell incision (Fig. 107-3). The incision provides adequate exposure for mobilization of intrapleural adhesions, even after previous pulmonary resections or pleurodesis, and also provides excellent access for institution of CPB and correction of intracardiac defects. In most patients, the entire incision is made at the beginning of the procedure, and both lungs are completely mobilized. For patients with emphysema who undergo bilateral-lung transplantation, however, the contralateral hemithorax may be left closed until after the first lung graft is implanted; this sequence minimizes the tendency to overinflation of the native lung that may occur during the initial implantation procedure. As mentioned above, a number of centers now prefer bilateral anterior thoracotomy incisions for bilateral-lung transplantation, which provides a superior cosmetic result as well as avoiding the risk of sternal dehiscence or infection. The mobilization and pneumonectomy of the native lung and the implantation of the lung graft are conducted in the same manner as described for single-lung transplantation. Thymic and anterior mediastinal tissue on a superiorly based vascular pedicle may be mobilized for coverage of the bronchial anastomoses. Finally, some centers utilize a median sternotomy approach.
Figure 107-3 Approaches to bilateral lung transplantation. Bilateral anterior thoracotomy is now used commonly. Bilateral thoracosternotomy or “clam shell” incision is an alternative, consisting of bilateral anterior thoracotomy with transverse sternotomy, defined by the line of the inframammary crease. Entrance into the chest cavity is via either the fourth or fifth intercostal space, followed by placement of bilateral rib retractors. Occasionally a midline sternotomy is performed.
Living-related lung transplantation is most commonly performed as a bilateral sequential transplant procedure using the clamshell incision. Cardiopulmonary bypass is instituted electively after the recipient native lungs are mobilized. Each of the donor lobes is implanted at the recipient hilum. Typically, there is little discrepancy in size between the lobar bronchus and pulmonary vein of the donor (usually an adult) and the main bronchus and left atrium of the typical pediatric recipient. The order of the anastomoses (bronchus first) and the technique are the same as for cadaveric single- and bilateral-lung transplantation. Overinflation of the lobar graft is more likely than with a cadaveric allograft and may contribute to postoperative pulmonary edema. A marked size discrepancy between the lobar allograft and the recipient hemithorax is uncommon; if present, the discrepancy should be treated conservatively (e.g., by avoiding chest tube suction rather than by aggressive surgical measures such as thoracoplasty). In all cases, sufficient remodeling of the thorax or hyperinflation of the lobar grafts will occur to obliterate any residual pleural space.
HEART–LUNG TRANSPLANTATION
Either a standard median sternotomy or a clamshell incision may be used for heart–lung transplantation. The latter provides better access for mobilization of intrapleural incisions and is particularly useful for recipients with septic lung disease or prior pulmonary procedures. Following institution of CPB, the lungs are removed by an extrapericardial approach using successive stapling of the bronchovascular structures at the pulmonary hila. The donor right atrium is incised from the inferior vena cava to the right atrial appendage. Inspection is made for the presence of an atrial septal defect and adequate closure of the superior vena cava. The donor bloc is positioned by passing the lungs into the pleural spaces via the retrophrenic pedicles. If a tracheal anastomosis is used, the posterior pericardium is incised between the ascending aorta and superior vena cava to expose the distal trachea and, after the donor and recipient tracheas have been trimmed, a distal tracheal anastomosis is performed. Some centers prefer bilateral bronchial anastomoses at the mediastinal pleural reflection, using the same technique employed for single-lung transplantation. This approach obviates dissection in the posterior mediastinum and may be associated with fewer anastomotic complications. The right atrial anastomosis is completed, followed by the aortic anastomosis. The aortic cross clamp is removed, and after reinflation of the lungs, the heart is deaired via the pulmonary artery and left ventricle. After defibrillation, the patient is weaned from CPB.
POSTOPERATIVE MANAGEMENT
Care of the lung transplant recipient in the immediate postoperative period is multifaceted and includes respiratory and hemodynamic management and initiation of antimicrobial prophylaxis and immunosuppressive agents.
VENTILATION
In most cases, ventilatory management follows standard criteria. The FIO2 is adjusted to maintain a PaO2 greater than 65 mm Hg. Standard volume ventilation is used, and many centers are now using lower tidal volume of 6 mL/kg and PEEP of 5 to 7.5 cm H2O. Transition from volume ventilation to pressure support ventilation is commonly performed once the patient is awake and spontaneously breathing. Appropriate management of postoperative pain is also helpful in weaning patients from the ventilator. Extubation is performed when the patient is awake and responsive to command and the patient has achieved a reasonable rate of ventilation and spontaneous tidal volume, typically 12 to 72 hours after the procedure. Maintaining good bronchopulmonary hygiene, with an early bronchoscopic inspection of the anastomoses and distal airways coupled with physiotherapy, is important in achieving and maintaining extubation in these patients.
Patients with emphysema who undergo single-lung transplantation are an exception to the guidelines mentioned earlier. These patients require particular attention to airway pressures and to the compliance difference between the allograft and the native lung. Hyperinflation of the native lung may not only result in compromise of cardiac filling but also interferes progressively with ventilation of the allograft. Efforts to control hyperinflation of the native lung include use of lower tidal volumes and respiratory rates and higher inspiratory flow rates to allow for more prolonged expiratory times. In rare circumstances, when significant edema has occurred in the allograft, independent lung ventilation using a double-lumen endotracheal tube may be needed.
In patients with significant pulmonary hypertension who undergo lung transplantation, the postoperative pulmonary hemodynamics are unique. In these patients, the right ventricle has been conditioned to generate peak systolic pressures against a markedly elevated PVR. Following lung transplantation, the PVR abruptly decreases to near-normal levels, accompanied by improved ventricular hemodynamics. Minimal catecholamine stimulation occurs when the patient awakens from anesthesia or is weaned from a ventilator, causing the right ventricle to respond by generating peak systolic pressures similar to those that existed preoperatively. The resultant abrupt increase in pulmonary artery pressure, in combination with increased capillary permeability due to ischemia and reperfusion injury and the absence of lymphatic continuity, causes fluid to accumulate rapidly in the donor lung. Typically, this pulmonary edema is very rapid in onset and results in hypoxia that elicits additional increase in pulmonary artery pressure. Preemptive treatment for this condition is necessary and requires maintenance of a high degree of sedation in the first 3 to 5 days after surgery. Following this period, patients can be awakened cautiously and weaned from the ventilator with standard methods while cardiac output, blood gases, and pulmonary artery pressures are closely monitored.
FLUID MANAGEMENT
The goal of fluid management after lung transplantation is to minimize the accumulation of edema fluid in the allograft(s) while maintaining optimal cardiac function. As previously noted, the effects of ischemia, reperfusion injury, and lymphatic discontinuity all contribute to a tendency to develop pulmonary edema in the lung graft. Pulmonary artery pressures and pulmonary capillary wedge pressures need to be kept as low as possible after surgery without compromising ventricular preload. For most patients, a reduction in PVR almost immediately after lung transplantation results in improved right ventricular and, secondarily, left ventricular performance. However, some inotropic support may be required in patients who have pre-existing right ventricular systolic dysfunction. Systolic vascular resistance may be low in the postoperative period, due to a systemic inflammatory response to surgery and to use of epidural analgesics, and this may necessitate the use of pressors to address hypotension.
ANTIMICROBIAL THERAPY
Bacterial prophylaxis entails the use of agents for prophylaxis against gram-positive, gram-negative, and anaerobic organisms in combination with targeted antibiotics to provide appropriate coverage for organisms identified preoperatively from the sputum of the recipient. Recipients who have been hospitalized recently, and therefore exposed to respiratory therapy equipment, or those with cystic fibrosis, require specific antibiotic coverage against Pseudomonas species, based on susceptibility data. Bear in mind that in vitro sensitivity does not always predict the in vivo response to antibiotics, which may show the effect of in vivo synergy and high-concentration gradients within the lung especially for quinolones and macrolides. For cystic fibrosis patients, ongoing surveillance of sputum flora and determination of antibiotic sensitivities are important in the waiting period before transplantation so that an appropriate multidrug antimicrobial regimen can be developed for perioperative use. The addition of antimicrobial inhalation therapy, using either tobramycin or Colistin, can have additive effects in reducing the colonizing load of organisms. Postoperative antibacterial coverage should be modified if pathogens not already covered by the recipient-specific regimen are found in the sputum or bronchoscopic specimens of the donor.
Routine prophylaxis for fungal organisms is useful when preoperative recipient sputum cultures have demonstrated the presence of Aspergillus species at any time before the transplant procedure or when there has been evidence of heavy overgrowth of yeast (e.g., Candida) in the donor sputum culture. In the case of Aspergillus, most units employ prophylactic therapy with the use of either nebulized amphotericin B or azole therapy with voriconazole or itraconazole. Some centers employ a hybrid strategy of initial universal fungal prophylaxis with inhaled therapies followed by preemptive therapy for any organisms detected on surveillance cultures.
The occurrence of herpes simplex virus (HSV) infection, including mucosal ulceration, esophagitis, and pneumonitis, has been virtually eliminated by the routine use of antiviral prophylaxis after lung transplantation but significant infections can still occur and may impact both survival and quality of life.73–75 Valganciclovir used to prevent CMV has cross reactivity for herpes simplex but it is important not to forget to institute prophylaxis in CMV donor-negative/CMV recipient–negative patients who may not be allocated valganciclovir.47,51 In such cases, acyclovir or valacyclovir should be administered for a minimum of 6 months. CMV infection is still a significant problem following lung transplantation.50,74–78 The incidence of CMV infection after lung transplantation is related to the preoperative CMV status of both the donor and the recipient. Recipients with prior exposure to CMV are at risk for reactivation of infection and, if they receive an organ from a CMV-positive donor, for reinfection. The greatest risk occurs when a CMV-negative recipient receives a lung from a CMV-positive donor; the primary infection of the recipient that can ensue tends to be more severe than either reactivation or reinfection. For this reason, some centers prefer to avoid mismatching a CMV-positive donor with a CMV-negative recipient. However, the use of antiviral prophylaxis with ganciclovir or valganciclovir prophylaxis has significantly reduced the incidence of primary disease.
The incidence of Pneumocystis jiroveci infection in lung transplant patients has also been significantly reduced by the routine use of trimethoprim–sulfamethoxazole.79 If this drug is not tolerated alternatives such as dapsone (watch for Coombs-positive hemolytic anemia) or inhaled pentamidine should be entertained.
IMMUNOSUPPRESSION
The induction of a state of relatively nonspecific immune suppression by pharmacologic means has been the key to successful clinical lung transplantation. While the ideal method would be to achieve specific, permanent tolerance of the allograft without the need for chronic medication, this is not possible at present. As a result, although the current regimens lead to satisfactory control of most acute rejection (AR) processes, the combined side effects of these medications and their incomplete ability to control CR in the lung account for the major long-term morbidity and mortality associated with lung transplantation. It is always a balance of efficacy versus toxicity and the transplanted lung has one risk factor that no other solid organ faces, namely it is exposed to the ambient environment with every breath.80,81
The immunosuppressive regimens used for lung transplantation are based on the successful protocols that have evolved for renal and heart transplantation. Recent trials have supported similar regimes in lung transplantation.82–89 Virtually all centers use a three-drug regimen for immunosuppression comprising a CNI, a cell-cycle inhibitor and a corticosteroid, with the hope of obtaining additive effects in terms of immune suppression while limiting drug toxicities. Most lung transplant programs use steroids as part of the regimen for the induction of immunosuppression. However, some centers have used cytolytic therapies such as daclizumab, basiliximab, alemtuzumab, OKT3, and antithymocyte globulin for this purpose. These therapies are typically initiated within 24 hours of transplantation, and are typically combined with the usual triple-drug regimen of steroids, a CNI, and a cell-cycle inhibitor.
Tacrolimus has now overtaken cyclosporine as the mainstay of immunosuppression for lung transplantation and there is a growing evidential base to support this policy.4,89 Tacrolimus (FK-506) is a macrolide compound with a mechanism of action similar to that of cyclosporine mediated through an immunophilin protein called the FK-binding protein (FKBP). Toxicity is similar to that of cyclosporine and includes renal dysfunction, hypertension, and neurotoxicity. New-onset diabetes mellitus has also been reported. In contrast to cyclosporine, hirsuitism and gingival hyperplasia have not been seen with tacrolimus. In a randomized trial in lung-transplant patients of three-drug regimens containing either cyclosporine or tacrolimus, the incidence of postoperative fungal infections was higher in patients receiving tacrolimus. A large 3-year study recently reported a significant reduction in the rate of BOS at 3 years in the arm taking tacrolimus but this was not associated with a difference in 3-year mortality.89
Intravenous or sublingual administration of tacrolimus is usually begun before the graft is implanted and continued postoperatively, provided renal function remains satisfactory. Subsequent conversion to oral dosing is completed when gastrointestinal function is normal. Blood levels of both tacrolimus and cyclosporine correlate with immunosuppressive effects and toxicity but there is still some debate regarding the optimum method of performing therapeutic drug monitoring. For cyclosporine there is good evidence that the best single-point surrogate measure of the area under the time–concentration curve is the value taken at 2 hours post dose.90–93 Less data are available for tacrolimus but it is clear that like cyclosporine, trough levels may both overestimate and underestimate total drug exposure, which may lead to risk of rejection and nephrotoxicity respectively. Nephrotoxicity, the major side effect of both cyclosporine and tacrolimus, results in part from vasoconstriction of the afferent glomerular arteriole and may be ameliorated by the concomitant use of diltiazem. However, chronic CNI toxicity is common and results in granular contracted kidneys with permanent renal failure.
Azathioprine, a purine analog, is converted to several purine metabolites, including 6-mercaptopurine, in red cells and hepatocytes. These purine metabolites have a variety of inhibitory effects on hematologic cell proliferation, with a somewhat greater effect on T cells than B cells. Azathioprine is begun at a dosage of 2 to 2.5 mg/kg per day and adjusted downward to maintain a white blood cell count of more than 4000 cells/mL. The dosage is the same for both the intravenous and oral routes. If necessary, azathioprine may be omitted for several days without significant compromise of its immunosuppressive effect.
Mycophenolate preparations subserve a similar role as cell-cycle inhibitors but despite demonstrable efficacy after heart transplantation in particular, trials after lung transplantation have not shown convincing evidence of superior efficacy or tolerability compared to azathioprine. The largest study to date was an open label study that may have been underpowered by the presentation of 1-year data that was favorable in the mycophenolate arm, perhaps leading to a large number of patients in the azathioprine arm switching to mycophenolate.88 Mycophenolic acid inhibits de novo purine synthesis by inhibiting the conversion of inosine monophosphate to xanthine monophosphate. Since lymphocytes depend almost exclusively on de novo purine synthesis, mycophenolic acid selectively inhibits their replication, including the formation of cytotoxic lymphocytes and both primary and secondary antibody formation. Mycophenolic acid has been shown to reverse AR that is resistant to both corticosteroids and OKT3. Side effects include nausea, gastritis, ileus, and myelosuppression.88
Corticosteroids have a variety of effects on the immune response, mediated by the interaction of the steroid with a high-affinity cytoplasmic receptor. Steroids affect both inflammation and immunity, and modulate lymphocyte-, mononuclear phagocyte–, and antigen-presenting cell functions. Prednisone, prednisolone, and methylprednisolone are all synthetic derivatives of cortisol that are used clinically for transplant patients.94 Intraoperatively, methylprednisolone is administered before reperfusion of the lung graft. Postoperatively, in the absence of cytolytic induction therapy, moderate-dose corticosteroid therapy is used in combination with a CNI and cell-cycle inhibitor for induction immunosuppression. Oral corticosteroids (0.5–1.0 mg/kg/d) are usually begun after the postoperative intravenous steroids and when oral therapies are commenced. Although corticosteroids have profound inhibitory effects on wound healing, their use in this fashion in the immediate postoperative period has not adversely affected the outcomes of lung transplantation.
Various antilymphocyte antibody preparations, the so-called cytolytic therapies, have been used in clinical lung transplantation. Both polyclonal preparations, such as antilymphocyte globulin and antithymocyte globulin (ATG), and a murine monoclonal antibody to the CD3 complex of human lymphocytes (OKT3) have been used. Initially, it was believed that strict avoidance of corticosteroids was needed in the early postoperative period to assure satisfactory healing of the bronchial anastomosis. As a result, cytolytic therapy was thought to be necessary to induce immunosuppression before the initiation of steroid therapy in the second postoperative week. The subsequent demonstration that moderate-dose corticosteroid therapy was well tolerated immediately after lung transplantation, as described, as well as concerns regarding the risks of cytolytic therapy, resulted in many centers reserving the use of these agents for the treatment of refractory AR. However, data from the ISHLT Registry suggest the majority of centers now use some form of cytolytic induction based on the belief that the benefit of a reduced rate of BOS so engendered is worth the risk.4
The two most significant concerns regarding the use of cytolytic therapy have been the increased incidence of CMV disease and of posttransplant lymphoproliferative disorder (PTLD).95–97
The mammalian target of rapamycin inhibitors sirolimus (rapamycin) and everolimus inhibit the response of T lymphocytes to IL-2 and other cytokines but do not inhibit IL-2 production. Sirolimus has been shown to reverse rejection and prolong graft survival in animal models. However, sirolimus has been associated with lethal airway anastomotic dehiscence when used for immunosuppression early posttransplantation.98 Recent reports also warn of an excess rate of venous thrombosis with sirolimus.99 Concurrent cyclosporine administration increases the potency of rapamycin, suggesting that it may be used in combination with cyclosporine to lower the overall toxicity of a multidrug immunosuppressive regimen. Importantly, rapamycin increases the AUC of cyclosporine by more than 150%, which may lead to CNI-induced nephrotoxicity and possibly hemolytic uremic syndrome.100
COMPLICATIONS
Lung transplant recipients are at risk for a number of complications that can adversely impact allograft function as well as post-transplant survival. These include technical, infectious, immune-mediated, and neoplastic complications.
SURGICAL COMPLICATIONS
Major technical complications following lung transplantation have become increasingly rare with improvements in surgical technique and perioperative management. Postoperative hemorrhage requiring exploration is very uncommon with the use of the clamshell incision to improve operative exposure for patients requiring bilateral-lung transplantation or heart lung transplantation. Patients with extensive pleural adhesions from their underlying disease or from prior thoracic surgery or pleurodesis are at greatest risk for intraoperative and postoperative bleeding. Pulmonary artery obstruction can occur as a result of anastomotic stenosis, kinking, or extrinsic compression. In these patients, persistent pulmonary hypertension and unexplained hypoxemia may be evident. Attention to anatomic factors, such as the length of donor and recipient pulmonary arteries and division of the pericardial attachments surrounding the donor pulmonary artery, as well as awareness of the potential for a flap wrapping the bronchial anastomosis to compress the adjacent anastomosis, helps to avoid these problems. Pulmonary venous or left atrial anastomotic obstruction can also occur because of faulty anastomotic technique, kinking upon closure of the chest, or thrombus. This problem results in more severe abnormalities than pulmonary artery obstruction, including marked pulmonary hypertension and ipsilateral pulmonary edema. Diagnostic methods for these vascular anastomotic complications include routine intraoperative measurement of anastomotic gradients and transesophageal echocardiography, which is particularly helpful in assessing the left atrial anastomosis. Postoperatively, diagnostic measures include contrast angiography and ventilation/perfusion scanning. Urgent reoperation and correction of the anastomosis are indicated if clinical compromise is apparent, which is particularly likely if there is significant left atrial anastomotic obstruction. The clinical clue is a diffuse infiltrate within the transplanted lung with evidence of suffusion of the bronchial mucosa at bronchoscopy with or without blood-stained secretions, that may mimic frank pulmonary edema fluid.
About 20% of patients have PGD, characterized by severe early abnormalities of lung function, with rapidly progressive pulmonary edema, persistent pulmonary hypertension, and markedly diminished pulmonary compliance that occurs rapidly after graft implantation (Table 107-7).101 PGD is the most common cause of early mortality after lung transplantation and is associated with long-term sequelae including BOS and increased mortality.101
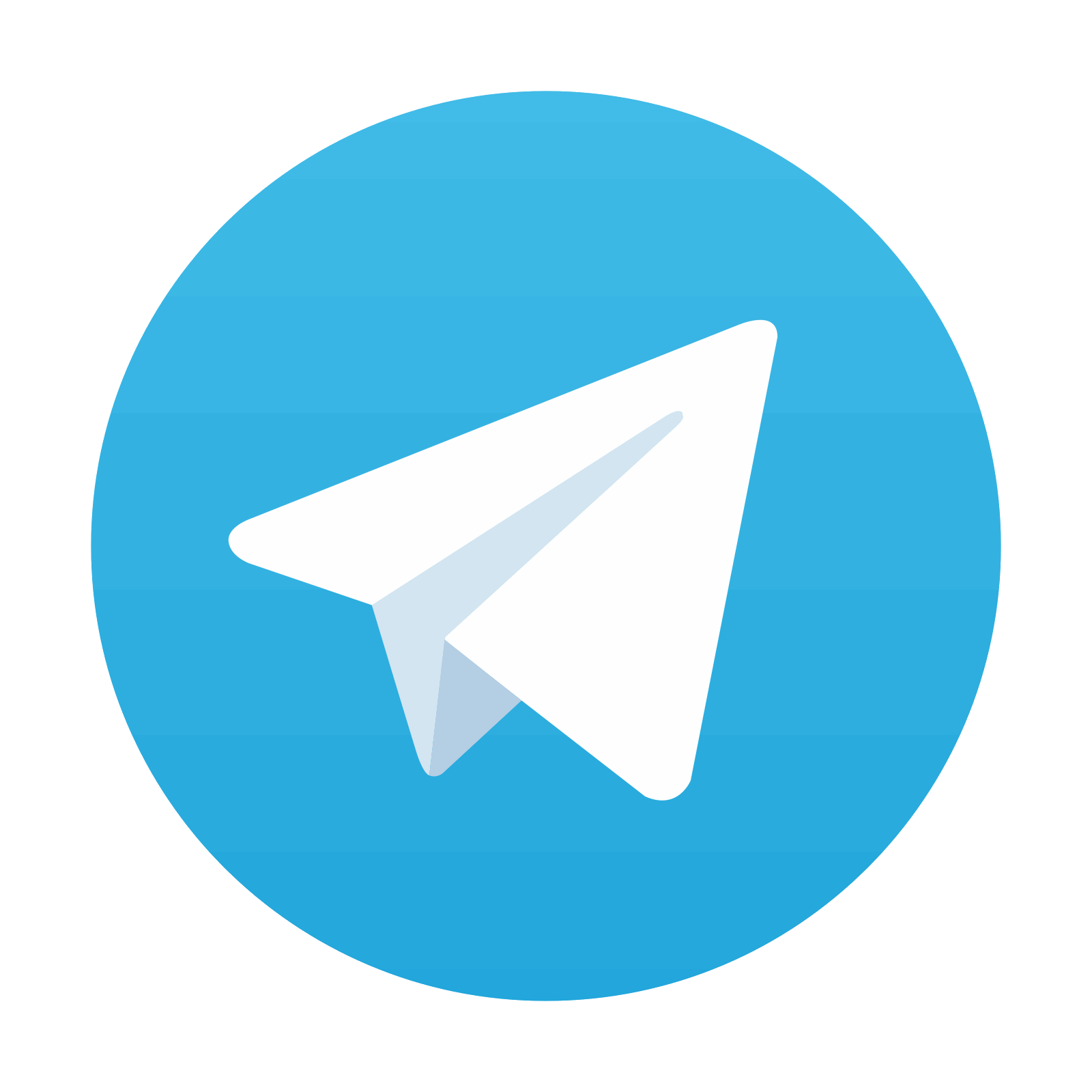
Stay updated, free articles. Join our Telegram channel

Full access? Get Clinical Tree
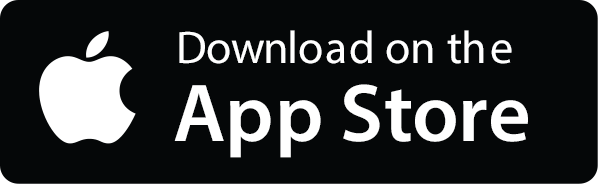
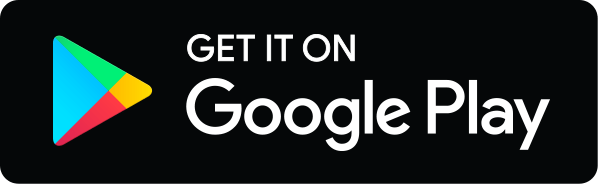