Lung Transplantation
R. Duane Davis
Mathew G. Hartwig
Following the initial report of human lung transplantation by Dr. James Hardy in 1963 at the University of Mississippi, numerous subsequent attempts failed until the first successful transplant by Dr. Joel Cooper at the University of Toronto in 1983. In the succeeding years, lung transplantation has become a viable and effective treatment for patients with end-stage lung disease and has been performed in more than 32,000 recipients worldwide. Progress has been made in surgical techniques, donor lung preservation, lung reperfusion protocols, perioperative care, and long-term medical management of this challenging patient population. These refinements in care are reflected in survival improvements. One-year survival reported by the Organ Procurement and Transplantation Network (OPTN) has improved from 77% in 1997 to 82% in 2007. Likewise, the International Society of Heart and Lung Transplantation (ISHLT) Registry indicated that the median survival increased from 4.0 years if transplanted in 1994, to 5.7 years for a recipient after the turn of the millennium. In fact, the era of a particular transplant procedure remains one of the best predictors of survival in the ISHLT Registry. Similar improvements in survival have occurred in the experience of the Duke Lung Transplant Program (Fig. 23.1A). In the most recent 6-year cohort, our 30-day and 5-year survivals exceeded 97% and 65%, respectively.
Death early after transplant is commonly related to primary graft dysfunction (PGD) that is usually caused by ischemia-reperfusion injury. PGD rates have substantially decreased with better lung preservation and controlled reperfusion strategies. Although posttransplant recipient and allograft survival have significantly improved recently, long-term survival continues to be less than that for other solid organ transplants. Current 5-year survival from the ISHLT Registry approaches 55% for lungs, compared to 73% for cardiac recipients. Late deaths are most frequently related to chronic allograft dysfunction, the causes of which are related to both immunologic and nonimmunologic factors. The control of these factors with better immunosuppressive regimens and with minimizing nonimmunologic injury such as gastroesophageal reflux-related aspiration into the tracheobronchial tree appears to be improving long-term survival.
INDICATIONS FOR LUNG TRANSPLANTATION
Lung transplantation has been successfully applied as a treatment for patients with a number of different end-stage lung diseases. While specific listing criteria and indications have been widely reported, lung transplantation is typically performed in patients (1) with a limited life expectancy secondary to lung failure, estimated to be 6 to 12 months; (2) with the capability of tolerating and following the complicated posttransplant medical regimen; and (3) without comorbidities that would significantly impact posttransplant survival and quality of life, such as concurrent/recent malignancy, extrathoracic infection, or significant other organ dysfunction/failure. In select patients, concomitant other organ transplant or reparative cardiac procedures can be performed with acceptable results.
The choice to perform a single versus a bilateral, sequential lung transplant depends upon multiple factors. Clearly, recipient characteristics such as comorbidities and underlying diagnosis play an important role in directing operative selection. Likewise, the value of increasing the number of recipients transplanted by performing two single-lung transplants (SLTs) from a single donor must be balanced with the benefit of improved long-term survival that is seen in bilateral lung transplants (BLT). While the majority of nonseptic lung disease, such as chronic obstructive pulmonary disorders (COPD), idiopathic pulmonary fibrosis (IPF), and primary pulmonary hypertension, can safely undergo single-lung transplantation, patients with septic lung disease, such as bronchiectasis from either cystic fibrosis (CF) or noncystic causes, require bilateral lung transplantation to prevent infectious complications emanating from the residual native lung. Because of the numerous complications arising from the native lung such as infection, hyperinflation, malignancy, and a 25% absolute difference in survival at 7 years (Fig. 23.1B), the practice of the Duke Lung Transplant Program has been to perform bilateral, sequential lung transplants in the majority of patients. This chapter describes the steps in procuring, preparing, and transplanting lungs in a bilateral, sequential fashion. These techniques are also utilized when only one lung is being transplanted.
DONOR LUNG RETRIEVAL
The improved outcomes after lung transplantation have led to an increased number of recipients waiting for transplant. In the United States, approximately 4,000 patients are listed and waiting for lung transplant. Annually, approximately 1,100 lung transplants are performed while 450 deaths occur on the waiting list. The shortage of organs is not unique as compared to other organs, but there are a number of differences. Of organs commonly transplanted, the lungs are the most sensitive to exogenous damage. Events prior to brain injury, such as smoking, and events associated with brain injury, such as aspiration, mechanical ventilation, or neurogenic pulmonary edema, may compromise the suitability of the donor lungs for transplantation. However, there is a wide variety of opinions regarding what is a suitable lung for transplantation. Throughout the United States, there has been a gradual increase in the percentage of organ donors in which lungs were retrieved to 17%. However, this number is substantially less than the bestperforming regions in the United States as well as in Australia, where the utilization is 40% to 50%. Moreover, survival rates for recipients from these high-utilization
regions exceed registry data. Aggressive donor management with hormone replacement, particularly with respect to corticosteroid administration, appropriate ventilatory settings with alveolar recruitment measures, suitable fluid resuscitation with vasoactive drug administration, aspiration prevention, and utilization of bronchoscopic suctioning have been shown to increase the yields of donor lungs. Importantly, aggressive evaluation and placement strategies by transplant centers are also factors in increasing donor lung yield.
regions exceed registry data. Aggressive donor management with hormone replacement, particularly with respect to corticosteroid administration, appropriate ventilatory settings with alveolar recruitment measures, suitable fluid resuscitation with vasoactive drug administration, aspiration prevention, and utilization of bronchoscopic suctioning have been shown to increase the yields of donor lungs. Importantly, aggressive evaluation and placement strategies by transplant centers are also factors in increasing donor lung yield.
Emerging technologies will also allow for an expanded donor pool and better utilization rates. For example, ex vivo lung perfusion (EVLP) has recently been initiated for human lung transplantation. An initial manuscript detailing 35 lungs transplanted following EVLP suggests equal outcomes in terms of PGD, BOS development, and 1-year survival when compared to 116 control recipients. Currently, this technology allows for rehabilitation of some lung allografts otherwise thought to be unusable. However, in the future it may provide a method for actually improving long-term outcomes through gene therapy or other treatments.
The donor lungs must (1) be ABO compatible with the recipient’s; (2) have no unacceptable HLA antigens if the recipient has anti-HLA antibodies as determined by prospective or virtual cross matching techniques; (3) not transmit diseases such as hepatitis B or C, HIV, or malignancies; (4) be size compatible; and (5) be able to function physiologically to immediately sustain cardiopulmonary function in the recipient. With respect to disease transmission, donors who test as serologic positives for hepatitis B, hepatitis C, or HIV, or have non-CNS, nonskin malignancy (excluding melanoma) are not used except in extraordinary circumstances. One exception is that donors with positive hepatitis core antibody, but negative surface antigen serologies, can be used with very low rates of disease transmission.
Donor evaluation includes the collection of a thorough medical history for the potential donor. Specific donor criteria vary according to individual transplant centers, but in general, the focus is on the donor’s blood type, size, age, smoking habits, pulmonary diseases, thoracic procedures, and mode of death. Additional information that should be provided at the time of a donor offer includes radiographic findings, arterial blood gas, ventilator settings, peak airway pressures, endotracheal tube size, and bronchoscopic findings. In an older donor with a substantial smoking history, a high-resolution chest CT can greatly assist in evaluating the lung parenchyma and allow for a better assessment of the presence of emphysema, lung nodules, and interstitial lung disease that would be contraindications to the use of the lungs. Corticosteroids (methylprednisolone 1,000 mg) should be administered to the donor and ventilatory settings should be optimized. Underventilation and inappropriate use of positive end-expiratory pressure (PEEP) is common and optimization can improve the donor arterial blood gas results. The most recent chest radiograph should be examined for pathology and can be used to help with sizing of the organs. Atelectasis does not preclude transplantation; however, clear consolidation would prevent the use of the organs, unless the area could be easily excised or the contralateral lung was to be utilized. This differentiation is rarely possible without direct visualization and palpation of the organs. Atelectasis is the most common reversible cause of low PaO2/FiO2 ratios in the donor. On-site evaluation of prospective donors greatly increases the number of lungs retrieved. In the absence of poor airway compliance (elevated peak airway pressure in the absence of a small endotracheal tube or morbid obesity) or combined abnormalities such as an older donor with extensive tobacco exposure and marginal arterial blood gases, we attempt to evaluate all donors.
Size matching of the donor and the recipient is initially based on height. Matching within 4 inches between donor and recipient is usually without consequence. However, recipients with obstructive lung disease will have larger than normal thoracic cavities, and larger donors are preferred. Recipients with restrictive lung disease, on the other hand, will have smaller than normal thoracic cavities, and smaller donors are preferred. Comparison of chest measurements, both vertical and horizontal dimensions, between donor and recipient from the chest X-ray provides additional information regarding sizing. It is important to account for size reformatting with digitized films. While significant size differences between donor lung size and recipient thoracic volume can be managed successfully in the operating room through the use of pneumoreduction techniques, appropriate size matching prior to committing to the transplant procedure is optimal.
Fiberoptic bronchoscopy is performed to evaluate for evidence of airway inflammation, intraluminal pathology, anatomic abnormalities, as well as to remove secretions from the airways. While it is common to encounter some purulent secretions,
this is not a contraindication to organ utilization unless the secretions do not clear or they recur after being suctioned clear. Donor airway cultures and gram stains are important for determining recipient antibiotic administration, but should not obviate the utility of lungs from donors.
this is not a contraindication to organ utilization unless the secretions do not clear or they recur after being suctioned clear. Donor airway cultures and gram stains are important for determining recipient antibiotic administration, but should not obviate the utility of lungs from donors.
Donor lung procurement is usually performed in the context of cardiac and hepatic organ procurement. The following donor procurement procedure can be utilized in all circumstances. Exposure of the thoracic and abdominal organs is through a median sternotomy with an extension of the incision in the midline to the pubis. The pericardium is opened in the midline with wide extensions laterally at the level of the diaphragm inferiorly. Pericardial stay sutures are placed for exposure. Both pleural spaces are opened widely, extending from the diaphragm inferiorly to the mammary pedicles superiorly. Adhesions are divided with the electrocautery. Manual examination of both lungs is performed serially to assess the general appearance, nodules, atelectasis, consolidation, edema, and compliance. Suspicious findings should be excised and sent for pathologic analysis to rule out malignancy. The elastic recoil of the lungs is then determined by inflating the lungs with bagged breaths and allowing the lungs to deflate independently by temporarily disconnecting the endotracheal tube from the ventilator. Lungs with adequate compliance should rapidly deflate following disconnection from the ventilator. During this phase of the evaluation, an aggressive alveolar recruitment is performed. Working with the anesthesiologist, the lungs are hand ventilated to expand any atelectatic areas. An arterial blood gas is obtained following these maneuvers. We use a minimum PaO2 of 300 mmHg with the FiO2 of 1.0 and PEEP of 5 cm H2O as criteria for suitability. Additional information can be obtained and nonpulmonary shunt causes of impaired oxygenation can be identified by individually sampling from the four pulmonary veins. The expected PaO2 should be greater than 400 mmHg from the veins draining appropriately functioning regions. This approach can identify areas of lung inappropriate for transplantation. Not infrequently, contused segments or areas of consolidation can be resected by wedge resection, lobectomy, or the use of only one lung. These resections are ideally done as a “back table” procedure after the procurement. Similarly, when the donor lung is oversized for the recipient chest cavity, lung resection can be performed, again, preferably as a back table procedure. In these situations, our preference is to perform a middle lobectomy on the right and a lingulectomy on the left, with a curvilinear wedge resection from the anteroapical section of either upper lobe. When even greater oversizing occurs, implanting only the lower lobe may be optimal.
Once the decision that the donor lung(s) is suitable for procurement is confirmed and this is communicated to the recipient surgical team, preparation for procurement begins by mobilizing the great vessels. Using primarily cautery dissection, the ascending aorta is dissected from the pulmonary artery (PA) and the superior vena cava (SVC). The SVC is mobilized by cautery dissection from the innominate vein to the right atrial juncture. This dissection is continued on to the heart to develop Waterston (interatrial) groove. By fully mobilizing the SVC and adequately developing the interatrial groove, the two most common sites of procurement injury can be minimized: (1) injury to the right PA posterior to the SVC often at the level of the right PA trunk bifurcation, and (2) an inadequate anterior right-sided left atrial cuff. The inferior vena cava (IVC) can usually be mobilized quickly with finger dissection. Commonly, temporary hemodynamic instability occurs, but can be lessened by placing the donor in Trendelenburg position prior to these maneuvers. The IVC should be well exposed below and above the diaphragm to ensure adequate tissue for abdominal and cardiac transplant teams.
The trachea is located by incising the posterior pericardium after gently retracting the SVC laterally and the aorta medially. An umbilical tape can be used to encircle the trachea after the plane is developed manually. Once all procuring teams are ready to cannulate, the donor is fully heparinized (250 units/kg). The ascending aorta is cannulated for standard antegrade cardioplegia. The cannula is secured using a 4-0 Prolene mattress or purse-string suture and a Rummel tourniquet. Placement of the PA cannula occurs in a similar fashion as the aortic cannula with placement comfortably distal to the pulmonary valve, but adequately proximal to assure equal perfusion through both pulmonary arteries. Care should be taken to ensure that the bent tip of the curved cannula is directed toward the bifurcation of the PA. After placement of the cannulae, a 500-µg bolus dose of prostaglandin E1 (prostacyclin) is administered directly into the pulmonary trunk, near the pulmonary cannula. Immediately following infusion of the prostacyclin, the SVC is ligated doubly or occluded using a vascular clamp and the IVC is divided allowing decompression of the right heart. The IVC should be divided above the pericardial reflection to provide adequate cuff for both the liver and heart implantations. The aorta is cross-clamped and the cardioplegia solution is infused through the aortic cannula. Even if the heart is not to be procured, aortic cross-clamping should be performed to prevent bronchial artery flow. Left heart decompression is achieved by performing a large left atrial appendage incision. Once this incision is created, perfusion of the lungs through the PA catheter is initiated with either Perfadex® or Celsior® preservation solutions (extracellular-based solutions). The height of the solution bag above the PA cannula should not be more than 30 inches. Approximately 3 L of perfusate (35 to 50 ml/kg) are flushed through the PA (antegrade flushing of the lungs). Upon commencing perfusate flow, the thoracic cavity should be bathed in iced saline solution. The procuring surgeon then assesses biventricular filling by directly palpating the heart. Exsanguination and subsequent flow of clear fluid from the left atrial appendage should be observed, as it usually indicates successful antegrade flushing of the preservation solution throughout the pulmonary vasculature. Ventilation of the donor lungs should continue during preservation solution administration. To optimize visibility for the subsequent organ removal, the abdominal IVC should be vented inferiorly and not into the chest.
Following adequate infusion of the appropriate preservation solutions into the aorta and the PA, removal of the heart is performed. It is paramount that adequate tissue for implanting be achieved for both the heart and lung allografts. The heart is retracted to the right and a left atriotomy is initiated midway between the coronary sinus and the left pulmonary veins. The interatrial groove on the right is further developed to ensure adequate atrial cuff size. The initial atriotomy is extended inferiorly and superiorly using scissors, while being mindful of the left pulmonary veins. The surgeon on the left side of the table typically has the optimal view of the right pulmonary vein orifices and should finish creating the atrial cuff while directly visualizing these orifices. Enough cuff width should be available for the left atrial anastomosis. The SVC is divided between the silk ligatures, or by using a vascular clamp one can maximize the length of SVC taken by repositioning the clamp distally at this time. The main PA is transected at the bifurcation and the aorta is divided proximal to the cross-clamp. As with the SVC,
the vascular clamp may be moved distally in order to maximize the length of the aorta. The heart is removed from the field.
the vascular clamp may be moved distally in order to maximize the length of the aorta. The heart is removed from the field.
After the heart is passed off the table, retrograde flushing of the lungs is performed through each large pulmonary vein orifice. Adequate retrograde perfusion can be determined when the perfusate eluting from the PA becomes clear. Usually this requires approximately 1 to 2 L of the preservation solution (250 to 500 ml of solution for each of the four pulmonary veins). Removal of the remaining thoracic contents ensues by dividing the inferior pulmonary ligaments and posterior attachments. The trachea is mobilized by blunt dissection to at least three cartilaginous rings proximal to the carina. Prior to stapling the trachea, the anesthesia team ventilates the lungs with several bagged breaths. The endotracheal tube is pulled proximally, the lungs are allowed to deflate to approximately two-thirds of vital capacity, and the trachea is stapled with the TA-30 stapler two to three rings above the carina. A second row of staples is immediately placed proximally and the trachea is divided between the two staple lines with a scalpel or scissors. Overexpansion of the lungs is avoided to prevent alveolar stretch injury and subsequent allograft failure.
Heavy scissors can now be used to swiftly divide the remaining posterior mediastinal tissue inferiorly and superiorly in the plane anterior to the esophagus, completely freeing the lungs for removal. Alternatively, the esophagus can be divided after removal of the nasogastric tube proximally and distally with a GIA stapler. The remaining dissection then occurs between the esophagus and the spine with subsequent removal of the lungs en bloc with the esophagus. At this point, they can be bagged together for travel; if the right and the left lungs are going to separate institutions, they require division at the procurement hospital. Our preference is to perform back table dissection at the implanting hospital when possible because of better lighting and equipment.
The lungs are divided by incising the posterior pericardium, the left atrium midway between the two sets of veins, and the main PA at its bifurcation (Fig. 23.2). When lungs are traveling to separate institutions, the left bronchus can be transected between staples just distal to the carina to maintain its inflation pressure for transport. Upon arrival at the transplanting institution, the grafts are prepared for implantation. This primarily involves dividing the main bronchus for each lung either one or two rings proximal to the upper lobe takeoff. Because of the reliance of the bronchus and airways on collateral perfusion from the PA, the length of the bronchus is kept to a minimum. Care should be taken to minimize dissection along the length of the bronchus. The pulmonary arteries are inspected for the presence of pulmonary emboli. If present, they are removed with forceps and retrograde flushing through the pulmonary veins is repeated until the perfusate clears. All structures are inspected for injuries. Reconstruction of vascular injuries or inadequate cuff lengths is best achieved on the back table before initiating implantation. Direct repair is usually possible, but autologous tissue from the explanted lungs, allograft tissue from the donor, and pericardium from the recipient can be used to repair injuries. The arteries and atrial cuff should also be evaluated for residual pericardial attachments, and these should be removed at this time. If retained they may lead to kinking and blood flow occlusion after the anastomosis is completed. Samples of donor bronchus for microbiologic testing can also be taken at this time.
RECIPIENT PROCEDURE
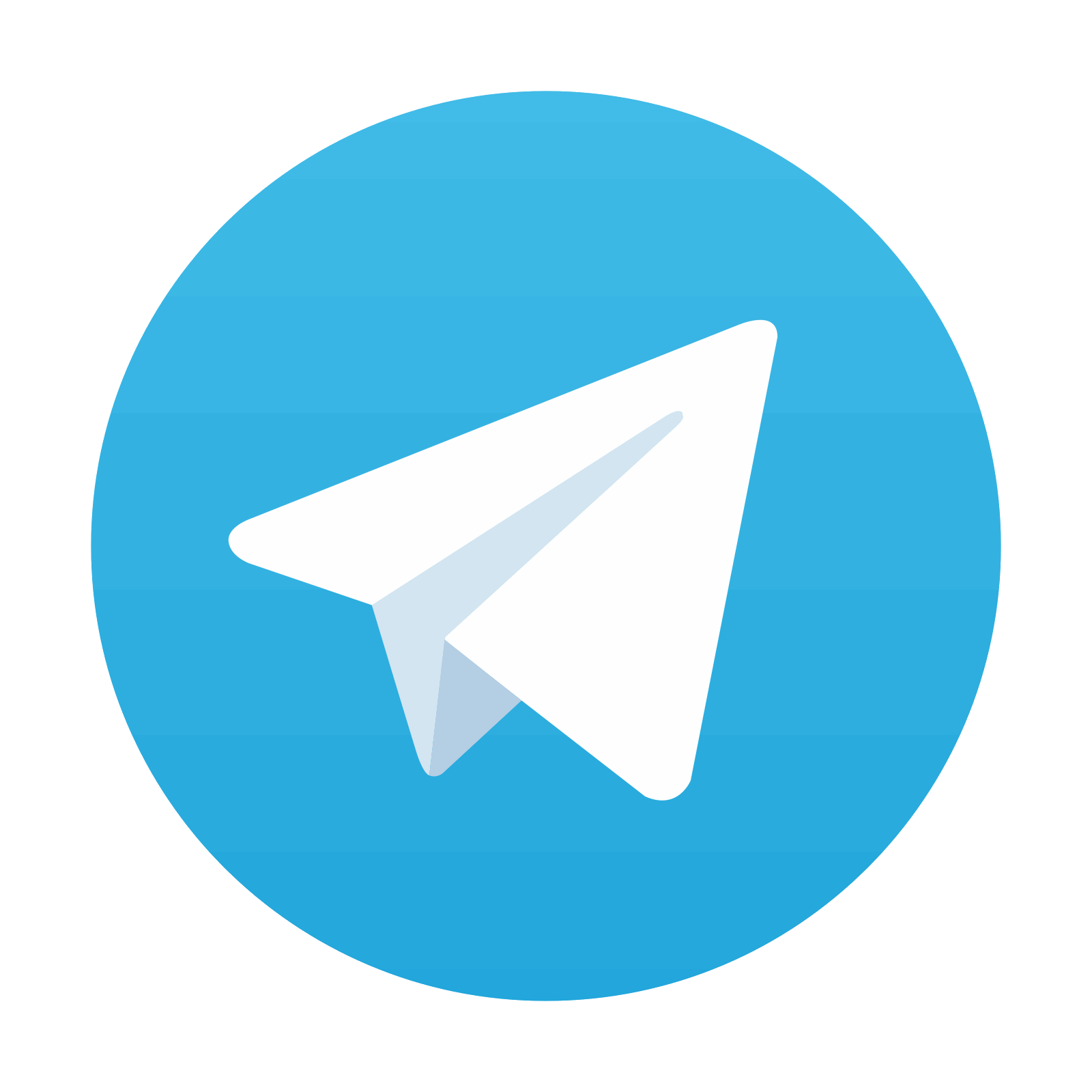
Stay updated, free articles. Join our Telegram channel

Full access? Get Clinical Tree
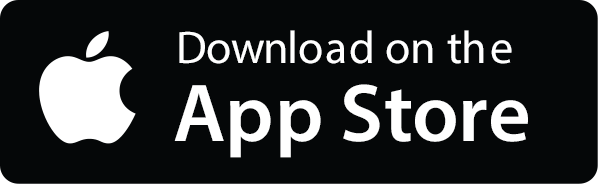
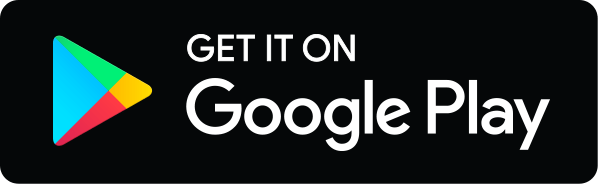
