Long-Term Anticoagulation for Cardiac Conditions
Richard D. Taylor, MD
Richard W. Asinger, MD
General Considerations
Intracardiac thrombi can form and lead to devastating consequences as a result of obstruction of blood flow and/or peripheral embolization. Long-term anticoagulation is recommended for primary and secondary prevention of thromboembolism in many cardiac conditions, and in selected conditions, it is essential. There are, however, risks associated with the use of anticoagulants, and an understanding of the risks and benefits of anticoagulant therapy for various cardiac conditions is important.
A. Anticoagulants
These agents affect the coagulation protein cascade to reduce thrombosis.
1. Unfractionated heparin—Unfractionated heparin (UFH) binds to antithrombin III, markedly increasing the effect of antithrombin III in neutralizing thrombin. It also inhibits the activation of factors IX and X. The effectiveness of UFH varies greatly from person to person due to its interactions with a number of plasma proteins and the endothelium. Monitoring the effects of full-dose UFH on hemostasis is mandatory. The activated partial thromboplastin time (aPTT) is used to monitor the effects of UFH and is titrated to 1.5–2.0 times greater than control. In certain situations, a higher level of anticoagulation is needed; for example, during coronary interventions where the activated clotting time (ACT) is used to monitor its effect and the dose of UFH is adjusted to keep the ACT 250–300 seconds or greater. When given intravenously, the effects of UFH are immediate. It is usually given as a bolus, followed by a continuous intravenous infusion. It may also be given in therapeutic doses subcutaneously. The effects of UFH will dissipate within 6 hours. Protamine can be given to reverse its effects more quickly. UFH can also be given subcutaneously in smaller doses, which will not affect the aPTT, for primary prevention of deep venous thrombosis.
2. Low-molecular-weight heparin—Low-molecular-weight heparins (LMWHs) are breakdown products of UFH. They have a greater effect on factor X than on thrombin. LMWHs bind less to plasma proteins than UFH, and therefore, the dosing is more predictable. They are more resistant to neutralization by platelet factor 4 than UFH and have less inhibitory effect on platelet function than UFH. LMWHs have a more predictable effect on coagulation than UFH, and laboratory monitoring is usually not necessary, but activated factor Xa levels can be used to monitor their effects. LMWHs are usually administered subcutaneously twice daily. They are not easily reversed by protamine. LMWH can also be given in smaller doses for the primary prevention of deep venous thrombosis.
3. Intravenous thrombin inhibitors—Lepirudin, bivalirudin, and argatroban inhibit thrombin formation and are useful when UFH and LMWH are contraindicated. These agents are administered by bolus followed by a continuous infusion. These agents inactivate both free and fibrin-bound thrombin. They do not bind to plasma proteins and, therefore, have a more predictable pharmacologic response, but their therapeutic window is narrow. The aPTT is used to monitor their effect. The international normalized ratio (INR) is also affected by these agents, making transition to warfarin more difficult. The bleeding risk with the direct thrombin inhibitors may be greater than with UFH. Due to the short half-life of these drugs, bleeding complications can usually be controlled by stopping the infusion. Argatroban is the preferred thrombin inhibitor for patients with renal insufficiency.
4. Fondaparinux—This agent is an analog of pentasaccharides found in UFH and LMWH. It increases the effect of antithrombin and inhibits factor Xa. It has a half-life of 17 hours when given subcutaneously and is given once daily according to the patient’s weight: 7.5 mg for 50–100 kg, 5 mg for < 50 kg, and 10 mg for > 100 kg. It should not be used if the creatinine clearance (CrCl) is less than 30 cc/min. It is used for primary prevention of deep vein thrombosis (DVT) and for treatment of DVT and pulmonary embolus.
5. Oral vitamin K antagonists—Coumarins, or vitamin K antagonists, have been the mainstay of oral anticoagulants for more than 50 years. Warfarin is the most commonly used oral anticoagulant. It blocks the conversion of vitamin K to the active moiety, vitamin K epoxide, which is necessary for the synthesis of factors II, VII, IX, and X and proteins C and S. The half-life of warfarin is about 40 hours. It is nearly completely absorbed when given orally and binds to albumin. Its effect on hemostasis is quite variable from person to person and, sometimes, for the same person at different times. Warfarin’s effect can be influenced by dietary factors, liver disease, congestive heart failure, hypermetabolic states, and numerous drug interactions. Monitoring the anticoagulant effect of warfarin with a prothrombin time (PT) is mandatory. The PT is now standardized to an international thromboplastin reagent and reported as the INR. Serial INRs are used to monitor warfarin effects and adjust its dose. Warfarin therapy may be started without a loading dose. It usually takes several days for the target INR to be achieved. During initiation of therapy, there may be a brief paradoxical hypercoagulable state due to the inhibition of proteins C and S (anticoagulant factors) before the inhibition of the coagulant factors. For this reason, UFH or LMWH may be used as a bridge until a therapeutic INR is achieved. During initiation of warfarin therapy, the INR should be checked frequently until a stable dose is found that achieves the target INR. Subsequently, the INR should be monitored at least once a month and more frequently if the dose needs to be adjusted. Monitoring can be done by an individual care provider or through an anticoagulation clinic, which tends to be more organized and efficient. Point-of-care monitoring of INR is now available and can be done at home. If warfarin needs to be stopped, the INR will normalize in about 3–4 days. If its anticoagulant effect needs to be reversed more quickly, vitamin K can be administered orally (preferred), subcutaneously or intravenously. The INR will usually correct in 1–2 days if vitamin K is given. If it is necessary to reverse the effects of warfarin more quickly, fresh frozen plasma or prothrombin complex concentrate can be given.
6. New oral anticoagulants—Recently, several new oral anticoagulants have been developed that, in distinction to warfarin, target only a single enzyme in the coagulation cascade. These have targeted thrombin and activated factor Xa. They can be given in a fixed dose that does not require routine monitoring.
A. DIRECT THROMBIN INHIBITORS—These inhibit free thrombin and also thrombin bound to fibrin. Two of these inhibitors, ximelagatran and dabigatran, have been evaluated in phase III clinical trials of nonvalvular atrial fibrillation patients comparing the drug with dose-adjusted warfarin for stroke and systemic embolus as well as bleeding complications. Ximelagatran was associated with liver enzyme elevation and is no longer used. Dabigatran has been evaluated in a phase III clinical trial in two doses. At 150 mg orally twice a day (bid), dabigatran was superior to warfarin in stroke prevention with a similar incidence of major bleeds. At 110 mg orally bid, dabigatran had a similar incidence of stroke with fewer major bleeds compared to dose-adjusted warfarin. It is excreted primarily through the kidneys and is recommended at 150 mg bid for those with CrCl > 30 cc/min. Based on pharmacodynamics data, a reduced dose of 75 mg bid has been approved by the U.S. Food and Drug Administration for those with CrCl of 15–30 cc/min, although there is no clinical evidence of efficacy. It is not recommended for those with a CrCl < 15 cc/min. The higher dose of 150 mg bid was associated with increased bleeds in those over 75 years of age, and the 110 mg bid dose is recommended in those of age 80 or older by Health Canada. The dose should also be reduced to 110 mg bid for those who weigh < 60 kg. Dabigatran is not metabolized by the cytochrome P450 system, but P-glycoprotein inhibitors can increase its concentration. The European Society of Cardiology (ESC) recommends the lower dose of 110 mg bid for those on verapamil and does not recommended it for those taking dronedarone. Potent P-glycoprotein inhibitors, including St. John’s wort, rifampin, carbamazepine, and phenytoin, reduce dabigatran concentration, and it should not be given in combination with these agents. Measurement of the anticoagulant effect is not readily available clinically, although the aPTT is affected. No specific therapy reverses its effect, although hemodialysis will reduce its concentration. In accidental overdose, activated charcoal may be used to absorb it from the stomach. The use of prothrombin complex concentrate and/or activated factor VII maybe helpful in cases of uncontrolled bleeding, but there are no clinical data establishing the efficacy of this approach.
B. FACTOR XA INHIBITORS—A number of factor Xa inhibitors have been or are being developed. Two have been reported in randomized, phase III clinical trials of the incidence of stroke and major bleeds in patients with nonvalvular atrial fibrillation comparing the new anticoagulant with dose-adjusted warfarin (target INR 2.0–3.0). Both rivaroxaban and apixaban are highly selective, reversible, direct factor Xa inhibitors.
Rivaroxaban is a once-a-day drug that is excreted primarily through the kidneys. It is not recommended in patients with a CrCl < 15 cc/min, and there are few data on its efficacy in those with CrCl of 15–30 cc/min. Although drug and diet interactions are minimal, it is metabolized in the liver through cytochrome P450 (CYP)–dependent and –independent mechanisms. It is not recommended for those receiving inhibitors of both CYP3A4 and P-glycoprotein, including azole antimycotics and human immunodeficiency virus (HIV) protease inhibitors. The anticoagulant effect of this drug is not easily measured clinically, although its effect is reflected in the INR. No specific agent is available to reverse its effect. Accidental overdose may be treated with activated charcoal to absorb the drug from the stomach. Hemodialysis is not effective in reducing its concentration because it is highly protein bound. Its anticoagulant effect can be reversed in vitro with prothrombin complex concentrate, but this has not been demonstrated clinically.
Apixaban is a twice-a-day drug that is metabolized in the liver by CYP3A4-dependent and -independent mechanisms, and about one-quarter is excreted in the urine. It is not recommended for patients with a CrCl < 15 cc/min or in those receiving HIV protease inhibitors. Also there is no easy way to monitor the anticoagulant effect of apixaban clinically, and there is no specific reversal agent. Accidental overdose may benefit from activated charcoal to absorb the drug from the stomach. The use of prothrombin complex concentrate and/or factor VIIa may be helpful for uncontrolled bleeding, but clinical evidence for the efficacy of this approach is limited.
Two other factor Xa inhibitors are in development. Edoxaban is currently being evaluated in a large phase III clinical trial of nonvalvular atrial fibrillation compared with dose-adjusted warfarin. Another factor Xa inhibitor, betrixaban, is also being developed.
B. Risks of Anticoagulant Therapy
Bleeding is the most common serious adverse effect seen with the use of anticoagulant agents. Bleeding complications are more likely to occur in patients who are: of age >65 years; have a history of previous stroke, gastrointestinal tract bleeding, recent myocardial infarction, anemia, renal insufficiency, serious concurrent illness or diabetes; drink alcohol excessively; take antiplatelet agents including aspirin (Table 4–1). Intracranial hemorrhage (ICH) is perhaps the most feared complication of anticoagulant therapy. In the absence of anticoagulation, ICH usually presents suddenly, with rapid progression to the maximal neurologic deficit. ICH that occurs while a patient is receiving anticoagulant therapy, however, can be much more devastating, with continued bleeding and progressive neurologic deterioration. In such situations, reversal of the anticoagulant effect is necessary as soon as possible. Of the anticoagulants available, the incidence of ICH in patients with nonvalvular atrial fibrillation is highest in those prescribed warfarin. For those taking dabigatran or factor Xa inhibitors, the incidence of ICH is significantly less, only one-third the incidence seen with dose-adjusted warfarin. The anticoagulant effect of these newer agents, however, cannot be easily measured clinically, and more problematic is the fact that they have no specific antidote.
Table 4–1. Risk Factors for Bleeding Complications with Anticoagulants
Thrombocytopenia can complicate the use of UFH and LMWH in two ways. A dose-dependent lowering of the platelet count is usually not serious and does not necessarily require stopping these agents. The second type of thrombocytopenia is termed “heparin-induced thrombocytopenia” (HIT). It is immune-mediated and can be life-threatening due to arterial and venous thrombus formation. An antibody to heparin interacts with a heparin–platelet factor 4 complex on the platelet surface, resulting in platelet destruction. HIT usually starts at least 4 days after heparin initiation unless there has been previous heparin exposure. It is not dose-dependent, and there is usually at least a 50% reduction in the platelet count. The diagnosis of HIT can be confirmed with an immunoassay for the antibody–heparin–platelet factor 4 complex. HIT is much less common with LMWH than with UFH, occurring about one-tenth as frequently. The treatment for HIT is immediate discontinuation of all heparin, even low doses used to maintain intravenous access. A direct thrombin inhibitor, such as lepirudin, bivalirudin, or argatroban, should be substituted.
Additional rare complications seen with UFH and LMWH include hyperkalemia (due to hyperaldosteronism), osteoporosis, skin necrosis, and alopecia.
Adverse effects seen with warfarin include skin necrosis and teratogenic effects. Skin necrosis due to warfarin is a rare complication seen within the first few days of treatment. It is most likely to occur in patients with protein C and S deficiencies. Because of its teratogenic effects, warfarin should be used cautiously during pregnancy (if at all), especially in the first trimester and specifically between the 6th and 12th weeks of gestation.
De Caterina R, et al. New oral anticoagulants in atrial fibrillation and acute coronary syndromes: ESC Working Group on Thrombosis-Task force on Anticoagulants in Heart Disease position paper. J Am Coll Cardiol. 2012;59:1413–25. [PMID: 22497820]
Furie KL, et al. Oral antithrombotic agents for the prevention of stroke in nonvalvular atrial fibrillation: a science advisory for healthcare professionals from the American Heart Association and American Stroke Association. Stroke. 2012;43:3442-53. [PMID: 22858728]
Hirsh J, et al. American Heart Association/American College of Cardiology Foundation guide to warfarin therapy. J Am Coll Cardiol. 2003;41(9):1633–52. [PMID: 12742309]
Levine MN, et al. Hemorrhagic complications of anticoagulant treatment. Chest. 2001;119(1 Suppl):108S–121S. [PMID: 11157645]
Pathophysiology & Etiology
A. Pathogenesis of Intravascular Thrombi
In the nineteenth century, Virchow theorized that stasis of intracavitary blood, endocardial injury, and a hypercoagulable state were necessary for the formation of intracardiac thrombi. In the normally functioning heart, stasis and thrombosis do not occur, but a high incidence of thrombosis is noted in cardiac chambers that are enlarged or have low flow. Left atrial thrombi can develop in persons with atrial fibrillation and left ventricular thrombi in those with acute myocardial infarction, left ventricular aneurysm, and dilated cardiomyopathy. In these clinical settings, there is generalized or localized low flow in the left atrium and left ventricle, respectively.
Acute ST segment elevation myocardial infarction (STEMI) causes stasis of intracavitary blood (secondary to dysfunction of part of the left ventricle) in the setting of a hypercoagulable state, and left ventricular thrombi are common, particularly if reperfusion therapy is not given or is ineffective. Left ventricular thrombi most commonly develop at the apex of the left ventricle 2–7 days after anterior infarction; they rarely develop with inferior STEMI or non–ST segment elevation myocardial infarction (NSTEMI). Because the left anterior descending coronary artery usually supplies the apex, apical stasis may occur with anterior infarction. Severe apical wall motion abnormalities (ie, akinesia and dyskinesia) precede thrombus formation. Endocardial injury in acute infarction also contributes to thrombosis.
Endocardial abnormalities may be present in other clinical settings such as atrial fibrillation, in which elevated von Willebrand factor is noted in the left atrium. The introduction of a foreign material, such as a prosthetic valve, can provide a nidus for thrombus formation. An inflammatory process that involves the myocardium, secondary to myocarditis or noninfectious endocarditis, may be responsible for thrombus formation, particularly when there is a coexistent hypercoagulable state. Reduced ventricular function may contribute to thrombosis with myocarditis. Eosinophils presumably cause the endothelial injury with Löffler endocarditis that leads to thrombus formation.
The final prerequisite for intracardiac thrombosis is a hypercoagulable state. Activation of the coagulation system can be found in conditions associated with intracardiac thrombi, including acute myocardial infarction and atrial fibrillation. Rare cases of intracardiac thrombi have been reported even with normal wall motion and presumably normal blood flow because of a hypercoagulable state such as factor V Leiden mutation, prothrombin gene mutation, anti-thrombin deficiency, protein C and S deficiencies, homocysteine deficiency, and antiphospholipid antibody syndrome. Platelets may also play an active role in the formation of intracardiac thrombi and are activated in such clinical situations as acute myocardial infarction and atrial fibrillation.
Although all three of Virchow’s prerequisites are operative in specific settings, most clinical data indicate a complex interaction exists, with stasis being the most frequent (or perhaps the most easily demonstrated) factor leading to intracardiac thrombosis.
B. Embolization of Thrombi
The major risk associated with intracardiac thrombi is end organ ischemia/infarction due to embolization. Factors that result in embolization of intracardiac thrombi are poorly understood, but restoration of mechanical function to an area of the heart that was previously noncontractile may result in embolization. Left ventricular thrombi usually form within the first week of an anterior myocardial infarction. However, embolization due to these thrombi usually occurs 5–21 days following infarction, a time at which left ventricular function may be improved, eliminating stasis and expelling the thrombus. Improved myocardial function at the margins of a thrombus could theoretically dislodge the thrombus or change the shape of a thrombus from mural to protruding and lead to embolization.
Recovery of the mechanical function of the left atrium following conversion from atrial flutter or fibrillation has also been implicated in development and embolization of left atrial thrombi. Following conversion of atrial fibrillation to sinus rhythm, the left atrium and/or appendage may be stunned, creating a low-flow state that leads to thrombus formation followed by embolization as its function recovers. Endogenous or pharmacologic thrombolysis could also result in disruption of thrombi and embolization. Embolization is more likely for certain intracardiac thrombi, especially protruding or mobile thrombi. Embolization may also be more likely when larger thrombi are present. Although all the factors that lead to embolization are not known, it is known that embolization is likely to recur in patients who have had previous embolization. Peripheral embolization of intracardiac thrombi can be to the brain, extremities, bowel, spleen, and kidneys. The most frequent site recognized clinically is to the brain, resulting in stroke.
The differentiation of thrombotic from embolic stroke is not always possible, although it is estimated that 15% of all ischemic strokes are cardioembolic. Patients with atrial fibrillation, prosthetic heart valves, or left ventricular mural thrombi are more likely to have cardioembolic events, whereas patients with known carotid artery disease are more likely to have a thrombotic stroke or an artery-to-artery embolus.
Patients who suffer a cardioembolic stroke are at risk for recurrent stroke. Antithrombotic therapy can lower the risk of recurrent stroke, but a serious and potentially life-threatening complication of cardioembolic stroke is intracerebral bleeding secondary to transformation of an ischemic to a hemorrhagic stroke. Computed tomography (CT) scanning should be performed in patients with a cerebral embolus to identify hemorrhage or if there are features indicating a high risk of hemorrhagic transformation. The Cerebral Embolism Study Group recommends that patients with cardiogenic cerebral emboli receive anticoagulation if they are not hypertensive and there is no evidence of intra-cerebral hemorrhage on CT scan at 24–48 hours. A delay of 7 days might be prudent in those with large cerebral infarction to lower the risk of hemorrhagic transformation.
Diagnostic Studies
An intracardiac source of embolization is often suspected clinically, and a number of diagnostic tests can be used to identify intracardiac thrombi.
Echocardiography is a readily available and reasonably accurate, noninvasive technique for detecting intracardiac thrombi. Transthoracic echocardiography (TTE) is sensitive and specific for detecting left ventricular thrombi (Figures 4–1 and 4–2). Transesophageal echocardiography (TEE) is required to reliably detect left atrial thrombi (Figure 4–3) and atherothrombotic material in the ascending, transverse, and descending thoracic aorta (Figure 4–4). Intravenous echo contrast may help in the correct identification of cardiac masses, especially thrombi (see Figure 4–1).
Figure 4–1. Mural thrombus (T) in the left ventricle (LV) of a patient with a left ventricular aneurysm following anterior myocardial infarction. Top panel is an apical four-chamber echocardiogram with second harmonic imaging. The thrombus is well imaged, but delineation of the underlying wall motion abnormality is difficult to appreciate. Bottom panel is the same view with a lipid-based echo contrast agent; note the clearer definition of the thrombus and aneurysm.
Figure 4–2. Apical four-chamber, two-dimensional echocardiogram of a patient with dilated cardiomyopathy showing a protruding thrombus (T) at the apex of the left ventricle (LV).
Figure 4–3. Transesophageal echocardiogram demonstrating the left atrium (LA) with a large thrombus (T) filling the left atrial appendage. Arrows outline the left atrial appendage.
Serial echocardiography greatly enhanced knowledge of the natural history of intracardiac thrombosis associated with acute myocardial infarction in part because the precise onset of the pathophysiologic thrombotic process can be accurately defined. Doppler studies of blood flow within the left ventricle of patients in whom thrombosis develops have shown that apical patterns of low flow precede thrombus formation. Because high-risk factors (anterior infarction and severe apical wall motion abnormality) are present and can be detected prior to thrombus development, it is possible to initiate prophylactic treatment with anticoagulants and decrease the development of thrombus and subsequent systemic emboli.
Spontaneous echo contrast (“smoke”) detected in a cardiac chamber represents low-flow and early rouleaux formation and is frequently associated with thrombus or subsequent development of thrombus. Patients with spontaneous echo contrast or low-flow velocities in the left atrium have a high incidence of left atrial thrombi and thromboembolism.
High-speed contrast CT and magnetic resonance imaging (MRI) can also detect intracardiac thrombus, especially in the left ventricle. These techniques require specialized equipment not always available and are expensive. Delayed enhancement cardiac MRI is, however, highly sensitive and specific in detection of left ventricular thrombi and can be use if TTE including echo contrast is poor quality or indeterminate for left ventricular thrombus or the clinical circumstances dictate.
Although intracardiac thrombi are the most common cardiac cause of peripheral embolization, there are other causes, including valvular vegetations due to infectious or noninfectious endocarditis, calcific emboli due to degenerative aortic and mitral valve disease, tumor emboli from left-sided myxoma, and atheroemboli from friable or mobile plaque in the aorta or great vessels (see Figure 4–4). In addition, embolic events can occur due to paradoxical emboli that originate in the venous system and transverse an intracardiac communication (most commonly a patent foramen ovale) (Figure 4–5). These conditions are readily identified by TTE or TEE, or both.
Figure 4–4. Transesophageal echocardiogram demonstrating a large atheroma (A) in the transverse thoracic aorta (Ao).
Figure 4–5. Transesophageal echocardiogram demonstrating a systemic venous thrombus, which has embolized to the heart and lodged in a patent foramen ovale. LA, left atrium; RA, right atrium. Arrows indicate the thrombus; the open arrow indicates the patent foramen ovale.
Kirkpatrick JN, et al. Differential diagnosis of cardiac masses using contrast echocardiographic perfusion imaging. J Am Coll Cardiol. 2004;43(8):1412–9. [PMID: 15093876]
Mollet NR, et al. Visualization of ventricular thrombi with contrast-enhanced magnetic resonance imaging in patients with ischemic heart disease. Circulation. 2002;106(23):2873–6. [PMID: 12460863]
Srichai MB, et al. Clinical, imaging, and pathological characteristics of left ventricular thrombus: a comparison of contrast-enhanced magnetic resonance imaging, transthoracic echocardiography, and transesophageal echocardiography with surgical or pathological validation. Am Heart J. 2006;152(1):75–84. [PMID: 16824834]
Thambidorai SK, et al; ACUTE Investigators. Utility of transesophageal echocardiography in identification of thrombogenic milieu in patients with atrial fibrillation (an ACUTE ancillary study). Am J Cardiol. 2005;96(7):935–41. [PMID: 16188520]
Weinsaft JW, et al. Detection of left ventricular thrombus by delayed-enhancement cardiovascular magnetic resonance prevalence and markers in patients with systolic dysfunction. J Am Coll Cardiol. 2008;52(2):148–57.[PMID: 18598895]
Treatment of Cardiac Conditions Requiring Anticoagulation
Short- and long-term anticoagulation are integral parts of the treatment of many cardiovascular conditions, including acute coronary syndromes, stroke, peripheral arterial disease, and venous thromboembolic disease. These conditions are discussed in other chapters. Long-term anticoagulation will be discussed in this chapter for the following conditions: atrial fibrillation, native valvular heart disease, prosthetic heart valves, left ventricular thrombus with acute myocardial infarction, remote myocardial infarction, left ventricular aneurysm, dilated cardiomyopathy, atherosclerotic sources of emboli, paradoxical emboli associated with a patent foramen ovale, and intracardiac devices.
Guidelines have been written by a number of organizations, including the American Heart Association (AHA), American College of Cardiology (ACC), American College of Chest Physicians (ACCP), and ESC for the management of anticoagulation in a number of conditions. These guidelines vary in their content and format; however, their recommendations are mostly in agreement.
A. Atrial Fibrillation
Atrial fibrillation can result in atrial thrombus formation (most often seen in the left atrial appendage [see Figure 4–3]), which can subsequently result in embolization (most often recognized clinically to the brain resulting in ischemic stroke). The incidence of stroke in patients with atrial fibrillation not related to rheumatic mitral valve disease is 4–5% per year, 4–5 times greater than in similar patients who do not have atrial fibrillation. The incidence of stroke with atrial fibrillation is age related. For those age 50–59 years, the incidence is about 1.5% per year, but this increases to > 10% per year for those 80 years of age and older.
AHA/ACC/ESC has recommended classifying atrial fibrillation as (1) first episode, (2) paroxysmal, (3) persistent, and (4) permanent. Acute atrial fibrillation is that which occurs for the first time. Chronic atrial fibrillation can be paroxysmal, persistent, or permanent. In paroxysmal atrial fibrillation, episodes spontaneously convert to normal sinus rhythm usually within 7 days. In persistent atrial fibrillation, episodes last longer than 7 days and/or conversion to normal sinus rhythm requires cardioversion, either chemical or electrical. In permanent atrial fibrillation, normal sinus rhythm can no longer be restored, and/or the patient and physician no longer attempt to restore or maintain normal sinus rhythm. The risk of stroke is considered the same for all three categories of chronic atrial fibrillation.
Atrial flutter is much less common than atrial fibrillation; however, these two arrhythmias are often seen in the same individual. Patients with atrial flutter are also at risk for thromboembolism, and therefore, the two arrhythmias are treated the same in terms of anticoagulation.
1. Chronic atrial fibrillation (nonvalvular)—The efficacy of long-term therapy with oral anticoagulants (warfarin) for primary and secondary prevention of stroke in patients with nonvalvular atrial fibrillation has been clearly shown in randomized, placebo controlled trials.
The risks of anticoagulant therapy in this particular patient population are considerable. ICH increases significantly with age greater than 75 years and INRs greater than 4.0.
Randomized studies have also evaluated the effects of aspirin in reducing the incidence of stroke in patients with nonvalvular atrial fibrillation. These studies showed little benefit of aspirin, much less than with dose-adjusted warfarin. The combination of aspirin and fixed, low-dose warfarin has been compared with dose-adjusted warfarin and was not as effective in lowering the incidence of stroke. Aspirin plus clopidogrel was more efficacious than aspirin alone for stroke prevention in nonvalvular atrial fibrillation patients, but inferior to dose-adjusted warfarin despite a similar risk of bleeds.
Anticoagulant therapy in atrial fibrillation reduces the incidence of stroke by > 60%. The usually accepted target INR for the prevention of stroke with warfarin is 2.0–3.0, and the time patients are within this range (time in target range) is predictive of the efficacy of anticoagulation—the best results are when patients are in this range > 65% of the time. Its efficacy is diminished if the INR is less than 2.0, and INRs greater than 4.0 are associated with a higher risk of bleeding, including ICH. In view of the higher risk of anticoagulation in the very elderly, the target INR is at times lowered to 2.0–2.5. The newer oral anticoagulants are more effective in maintaining continuous effective anticoagulation.
In patients with atrial fibrillation and coronary artery disease, antiplatelet drugs may be added to warfarin in certain situations. The ACCP guidelines suggest that patients with stable coronary disease (no acute coronary syndrome in the last year) and atrial fibrillation (requiring anticoagulant therapy) should receive warfarin without antiplatelet agents. For atrial fibrillation patients with an acute coronary syndrome, who are not treated with coronary stent(s), anticoagulant therapy with warfarin and a single antiplatelet agent for 1 year is advised. Atrial fibrillation patients at high risk for thromboembolism, in particular those with previous thromboembolism or an acute coronary syndrome, who do not receive a stent should be treated with warfarin and dual antiplatelet agents (aspirin and clopidogrel) for 12 months. After that time, warfarin alone is advised, just as for patients with atrial fibrillation and stable coronary disease. For atrial fibrillation patients requiring anticoagulant therapy who develop an acute coronary syndrome treated with stent(s), the ACCP guidelines advise warfarin and dual antiplatelet therapy for 1 month for bare metal stents and 3–6 months for drug-eluting stents. After these time periods, warfarin and single antiplatelet therapy (frequently clopidogrel) is advised for the remainder of the first year, followed by warfarin alone, just as for atrial fibrillation and stable coronary artery disease. Patients who require long-term anticoagulation with warfarin are preferably treated with a bare metal stent, rather than a drug-eluting stent if possible, to lower the time of exposure to triple therapy and its risk of bleeding complications. Guideline recommendations for the combination of warfarin and antiplatelet agent(s) vary considerably.
2. Balancing the risk of stroke and anticoagulation in patients with nonvalvular atrial fibrillation—In choosing long-term anticoagulation for patients with nonvalvular atrial fibrillation, the balance between risks and benefits should be considered. The Stroke Prevention in Atrial Fibrillation (SPAF) investigators, the Atrial Fibrillation Investigators, the AHA/ACC/ECS, and the ACCP have determined risk factors for stroke in patients with atrial fibrillation. Assessment of patients for these risk factors is helpful in determining antithrombotic therapy. CHADS2 is a modified stroke risk classification that integrates the above schemes (Table 4–2, left column). Congestive heart failure (C), hypertension (H), age greater than 75 years (A), and diabetes (D) are each assigned 1 point. Patients with previous stroke (S) are assigned 2 points. The CHADS2 risk score assists in determining the approach to antithrombotic treatment (Table 4–3, left column). Lowest risk patients have a score of 0 and do not require warfarin. Intermediate-risk patients have a score of 1 and may be treated with anticoagulant or aspirin when the net clinical benefit is considered (stroke risk reduction with anticoagulant versus risk of major bleeding with anticoagulant). High-risk patients have a score of 2 or greater and derive the most benefit from anticoagulants. Patients with atrial fibrillation who have had a previous stroke should receive anticoagulation therapy unless there is a contraindication.
Table 4–2. CHADS2 and CHA2DS2-Vasc Risk Assessment for Anticoagulation in Nonvalvular Atrial Fibrillation
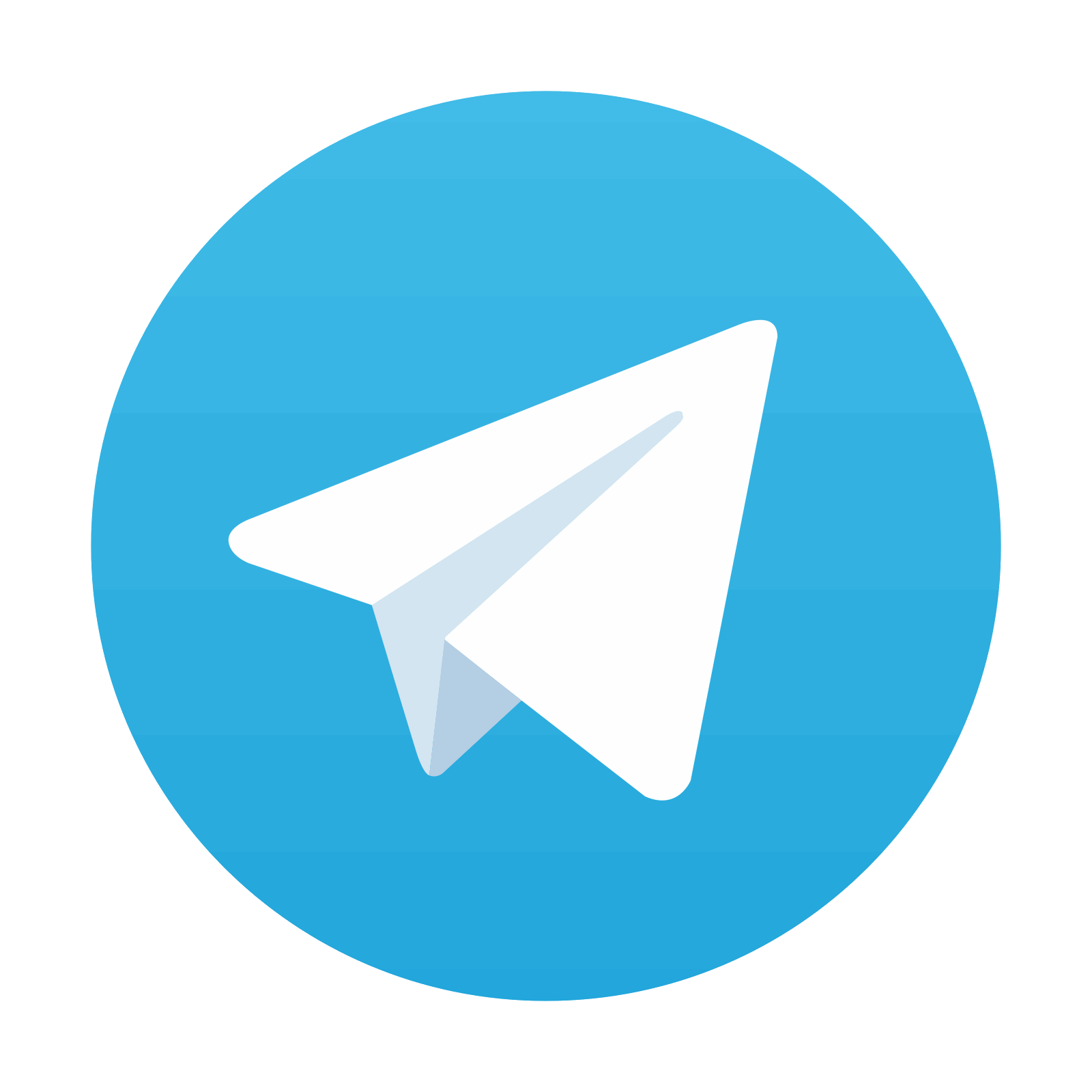
Stay updated, free articles. Join our Telegram channel

Full access? Get Clinical Tree
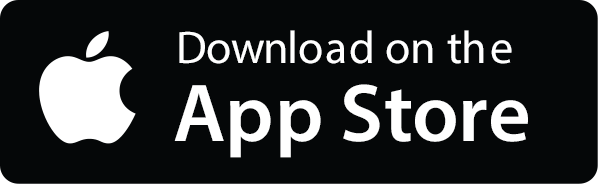
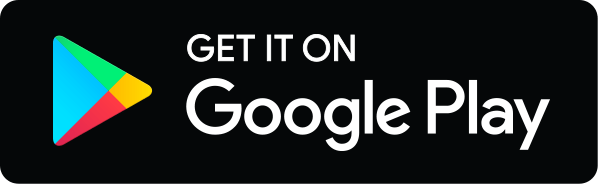