Acute limb ischemia (ALI) refers to the sudden or rapid reduction (i.e., typically 14 days) in arterial extremity perfusion wherein its viability is threatened. Although epidemiologic data are limited, ALI is reported to have an annual incidence of 4 to 17 cases per 100,000 population (1–4). ALI continues to be associated with an extremely grave prognosis, with amputation rates varying from 5% to 30%, and mortality rates as high as 14% to 18% in some series (5–7). This chapter is divided into four sections: (1) A general discussion of ALI, detailing the etiology and pathophysiology of ALI, and the initial clinical assessment, medical management, and general treatment strategies for patients with ALI (2). The role of catheterdirected thrombolysis in the treatment of ALI (3). The role of percutaneous mechanical thrombectomy in the treatment of ALI (4). Case examples of ALI are presented that reinforce the concepts discussed in the earlier sections.
PART I: OVERVIEW OF ALI
Etiology and Pathophysiology of ALI
ALI may result from extrinsic or intrinsic vascular processes leading to sudden compromise of limb perfusion. Acute arterial occlusion secondary to trauma is beyond the remit of this chapter. Classically, intrinsic ALI may result from embolic or thrombotic interruption of arterial blood flow (Table 14.1). Differentiating an occlusion due to embolism from an occlusion due to local thrombosis is frequently challenging (Table 14.2). However, a thorough history and clinical evaluation often yield incisive data, which may help direct patient management.
Arterial embolic occlusion precipitating ALI is cardiogenic in origin in over 80% of cases (8). Intracardiac thrombus formation is most commonly found in the left atrial appendage in the setting of atrial fibrillation (9) and within hypokinetic/ akinetic areas of the left ventricle in the setting of a cardiomyopathy or prior myocardial infarction (10). Artery-to-artery embolization may also occur. Aneurysms within the aorta and iliac, femoral, or popliteal arteries may give rise to in situ thrombus formation, which can then embolize, blocking the downstream arterial system. Other potential causes of embolism are listed in Table 14.1. Emboli tend to lodge at bifurcation points within the arterial tree such as at the distal common femoral, iliac, and popliteal arteries in order of decreasing incidence (Fig. 14.1). Similarly, the distal brachial artery is the most common site of embolic occlusion in the upper limb, which together with the renal and mesenteric vascular beds receive approximately 15% of all systemic emboli (11,12).
Acute peripheral arterial occlusion may also result from abrupt thrombus formation at the site of pre-existing atherosclerotic plaque or prior endovascular intervention or within surgical bypass grafts (native vein or prosthetic). Spontaneous plaque rupture complicated by thrombotic occlusion appears to be a rare event in the periphery and occurs most commonly within the superficial femoral artery (13) (Fig. 14.2). While the key event in the vast majority of acute coronary syndrome (ACS) presentations appears to be related to acute disruption of a pre-existing thin fibrofatty plaque with subsequent thrombosis of the coronary artery (14), less is known about spontaneous plaque rupture precipitating ALI. A recent study demonstrated a very high incidence of plaque rupture (~42%) as determined by intravascular ultrasound in the iliofemoral segment of patients undergoing angioplasty, the majority of which were confined to the proximal segment of each artery, where the greatest atherosclerotic burden was found (15). This suggests that plaque rupture may be more frequent than previously believed in the periphery, even if it is not as frequently complicated by thrombotic occlusion as coronary plaque rupture.
Clinical Approach to the Patient with Suspected ALI
The key determinants of the clinical presentation of ALI include the vascular distribution of the occluded artery, the extent of pre-existing atherosclerotic disease, and the degree of prior collateral development. Typically, embolic occlusion of a peripheral artery with no pre-existing peripheral artery disease (PAD) or collateral network results in the acute onset of the classical ALI findings: pain, pallor, poikilothermia (cold), pulselessness, paresthesia, and paralysis. Physical findings consistent with ALI are usually found one joint level distal to the site of arterial occlusion.
The clinical presentation of acute arterial thrombosis may involve less of the classic signs and symptoms of ALI, which may delay the initial diagnosis. The precise duration of symptoms may also be more difficult to discern, and often the vascular exam of the contralateral limb reveals evidence of significant PAD. Table 14.2 lists the clinical characteristics that may aid differentiation of embolic from thrombotic ALI.
Irreversible limb injury is thought to occur in a limb after about 6 hours of complete interruption of arterial inflow. However, clinically, complete interruption of arterial inflow is rare, due to the presence of collaterals. Hence, the ischemic window is typically considerably longer than 6 hours (16). Muscle cells and subcutaneous tissue are less vulnerable to progressive ischemia compared with neurons and skin. During ischemia, the acute reduction in oxygen impairs mitochondrial production of normal energy products such as ATP and cell contraction ceases. In an attempt to maintain cell integrity, a switch to anaerobic glycolysis is initiated. However, a resultant accumulation of lactic acid limits this salvage pathway and a paradoxical energy production situation occurs, known as the “oxygen wastage phenomenon,” with a buildup of intracellular hydrogen ions and a reduction of pH. The ensuing processes of lipid breakdown and beta oxidation expends more ATP, culminating in failure of cell membrane integrity and elevated systemic levels of potassium, creatinine kinase, and myoglobin (17,18). Ischemic reperfusion injury may have harmful contributory effects enhancing the systemic inflammatory response (19,20).
The Society of Vascular Surgery/International Society of Cardiovascular Surgery (SVS/ISCVS) classification provides an important aid to classify the severity of ALI and guide clinical management. The critical components of this assessment in determining the severity of extremity hypoperfusion include the sensory and motor function of the limb, and the condition of the arterial and venous Doppler signals. Based on the constellation of the clinical findings observed, ALI may be divided into one of three categories, as described in Table 14.3. Category I describes a viable limb that is not under threat. These patients have no motor or sensory symptoms, and have audible arterial and venous Doppler signals. Category II describes a threatened limb that requires revascularization to salvage the limb. This category is distinguished from category I by the loss of arterial Doppler signals in the limb and the onset of sensory and motor dysfunction. Category II is subdivided into marginally (category IIa) and immediately (category IIb) threatened groups. Patients with category IIa ALI have mild sensory symptoms, usually localized to the forefoot. Patients with category IIb have more extensive sensory symptoms extending above the level of the forefoot, and have signs of motor weakness. Category III describes a nonviable limb that has profound sensory loss (typically anesthetic) and significant limb paralysis. Loss of venous Doppler signals is typical. If patients present late, muscle rigor and marbling of the skin may be observed. The limb in these patients has experienced irreversible injury that is not salvageable, even if successful revascularization is performed. Approximately 45% of patients with ALI present with category I ischemia, with 10% having a category III nonviable limb on initial evaluation (21).
Figure 14.1 • Embolic arterial occlusion. A: Angiographic appearance of embolic occlusion in distal popliteal artery (arrow). B: Extracted embolic material removed following left superficial femoral artery cut-down and Fogarty balloon thromboembolectomy.
Figure 14.2 • Thrombotic arterial occlusion. Angiogram from patient with thrombotic occlusion of the length of the superficial femoral artery (SFA) extending from the proximal segment (blac arrow, A) to the distal SFA (white arrow, B), with reconstitution of the distal SFA via collaterals from the profunda femoral artery.
Initial Medical Assessment and Therapy
ALI constitutes a vascular emergency that requires rapid diagnosis and prompt management to reduce the substantial risk to both life and limb. In addition to thorough examination of the affected extremity, clinical examination should focus on the cardiovascular system. Features suggestive of high procedural (especially surgical) risk include the presence of left ventricular failure as evidenced by pulmonary edema, hypotension, or arrhythmia. Clinical signs of other potential diagnoses that may mimic ALI should be looked for such as severe cardiac failure, acute deep venous thrombosis, and acute compressive neuropathy. Baseline metabolic and hematologic laboratory profiles should be obtained, and basic resuscitation measures such as intravenous fluids and continuous cardiac monitoring should be instigated. Fluid and electrolyte replacement requires careful consideration, given the severe metabolic derangements frequently encountered with ALI. Furthermore, mechanical thrombectomy devices resulting in intravascular hemolysis may lead to acute renal failure secondary to nonimmune hemoglobinuria. Due to the significant morbidity and mortality of ALI, further management of the patient often requires the input of multiple specialists and can be best achieved in a critical care setting. In particular, where surgery appears to be the most likely treatment path, immediate consultation should be sought prior to the administration of anticoagulation so as not to preclude spinal anesthesia.
Specific guidelines to aid the physician managing the ALI patient remain extremely limited in comparison with those governing care for patients with an ACS. The Trans-Atlantic Inter-Society Consensus Document on Management of Peripheral Arterial Disease (TASC) II guidelines recommend immediate anticoagulation with intravenous unfractionated heparin (UFH) unless a specific contraindication exists (21). Difficulties with UFH administration include reduced bioavailability at low doses, in addition to marked interpatient variability in anticoagulation, necessitating activated partial thromboplastin time measurement. Low-molecular-weight heparins (LMWHs) (mean molecular weight 4000 to 5000 Da) have a number of advantages over UFH largely due to their more predictable anticoagulation response. They act predominately by enhancing antithrombin activity, but unlike UFH they exhibit reduced thrombin-inactivating properties. Also they have higher ratios of anti-Xa to anti-IIa activity, and lead to lower rates of platelet activation and heparin-induced thrombocytopenia (HIT) (22,23). However, the ease of reversing the anticoagulant effect of UFH with protamine sulfate, and its short half-life compared with that of LMWHs, ensure its continued usefulness in clinical practice. Where heparin is contraindicated (due to the presence of HIT), direct thrombin inhibitors such as bivalirudin or argatroban that bind directly to the active site on thrombin, and the selective factor Xa inhibitor fondaparinux, may be used (14,24). In a retrospective review of 48 patients with suspected or confirmed HIT undergoing lower extremity revascularization, the use of the direct thrombin inhibitor argatroban has been shown to be safe and feasible (25). Major bleeding and the composite end point of death, urgent revascularization or amputation occurred in 6% and 25% of patients, respectively.
Although no specific recommendations exist concerning antiplatelet therapy in ALI, it seems reasonable to administer aspirin (ASA) as soon as possible, given the high burden of cardiovascular disease in this patient cohort (21). The Antithrombotic Trialists’ Collaboration found that ASA and other antiplatelet agents reduced the risk of vascular death, stroke, or myocardial infarction by 22% in high-risk ACS patients (26). PAD patients are known to have higher levels of platelet reactivity and exhibit a lesser response to aspirin (27,28). General guidelines for PAD, such as the 2006 ACC/AHA guidelines for PAD, stipulate that antiplatelet therapy should be initiated where possible (29). Aspirin at a dose of 75 to 325 mg/day is recommended primarily as the drug of choice, with clopidogrel considered an effective alternative therapy in the general management of PAD (30).
Imaging
Following the initial medical assessment and stabilization of the patient with suspected ALI, a decision with regard to further vascular imaging to anatomically define the arterial obstruction and the arterial inflow and outflow needs to be made. Imaging options include computed tomographic angiography, magnetic resonance angiography, arterial duplex ultrasonography, and invasive contrast arteriography. Ultimately, the choice should be individualized based on patient variables (e.g., clinical stability of the patient, the severity of ALI, and comorbidities such as renal dysfunction) and system variables that influence the availability of the different imaging modalities. In general, invasive angiography allows the minimal delay between diagnosis and potential endovascular revascularization. As such, patients with Category IIb ALI should routinely proceed directly to invasive angiography as immediate revascularization is vital to preserve limb viability. For patients with Category I ALI, noninvasive evaluation is generally recommended, as there is typically sufficient time to allow such studies to be performed. Since Category IIa ALI represents the intermediate group, clinical judgment will determine whether noninvasive imaging is performed prior to invasive angiography. For example, where complex anatomic issues are anticipated that might influence the choice of arterial access of the revascularization strategy, preprocedural noninvasive imaging is clearly of benefit. Noninvasive or invasive imaging is futile if the limb is nonviable.
Primary Management Strategies in Patients with ALI
The clinical categories of ALI described above form the basis for broad recommendations regarding the primary management strategy for ALI (31).
For patients with category I ALI (i.e., viable limb), medical therapy with heparin and careful assessment regarding the need for revascularization are appropriate. Indications for proceeding to revascularization include the anticipation of symptoms of limb ischemia based on the functional status and activity level of the patient. The threshold for offering revascularization should reflect the balance between the likelihood of achieving a successful revascularization with the anticipated complication rate based on the patient age, comorbidities, and vascular anatomy (e.g., vascular access, anatomy of occlusion, and quality of arterial inflow and outflow). Therefore, in older sedentary patients who have severe comorbidities and complex arterial anatomy, medical therapy alone may be a reasonable option.
The management of patients with category IIa ALI is similar to that of patients with category I ALI. In this patient cohort, there is typically sufficient time to anatomically define the arterial anatomy while medical therapy is instituted. In general, the threshold for offering revascularization in this cohort is low, with the expectation that reasonably active individuals will experience significant limb ischemia in the absence of revascularization. Since the limbs of these individuals are marginally threatened, there is sufficient time to allow the typical 10- to 24-hour duration of catheter-directed thrombolysis (CDT) therapy. Percutaneous mechanical thrombectomy (PMT) may also reasonably be offered by trained operators with access to a range of thrombectomy technologies, assuming the vascular anatomy is appropriate. If either of these modalities is contraindicated, surgical revascularization should be offered.
The most critical decision making relates to the management of patients with category IIb ALI. In these patients, immediate restoration of flow is essential to maximize the chance of limb salvage. Therefore, the use of traditional methods of CDT that require prolonged infusion of thrombolytic agents to achieve resolution of thrombus is not appropriate. The options, therefore, include the use of surgical revascularization or PMT as the dominant strategy to achieve reperfusion to the limb. In the past, there was a bias toward offering surgical revascularization for this group. For patients who present late (i.e., >14 days), surgical revascularization may remain a better option, given the difficulty in dealing with more chronic clot using endovascular techniques. However, there is an increasing shift toward PMT for the treatment of category IIb ALI, which is driven by an increasing array of thrombectomy technologies and increased operator experience. The use of adjunctive thrombolysis with PMT using bolus dosing rather than infusions may facilitate the aim of restoring limb perfusion quickly.
In general, patients with category III ALI are best managed with a major amputation of the limb. Attempts to revascularize such a limb may actually result in harm, since reperfusion of a limb with extensive muscle necrosis can result in severe metabolic disturbance, including myoglobinemia, which can precipitate acute renal failure. The exception to this generalization is the patient who presents acutely (i.e., within 1 to 2 hours) with signs of category III ALI. These patients have typically experienced an embolic event. In such cases, rapid revascularization of the limb (using surgical revascularization or PMT) should be attempted. Careful observation of such patients following revascularization is clearly critical, understanding that such patients have a significantly increased likelihood of metabolic disturbances and development of compartment syndrome (see below).
PART II: CATHETER-DIRECTED THROMBOLYSIS
Choice of Candidates for Catheter-Directed Thrombolytic Therapy
Decisions regarding the use of thrombolytic therapy for a patient with ALI are founded upon a fundamental knowledge base and the personal experiences that a clinician gains over the course of his or her clinical practice. There exist some data in the literature to suggest which patients do best with thrombolytic therapy, in comparison to other options (e.g., open surgical revascularization), but much of the decision-making process must be intuitive and based on something less than objective information.
Most importantly, thrombolytic therapy is contraindicated in patients with bleeding diatheses. Thus, patients with a history of gastrointestinal bleeding, recent major surgery, or stroke should normally not be considered for pharmacologic thrombolysis (Table 14.4). In addition, patients with contraindications to the angiographic procedures inherent in thrombolytic therapy may represent poor candidates for thrombolysis. Specifically, patients with impaired renal function should be treated with caution. Open surgery, mechanical thrombectomy, or anticoagulation alone (if the ischemia is not severe) may be more appropriate options.
Certain clinical variables have been identified as predictors of outcome with thrombolytic therapy. In a series of 80 patients undergoing arterial thrombolysis, success was defined by the complete (i.e., >80% volume) dissolution of thrombus and the absence of the need for open surgical intervention (32). Success was more frequent in prosthetic graft (78%) and native arterial (72%) occlusions than in vein graft occlusions (53%, P = 0.017) and in nondiabetics than in diabetics (80% vs. 52%, P = 0.031). Lysis was dependent on placement of the catheter into the substance of the thrombus (85% vs. 0% success, P = 0.004) and passage of a guidewire through the occlusive process (92% vs. 10% success, P = 0.001). The only parameter independently predictive of successful outcome, without the use of adjuvant procedures, was the location of the occlusion; additional procedures were necessary in 88% of aortoiliac and 82% of infrainguinal occlusions versus only 17% of upper extremity occlusions (P = 0.005).
The Surgery or Thrombolysis for the Ischemic Lower Extremity (STILE) trial randomized 393 patients with lower extremity ischemia to thrombolysis (i.e., urokinase [UK] or alteplase [rt-PA], 1:2 ratio) or primary surgical intervention (33). Subsequent to the primary publication, two subgroup analyses were published. These studies confirmed the finding that patients with bypass graft occlusions had improved outcome with thrombolysis, compared with results of primary surgical intervention (34). The amputation rate at 1 year was significantly lower in patients treated with thrombolysis, compared with those treated with primary surgical revascularization (P = 0.02). By contrast, patients with native artery occlusions had better outcomes with surgical treatment, with a 1-year amputation rate of 0% versus 10% (P = 0.002) (35). While these data do not exclude the appropriate use of thrombolysis for native artery occlusions, the threshold for using a percutaneous means of restoring arterial perfusion in these cases should be higher than for bypass graft occlusions. Specifically, surgical management should be the primary consideration in patients with more chronic native artery occlusions (i.e., older than 14 days) and those with a reasonable, open surgical option (e.g., those patients with an adequate autogenous saphenous vein conduit).
In a multivariable analysis of the 544 patients randomized to thrombolytic therapy or open surgery in the Thrombolysis or Peripheral Arterial Surgery (TOPAS) trial, 28 variables predictive of amputation-free survival were evaluated (36). Among these, eight main effects were predictive of amputation-free survival. These included two demographic factors: white race (risk ratio [RR] = 1.75; P = 0.003) and younger age (RR = 1.015; P = 0.046). Comorbidities comprised four of the eight main effects: history of central nervous system disease (RR = 1.726; P = 0.005), history of malignancy (RR = 1.615; P = 0.024), congestive heart failure (RR = 2.202; P 0.001), or low body weight (RR = 1.007 per pound; P = 0.006). The severity of the process was also predictive, as gauged by the presence of skin color changes (RR = 1.585; P = 0.007) or pain at rest (RR = 0.503; P = 0.003). All eight effects were similar in the two treatment groups; none of these variables predicted improved outcome with one form of initial therapy over the other (i.e., there was no therapy-by-variable interaction). The length of occlusion, however, predicted whether a patient would fare better with thrombolysis or operation. With a threshold occlusion length of 30 cm, the relative risk for longer occlusions to shorter occlusions was 43% better in patients who received thrombolysis, whereas the situation was reversed for those who were randomized to surgery.
Choice of the Thrombolytic Agent
There exist four clinically available thrombolytic agents: streptokinase (SK), urokinase (UK), alteplase (rt-PA), and reteplase (r-PA). Of interest, SK is the only agent to have been approved for peripheral vascular indications by the U.S. Food and Drug Administration (FDA), although it is rarely used in clinical practice today. UK holds FDA indications for pulmonary embolism, rt-PA for coronary occlusion and pulmonary embolism, and r-PA for coronary occlusion alone. Nevertheless, each agent has been used with success for peripheral artery occlusions, despite this use being off-label for UK, rt-PA, and r-PA (37).
The history of the development of pharmacologic thrombolysis began over 70 years ago. In 1933, Tillett and Garner at the Johns Hopkins School of Medicine discovered that filtrates of broth cultures of certain strains of hemolytic streptococcus bacteria had fibrinolytic properties (38). This streptococcal byproduct was originally termed streptococcal fibrinolysin. The purity of this agent was poor, however. Clinical use, of necessity, awaited adequate purification. Tillett and Sherry administered SK intrapleurally, to dissolve loculated hemothoraces, in the late 1940s (39), but intravascular administration was not attempted until the following decade. Tillett et al. first reported intravascular administration of a thrombolytic agent in an article published in 1955 (40). A concentrated and partially purified SK was injected into 11 patients. This investigation was performed with the intent to gain data on the safety of the agent in volunteers; in no case was the SK administered to dissolve pathologic thrombi. Fever and hypotension developed as the amount of SK approached therapeutic levels. Whereas fever was generally mild and controllable with antipyretics, hypotension was sometimes prominent. The mean fall in systolic pressure was 31 mm Hg, and three of the patients manifested systolic pressures below 80 mm Hg. These untoward reactions were more likely a result of contaminants in the preparation, rather than in the SK itself. Despite these reactions, systemic proteolysis was observed, with a decrease in fibrinogen and plasminogen, concurrent with a mild increase in the prothrombin time.
These early studies were followed by reports on the use of SK in patients with occlusive vascular thrombi. In 1956, E. E. Cliffton, at the Cornell University Medical College in New York, was responsible for the first brief description of the clinical effectiveness of intravascular thrombolytic administration (41). The following year, Cliffton published his results for 40 patients with occlusive thrombi treated with a SK– plasminogen complex (42). The locations of the thrombi were diverse, and included peripheral arterial thrombi, venous thrombi, pulmonary emboli, retinal occlusions, and in two patients, occlusive carotid thrombi. The Cliffton clinical results were far from exemplary; recanalization was not uniform and bleeding complications were frequent. Nevertheless, he must be credited with the first use of thrombolytic agents for the treatment of pathologic thrombi, as well as with the first use of catheter-directed administration of a thrombolytic agent.
Several schemes may be used to classify thrombolytic agents. The agents may be grouped by their mechanisms of action (i.e., those that directly convert plasminogen to plasmin vs. those that are inactive zymogens and require transformation to an active form before they may cleave plasminogen). Thrombolytic agents may be grouped by their mode of production (i.e., those manufactured via recombinant techniques and those of bacterial origin). Of interest, recombinant agents harvested from a bacterial-expression system, such as Escherichia coli, do not contain carbohydrates, while products of mammalian hybridoma (e.g., recombinant prourokinase [r-ProUK] from mouse hybridoma SP2/0 cells) are fully glycosylated. Thrombolytic agents may be classified by their pharmacologic actions (i.e., those that are fibrin specific, binding to fibrin but not to fibrinogen, vs. nonspecific, as well as those that have a great degree of fibrin affinity, binding avidly to fibrin, vs. those that do not). It is most efficient to divide the agents into four groups: the SK compounds, the UK compounds, the tissueplasminogen activators, and then an additional, miscellaneous group, consisting of novel agents distinct from agents in the three other groups.
STREPTOKINASE COMPOUNDS
Streptokinase (SK), originating from the streptococcus bacteria, was the first thrombolytic agent to be described. SK is a 50-kDa molecule with a biphasic half-life comprising a rapid t½ of 16 minutes, and a second, slower t½ of 90 minutes (43). Whereas the initial half-life is accounted for by the complex of the molecule with SK antibodies, the second half-life represents the actual biologic elimination of the protein. SK differs from other thrombolytic agents with respect to the stoichiometry of plasminogen binding. Whereas other agents directly convert plasminogen to plasmin, SK must form an equimolar stoichiometric complex with a plasmin or plasminogen molecule, to gain activity. Only then may this SK–plasmin(ogen) complex activate a second plasminogen molecule to form active plasmin; thus, two plasminogen molecules are used in SKmediated plasmin generation. Unfortunately, SK suffers from the limitation of antigenic potential. Preformed antibodies exist, to a certain extent, in all patients who have been infected with the streptococcus bacterium. Similarly, patients with exposure to SK may have high antibody titers on repeat exposure. These neutralizing antibodies inactivate exogenously administered SK. SK antibodies may be overwhelmed through the use of a large, initial bolus of drug, and a large, initial SK loading dose may be employed in this regard. Some investigators have recommended measurement of antibody titers prior to beginning SK therapy, gauging the loading dose on the basis of this titer (44).
SK administration is complicated by allergic reactions in approximately 2% of patients treated with the development of urticaria, periorbital edema, and bronchospasm. Pyrexia may also occur, but is usually adequately treated with acetaminophen. The major untoward effect associated with SK is hemorrhage. SK-associated hemorrhage may be no different than bleeding associated with any thrombolytic agent. The primary cause is likely the actions of the systemic agent on the thrombi, sealing the sites of vascular disintegrity. The generation of free plasmin, however, may contribute to the problem, with degradation of fibrinogen and other serum clotting proteins, as well as the release of fibrin(ogen)-degradation products that are potent anticoagulants and exacerbate the coagulopathy.
Recognizing potential limitations with SK, anisoylated plasminogen–SK activator complex (APSAC) was developed by pharmacologists at Beecham Laboratories (45). APSAC has a longer half-life than SK, since acylation rendered the complex less susceptible to degradation. Owing to this property, it was anticipated that APSAC would be associated with a reduced risk of rethrombosis. Contrary to expectations, APSAC offered little clinical benefit over other agents, and at present, is not used to treat thrombi in the peripheral vasculature.
UROKINASE COMPOUNDS
MacFarlane first described the fibrinolytic potential of human urine in 1947 (46). The active molecule was extracted, isolated, and named urokinase (UK) in 1952 (47). This UK-type plasminogen activator is a serine protease composed of two polypeptide chains, occurring in low-molecular-weight (32 kDa) and high-molecular-weight (54 kDa) forms. The high-molecularweight form predominates in UK isolated from urine, while the low-molecular-weight form is found in UK obtained from tissue cultures of kidney cells. Unlike SK, UK directly activates plasminogen to form plasmin; prior binding to plasminogen or plasmin is not necessary for activity. Also in contrast to SK, preformed antibodies to UK are not observed. The agent is nonantigenic, and untoward reactions of fever or hypotension are rare. Presently, the most commonly employed UK in the United States is of tissue-culture origin, manufactured from human neonatal kidney cells. UK has been fully sequenced, and a recombinant form of UK (r-UK) was tested in two multicenter trials of patients with peripheral arterial occlusion (48,49). r-UK is fully glycosylated, since it is derived from a murine hybridoma cell line. r-UK differs from UK in several respects. First, r-UK has a higher molecular weight than UK. Second, r-UK has a shorter half-life than its low-molecularweight counterpart. Despite these differences, however, the clinical effects of the two agents have been quite similar.
A precursor of UK was discovered in urine in 1979 (50). ProUK was characterized and subsequently manufactured by recombinant technology, using E. coli (nonglycosylated) or mammalian cells (fully glycosylated) (51). This single-chain form is an inactive zymogen, inert in plasma, but may be activated by kallikrein or plasmin, to form active two-chain UK. This property accounts for amplification of the fibrinolytic process; as plasmin is generated, more ProUK is converted to active UK, and the process is repeated. ProUK is relatively fibrin specific; that is, its fibrin-degrading (fibrinolytic) activity greatly outweighs its fibrinogen-degrading (fibrinogenolytic) activity. This feature is explained by the preferential activation of fibrin-bound plasminogen found in a thrombus over free plasminogen found in flowing blood. Nonselective activators, such as SK and UK, activate free and bound plasminogen equally, and induce systemic plasminemia with resultant fibrinogenolysis and degradation of factors V and VII. Given the potential advantages of ProUK over UK, Abbott Laboratories produced a recombinant form of ProUK (r-ProUK) from a murine hybridoma cell line. This recombinant agent is converted to active two-chain UK by plasmin and kallikrein. r-ProUK has been studied in the settings of myocardial infarction, stroke, and peripheral arterial occlusion. To date, it appears that r-ProUK offers the advantages associated with an agent that does not originate from a human cell source. Fibrin specificity, however, may be lost at the higher-dose levels necessary to effect more rapid thrombolysis.
TISSUE-PLASMINOGEN ACTIVATORS
Tissue-plasminogen activator (t-PA) is a naturally occurring fibrinolytic agent produced by endothelial cells and intimately involved in the balance between intravascular thrombogenesis and thrombolysis. Wild-type t-PA is a single-chain (527 amino acid) serine protease with a molecular weight of approximately 65 kDa. Plasmin hydrolyzes the Arg275–Ile276 peptide bond, converting the single-chain molecule into a two-chain moiety. In contrast to most serine proteases (e.g., UK), the single-chain form of t-PA has significant activity. t-PA has potential benefits over other thrombolytic agents. The agent exhibits significant fibrin specificity (52). In plasma, the agent is associated with little plasminogen activation. At the site of the thrombus, however, the binding of t-PA and plasminogen, to the fibrin surface, induces a conformational change in both molecules, greatly facilitating the conversion of plasminogen to plasmin and resulting in dissolution of the clot. t-PA also manifests the property of fibrin affinity; it binds strongly to fibrin. Other fibrinolytic agents, such as ProUK, do not share this property of fibrin affinity. Recombinant t-PA (rt-PA, alteplase) was produced in the 1980s after molecular cloning techniques were used to express human t-PA DNA (53). A predominantly single-chain form of rt-PA was eventually approved in the United States for the indications of acute myocardial infarction and massive pulmonary embolism. rt-PA has been studied extensively in the setting of coronary occlusion. In the GUSTO-I study of approximately 41,000 patients with acute myocardial infarction, rt-PA was more effective than SK in achieving vascular patency (54). Despite a slightly greater risk of intracranial hemorrhage with rt-PA, overall mortality was significantly reduced.
In an effort to lengthen the duration of bioavailability of t-PA, the molecule was systematically bioengineered. Initial investigations identified regions in kringle 1 and the protease portion of t-PA that mediated hepatic clearance, fibrin specificity, and resistance to plasminogen-activator inhibitor. Three sites were modified to create TNK-tPA (tenecteplase), a novel molecule with a greater half-life and fibrin specificity (55). The longer half-life of TNK-tPA allowed successful administration as a single bolus, in contrast to the requirement for an infusion with rt-PA. In addition, TNK-tPA manifests greater fibrin specificity than rt-PA, resulting in less fibrinogen depletion. In studies of acute coronary occlusion, TNK-tPA performed at least as well as rt-PA, concurrent with greater ease of administration (56).
RETEPLASE
Similar to TNK-tPA, the novel recombinant plasminogen activator, reteplase, comprises the kringle 2 and protease domains of t-PA. Reteplase was developed with the goal of avoiding the necessity of a continuous intravenous infusion, thereby simplifying ease of administration (57). Reteplase, produced in E. coli cells, is nonglycosylated, demonstrating a lower fibrin-binding activity and a diminished affinity to hepatocytes (58). This latter property accounts for a longer half-life than that of rt-PA, potentially enabling bolus injection versus prolonged infusion. The fibrin affinity of reteplase was only 30% of that exhibited with t-PA, similar to UK. The decrease in fibrin affinity was hypothesized to reduce the incidence of distant bleeding complications, in a manner similar to that of SK over rt-PA, in the GUSTO trial. In fact, several properties of reteplase may account for a decreased risk of hemorrhage, including poor lysis of platelet-rich, older clots. Reteplase has demonstrated some benefit over rt-PA in the RAPID 1 and RAPID 2 studies, as well as in GUSTO-III (59). To date, a handful of peripheral arterial and venous studies have been published using this agent (60–63).
Comparison of the Agents in Studies of Peripheral Vascular Disease
To date, there have been few well-designed clinical comparisons of various thrombolytic agents in the peripheral vasculature. There exist a variety of in vitro studies and retrospective clinical trials, most pointing to improved efficacy and safety of UK and rt-PA over SK (64–66). In an analysis of data collected in a prospective, single institution registry at the Cleveland Clinic Foundation, UK demonstrated a diminished rate of bleeding complications when compared with rt-PA (67). Efficacy was not evaluated in this trial.
There have been two prospective, randomized comparisons of UK and rt-PA. Neither was blinded. Meyerovitz and associates from the Brigham and Women’s Hospital (Massachusetts) randomized 32 patients with peripheral artery or bypass graft occlusions, of less than 90 days’ duration, to rt-PA (10 mg bolus, 5 mg/h for a maximum of 24 hours) or UK (60,000 IU bolus, 4000 IU/min for 2 hours, 2000 IU/ min for 2 hours, and then 1000 IU/min to a maximum of 24 hours, total administration) (68). There was significantly greater systemic-fibrinogen degradation in the rt-PA group (P = 0.01), indicating that the fibrin specificity of rt-PA was lost at this dosing regimen. rt-PA patients achieved more rapid initial thrombolysis, but efficacy was identical in the two groups, by 24 hours. The trade-off to more rapid thrombolysis was a trend toward a higher rate of bleeding complications in the rt-PA-treated patients (P = 0.39). The second, randomized comparison of UK and rt-PA was the STILE trial, a three-armed, multicenter comparison of UK (250,000 IU bolus, 4000 IU/min for 4 hours, and then 2000 IU/min for up to 36 hours), rt-PA (0.05 to 0.1 mg/kg/h, for up to 12 hours), and primary operation (33). There was one intracranial hemorrhage in the UK group (0.9%) and two in the rt-PA group (1.5%, no significant difference). Although actual rates of overall bleeding complications and efficacy were not reported for the two thrombolytic groups, the authors remarked that there were no significant differences detected in any of the outcome variables. In a subsequent reanalysis of the data, reported in 1999, the frequency of complete clot lysis was similar with UK and rt-PA, at the time of the early arteriographic study (69). These recent data suggest that the rate of thrombolysis may be quite similar, in direct contradiction to the popularly held view that rt-PA is a much more rapidly acting agent.
A multicenter, blinded trial compared the results of thrombolysis with UK versus r-UK in 300 patients with peripheral arterial occlusion (70). Despite a shorter half-life for r-UK, there were no significant clinical differences noted between the two agents. A North American multicenter trial compared three different doses of r-ProUK to UK in 241 patients with lower extremity arterial occlusions of less than 14 days’ duration (71). While the higher r-ProUK dose was associated with a slightly greater percentage of patients with complete (>95%) clot lysis at 8 hours, there was a mild increase in the rate of bleeding complications in this group, compared with either the UK or the lower-dose r-ProUK group. The fibrinogen levels fell in the higher r-ProUK group, suggesting that fibrin specificity is lost at the higher-dose regimens for this compound.
Technique for Thrombolysis of Peripheral Arterial Occlusions
Once the determination to implement thrombolytic therapy has been made, and the particular agent has been selected, several procedural issues must be resolved. Each of these issues plays an important role in achieving a therapeutic success. Precise attention to every clinical detail is imperative to accomplish reasonably rapid dissolution of the thrombus and normalization of arterial blood flow, without complications that may result in the loss of limb or life.
MANAGEMENT OF THE ANTICOAGULATED PATIENT
At the outset, one must be cognizant of coagulation abnormalities that are frequent in patients with acute arterial occlusions. Many patients are on aspirin or clopidogrel, potentially increasing the risk of puncture site bleeding complications. A significant proportion of patients will be fully anticoagulated with warfarin at the time of presentation, prescribed and administered as a result of cardiac arrhythmia or a previous occlusive event. As well, patients with a distal bypass conduit, such as a prosthetic femoral-tibial graft, may be on long-term anticoagulation. There are two choices in such patients; either correct the international normalized ratio (INR) to acceptable levels (e.g., 1.5 or below) or proceed, using a micropuncture technique. In cases of severe ischemia, a micropuncture technique is elected, using a 4-Fr. system and, if necessary, ultrasound guidance, to ensure a single anterior wall puncture. In cases where an additional period of several hours between presentation and treatment may be tolerated, fresh frozen plasma is given to restore a normal INR. In general, it is recommended to refrain from the use of vitamin K to reverse anticoagulation, since the administration of more than trivial amounts of vitamin K makes subsequent re-anticoagulation extremely difficult.
CHOICE FOR ARTERIAL ACCESS
The choice for the arterial access site is of great importance and is one of the primary determinants of complications associated with thrombolytic therapy. The peripheral pulse examination, preprocedural anatomic assessment (i.e., using preprocedural imaging), and the location of the arterial occlusion guide this decision. In most circumstances, access is attempted using a retrograde approach through the contralateral common femoral artery (e.g., occluded femoral–popliteal graft with normal iliac anatomy) or an ipsilateral antegrade approach through the common femoral artery (e.g., ipsilateral popliteal artery occlusion with diseased contralateral iliac artery). If the contralateral femoral pulse is weak or absent, or in patients with an aortofemoral-bypass graft, a left brachial approach may be more appropriate. For femoral access, a single-wall puncture technique is important. For brachial access, one should maintain a low threshold for an open exposure of the artery. Direct cannulation of the brachial artery, just above the antecubital crease, may prevent complications such as brachial sheath hematoma and peripheral nerve palsies or brachial artery thrombosis.
Irrespective of the site of access, a complete diagnostic arteriogram is necessary in all patients with adequate renal function, obtaining full views of the runoff vessels in the affected limb. A complete interrogation of the lower extremity vessels will allow one to make an accurate assessment of whether or not the event is secondary to thrombosis or embolization, and to provide some indication of the chronicity of the problem. Lastly, it is important to image the ipsilateral outflow vessels adequately to provide baseline distal views should subsequent distal embolization occur during thrombolysis.
PLACEMENT OF THE INFUSION SYSTEM
Just as gaining uncomplicated arterial access is the most important determinant of local bleeding complications (safety), accurate placement of the infusion system into the occluding thrombus is the primary determinant of successful and efficacious clot dissolution.
In the case of occlusion of the superficial femoral artery or a bypass graft that originates from proximal femoral inflow, the contralateral femoral approach is the best. The 5-Fr. short sheath is exchanged for a 6-Fr. crossover sheath. When possible, it is best to place the distal tip of the sheath within the external iliac artery (EIA), to minimize contrast agent loss into the hypogastric (i.e., internal iliac) system.
Next, attempts are made to cannulate the occluded artery or bypass graft. Oblique views are helpful to find the orifice. One useful method is to use a 5-Fr. angled catheter (e.g., Bernstein or glide catheter) and an angled 0.035″ hydrophilic wire, placing the catheter just proximal to the expected occluded ostium with careful manipulation of the wire to gain access to the occlusion. Once access has been achieved, the wire is advanced well into the occlusion, so that the catheter may be advanced into the occluded artery or bypass graft.
At this point, the guidewire may be exchanged for a stiffer wire. A sturdy wire is necessary to advance the thrombolytic infusion catheter into the occlusion, since the infusion catheters do not track as well as other, more flexible catheters. After estimating the length of the occlusion, an infusion catheter with an appropriate length of side holes is chosen and advanced into position. Typically, the infusion catheter should be long enough to cover the length of the occlusion plus a 2 to 5 cm margin at either end of the occlusion (Fig. 14.3).
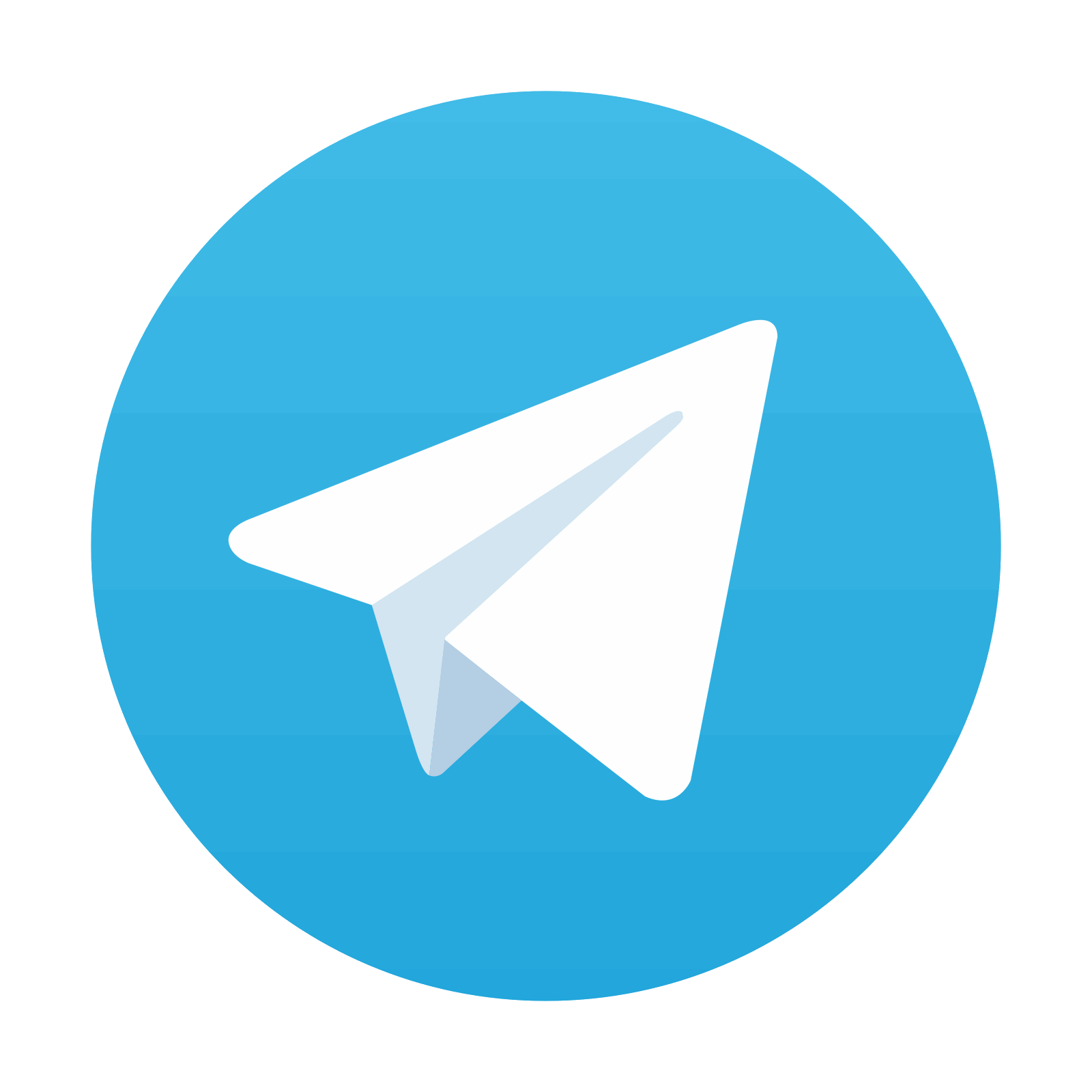
Stay updated, free articles. Join our Telegram channel

Full access? Get Clinical Tree
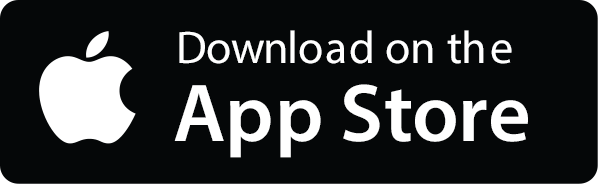
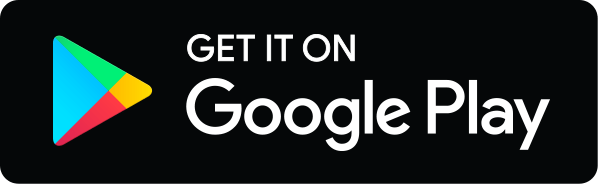