Background
The authors investigated left ventricular (LV) rotational indices, twist, and torsion in a large cohort of fetuses using two-dimensional speckle-tracking echocardiography.
Methods
Pregnant women ( N = 102) were prospectively recruited for fetal two-dimensional speckle-tracking echocardiography. Because of variable fetal position, ventricular orientation was established using the location of the liver and LV position in relation to the right ventricle. Twist measurements required correction to account for fetal position, reconciling directionality across all fetal orientations. Peak apical and basal rotations, global longitudinal strain, global circumferential strain (GCS), longitudinal strain rate, circumferential strain rate (CSR), twist, and torsion were reported and tested for associations with gestational age (GA) and estimated fetal weight (EFW).
Results
Measurement of rotational indices was feasible in 175 examinations (73%). The mean maternal age was 31 ± 6 years, the mean GA 24 ± 6 weeks, and the EFW 1.0 ± 1.0 kg. Mean peak apical rotation, basal rotation, twist, and torsion were 9.5 ± 2.0°, −4.4 ± 1.1°, 13.1 ± 2.3°, and 7.9 ± 3.4°/cm, respectively. Mean global longitudinal strain, GCS, longitudinal strain rate, and CSR were −22.3 ± 4.3%, −25.0 ± 6.1%, −1.4 ± 0.5 sec −1 , and −1.6 ± 0.5 sec −1 , respectively. Absolute GCS, CSR, apical rotation, and twist declined moderately with GA and EFW ( P < .05). There were strong negative nonlinear correlations of torsion with GA and EFW: torsion = 1,104 × GA −1.591 ( r 2 = 0.760, P < .001); torsion = 115.88 × EFW −0.427 ( r 2 = 0.772, P < .001).
Conclusions
Determination of fetal LV rotational mechanics is feasible and reproducible using two-dimensional speckle-tracking echocardiography. Basal rotation, longitudinal strain, and strain rate vary little with EFW and GA. Interpretation of LV apical rotation, twist, torsion, GCS, and CSR, however, must take GA or EFW into account. These data form the basis for comparison with findings in fetuses with abnormal cardiac anatomy and function.
Highlights
- •
Rotational and twist deformation was prospectively investigated using two-dimensional speckle-tracking in a large cohort of fetuses.
- •
Key changes in rotational and twist deformation were noted in relation to gestational age and estimated fetal weight.
- •
This data forms a basis for comparison of rotational and twist deformation to fetuses with abnormal cardiac anatomy and function.
Ventricular function is increasingly understood not simply as myocyte shortening but also as a more complex process in which rotational mechanics are important. Helical myofiber arrangements determine the rotational or wringing motion of the left ventricle, resulting in twist deformation. The outer epicardial myofiber layer rotates the base in a clockwise direction and the apex in a counterclockwise direction, while the inner endocardial layer rotates the apex and base in opposite directions. Because there is a larger epicardial circumference, the epicardial rotation dominates the overall direction. The absolute apex-to-base difference in left ventricular (LV) rotation is the net LV twist (expressed in degrees). The base-to-apex gradient in rotation angle, expressed in degrees per centimeter, is torsion. Although myocardial twist and torsion are important contributors to function in the mature left ventricle, and there is age-dependent variation in twist in children, adolescents, and older adults, there are few data on rotational mechanics in the fetal left ventricle. Two-dimensional (2D) speckle-tracking echocardiography (STE) enables the detection and tracking of tissue pixels and provides measurements of rotation, twist, and torsion that correlate with magnetic resonance imaging methods. We hypothesized that LV rotational indices, twist, and torsion are measurable in the fetus. The purpose of this study was to investigate the feasibility of measurement of fetal LV rotational indices, twist, and torsion and to derive normal values from a large cohort of fetuses using 2D STE.
Methods
Patients
The University of Nebraska Medical Center institutional review board approved the study. One hundred two pregnant women were enrolled through an advertisement in our institutional news bulletin inviting participation in a longitudinal study of normal fetuses. Written informed consent was obtained from all recruited pregnant women. Inclusion criteria were maternal age ≥ 18 years; maternal body mass index 18.5 to 29.9 kg/m 2 ; intact membranes; no history of medical, surgical, or obstetric complications; absence of labor; and normal fetal growth on the basis of US percentiles (estimated fetal weight [EFW] by 2D ultrasound between the 10th and 90th centiles). Fetuses with congenital or known genetic and chromosomal anomalies, heart failure, abnormal cardiac rhythm, or intrauterine growth retardation, as well as multiple-gestation pregnancies, were excluded from the study. Other exclusion criteria were maternal self-reported use of illegal drugs, maternal smoking, antepartum hemorrhage, proven chorioamnionitis, chronic maternal illness, and recent acute illness. Gestational age (GA) was determined by last menstrual period and had been confirmed by a first-trimester sonographic measurement of crown-rump length.
Fetal Ultrasound Acquisition
At enrollment, all pregnant women underwent detailed fetal echocardiographic evaluation in accordance with published guidelines. All images were obtained using a Vivid E9 ultrasound system (GE Medical Systems, Milwaukee, WI) with a C2-9 MHz broadband transducer. Two research sonographers, each with >7 years’ fetal echocardiography experience, performed all examinations (L.L., M.C.).
Images were optimized for gain, compression, depth, and sector width and acquired at frame rates of 70 to 110 frames/sec. Apical or subcostal four-chamber, LV basal and apical short-axis images were acquired. Anatomic landmarks were used to define the basal and apical short-axis levels. The basal level was defined by the mitral valve, and the apical level was defined as the segment of the left ventricle distal to the insertion of the moderator band on the ventricular septum, proximal to the apical cap. Data collected included maternal age, GA, maternal height and weight, fetal biparietal diameter, head circumference, abdominal circumference, femur length, and EFW.
Fetal Heart Orientation
Because of variable fetal position, fetal LV short-axis images are different from the standard postnatal transthoracic parasternal short-axis images. Therefore, fetal heart orientation was established using two parameters: the location of liver and the LV position in relation to the right ventricle. Accordingly, there are four types of orientation for fetal heart short-axis images: (I) the liver is toward the “left of the image” and the left ventricle is at the “bottom of the image,” similar to the traditional transthoracic short-axis image; (II) the liver is toward the “right of the image” and the left ventricle is at the “top of the image”; (III) the liver is toward the “right of the image” and the left ventricle is at the “bottom of the image”; and (IV) the liver is toward the “left of the image” and the left ventricle is at the “top of the image” ( Figure 1 ).

In orientation II, as viewed from the apex toward the base, the LV apex undergoes counterclockwise rotation, whereas the LV base rotates in the opposite direction, clockwise in systole ( Figure 2 A). In orientations III and IV, the fetal heart is viewed from the base toward the apex, so the LV apex rotates in the clockwise direction and the LV base rotates counterclockwise, which are opposite directions compared with orientations I and II ( Figure 2 B). Therefore, in orientations III and IV, the rotation and twist directions on software output were inverted.

Fetal Twist and Torsion Analysis
The raw echocardiographic data sets were transferred from the ultrasound system to a computer workstation for semiautomated analysis (2D Cardiac Performance Analysis version 1.2.3.6 and Image Arena version 4.6; TomTec, Unterschleissheim, Germany). One experienced observer (L.L.) performed all analyses. The fetal cardiac cycle was decided on the basis of anatomic M-mode imaging by detecting atrioventricular valve and LV wall movement. One fetal cardiac cycle was selected from end-diastole to end-diastole. The LV endocardial surfaces were manually traced in basal and apical images using a point-and-click approach when the chamber was at its maximum size after diastole. The software, creating a region of interest, automatically generated an epicardial surface tracing. The region of interest was adjusted to fit the ventricular wall thickness before the automated tracking algorithm was applied. Tracking performance was reviewed to ensure accurate tracking of the ventricular myocardium, and when necessary, manual adjustments were made to the region of interest width and shape before the algorithm was reapplied. The software provided segmental and average values for rotational displacement in degrees on basal and apical views for one complete heart cycle.
LV counterclockwise rotation as viewed from the apex is expressed as a positive value, whereas clockwise rotation is expressed as a negative one. The data depicting basal and apical LV rotation from the software were imported into a custom spreadsheet (Microsoft Excel 2010, Microsoft, Redmond WA) and plotted as graphs (rotational displacement over time). The stepwise calculation of rotational mechanics is shown in Figure 3 with a representative example of apical and basal rotation and LV twist data obtained. Details of the custom Excel spreadsheet are shown in Supplemental Figure 1 (see the Appendix; available at www.onlinejase.com ). Twist is the net difference between apical and basal rotation (value of instantaneous apical rotation − basal rotation). Torsion is the twist value normalized to end-diastolic length, which was obtained from the four-chamber view, measuring from the center of a horizontal line connecting the hinge points of the mitral valve to the apex.

To determine the reproducibility of fetal heart rotation, twist, and torsion parameters, a blinded second observer (M.C.) repeated fetal torsion assessment in 20 randomly selected studies, and the first observer (L.L.) repeated the analysis after a 3-month interval.
Statistical Analysis
All values are expressed as mean ± SD. Values of fetal LV strain, strain rate, rotation, twist, and torsion were evaluated against GA and EFW using linear and nonlinear regression. Although many fetuses were examined more than once, the primary analytic design was cross-sectional, in which only one examination per fetus was included. The examinations for any fetus seen more than once were randomized to allow nonbiased selection of a single examination to represent that fetus. The secondary design of the study was longitudinal, in which only those fetuses examined more than once were included. For each echocardiographic parameter, two-way analysis of variance on a general linear model was applied to determine if a “case effect” was present, in which the identity of the fetus carried a significant correlation with the parameter value independent of GA and EFW. Bland-Altman limit-of-agreement analysis was used to assess inter- and intraobserver reliability. Intraclass correlation coefficients were calculated to test measurement variability. P values < .05 were considered to indicate statistical significance. Statistical analyses were performed using SPSS version 17.0 (IBM, Armonk, NY) and Minitab version 16.0 (Minitab, State College, PA).
Results
Feasibility (All 240 Examinations)
Sixty-five studies (37 with inadequate apical images and 28 with inadequate basal images) were excluded from the analysis. Technical issues in these 65 studies that precluded basal and apical image acquisitions were multifactorial and included ultrasound window limitations in 13 (20%), transducer-heart distance in nine (14%), high maternal body mass index in 18 (28%), placental interposition in five (8%), and unfavorable fetal position in 20 (31%). Measurement of rotational indices was feasible in the remaining 175 studies (73%). Demographic data are shown in Table 1 .
Variable | Mean ± SD | Range |
---|---|---|
Maternal age (yrs) | 30.1 ± 3.8 | 21–40 |
Maternal height (cm) | 166.6 ± 8.0 | 150–185.4 |
Maternal weight (kg) | 75.0 ± 15.9 | 44.1–138.0 |
Maternal BMI (kg/m 2 ) | 24.44 ± 4.75 | 17.66–30.0 |
Fetal GA (wk) | 24.3 ± 5.9 | 15.7–39.3 |
BPD (cm) | 6.7 ± 1.4 | 3.1–9.8 |
HC (cm) | 24.6 ± 6.0 | 11.2–34.6 |
AC (cm) | 23.3 ± 7.0 | 10.1–35.7 |
FL (cm) | 5.1 ± 1.5 | 1.9–7.9 |
EFW (g) | 1,007.9 ± 916.7 | 129.1–3,792.0 |
Fetal HR (beats/min) | 146.5 ± 9.5 | 125–175 |
FR of acquisitions | 75.7 ± 6.6 | 70–110 |
Frames per cardiac cycle | 31.2 ± 3.4 | 24–46 |
Fetal LV Strain and Strain Rate (Cross-Sectional Sample, 102 Examinations)
Global longitudinal strain (GLS), global circumferential strain, longitudinal strain rate, and circumferential strain rate were −22.3 ± 4.3%, −25.0 ± 6.1%, −1.4 ± 0.5 sec −1 , and −1.6 ± 0.5 sec −1 , respectively ( Table 2 ). There was no significant correlation of GLS or longitudinal strain rate with GA or EFW. Absolute value of global circumferential strain and circumferential strain rate declined moderately with GA and EFW ( P < .05; Figure 4 ).
GA | n | Twist (deg) | Base rotation (deg) | Apex rotation (deg) | Torsion (°/cm) | GLS (%) | GCS (%) | LSR (sec− 1 ) | CSR (sec− 1 ) |
---|---|---|---|---|---|---|---|---|---|
Total | 13.1 ± 2.3 | −4.4 ± 1.1 | 9.5 ± 2.0 | 7.9 ± 3.4 | −22.3 ± 4.3 | −25.0 ± 6.1 | −1.4 ± 0.5 | −1.6 ± 0.5 | |
15–19 wk + 6 d | 25 | 13.9 ± 2.1 | −4.4 ± 1.1 | 10.5 ± 2.0 | 12.0 ± 3.0 | −22.3 ± 3.6 | −27.0 ± 7.0 | −1.5 ± 0.6 | −1.8 ± 0.6 |
20–23 wk + 6 d | 34 | 13.6 ± 2.2 | −4.8 ± 1.3 | 9.7 ± 1.7 | 8.4 ± 1.9 | −22.2 ± 4.7 | −25.9 ± 5.8 | −1.4 ± 0.5 | −1.6 ± 0.4 |
24–27 wk + 6 d | 18 | 13.0 ± 2.1 | −4.2 ± 1.1 | 9.6 ± 2.0 | 6.0 ± 1.1 | −21.2 ± 3.2 | −23.0 ± 4.8 | −1.5 ± 0.4 | −1.4 ± 0.4 |
28–31 wk + 6 d | 11 | 11.3 ± 1.9 | −4.1 ± 0.9 | 7.9 ± 1.4 | 4.9 ± 1.1 | −24.1 ± 3.9 | −21.2 ± 4.0 | −1.2 ± 0.3 | −1.4 ± 0.3 |
32–35 wk + 6 d | 8 | 11.7 ± 2.6 | −4.5 ± 1.2 | 7.8 ± 2.3 | 4.5 ± 1.0 | −22.6 ± 5.1 | −26.7 ± 5.9 | −1.4 ± 0.4 | −1.7 ± 0.4 |
36–40 wk | 6 | 12.1 ± 1.4 | −3.9 ± 0.7 | 8.9 ± 1.6 | 3.8 ± 0.6 | −23.3 ± 6.7 | −22.9 ± 6.7 | −1.3 ± 0.3 | −1.5 ± 0.4 |
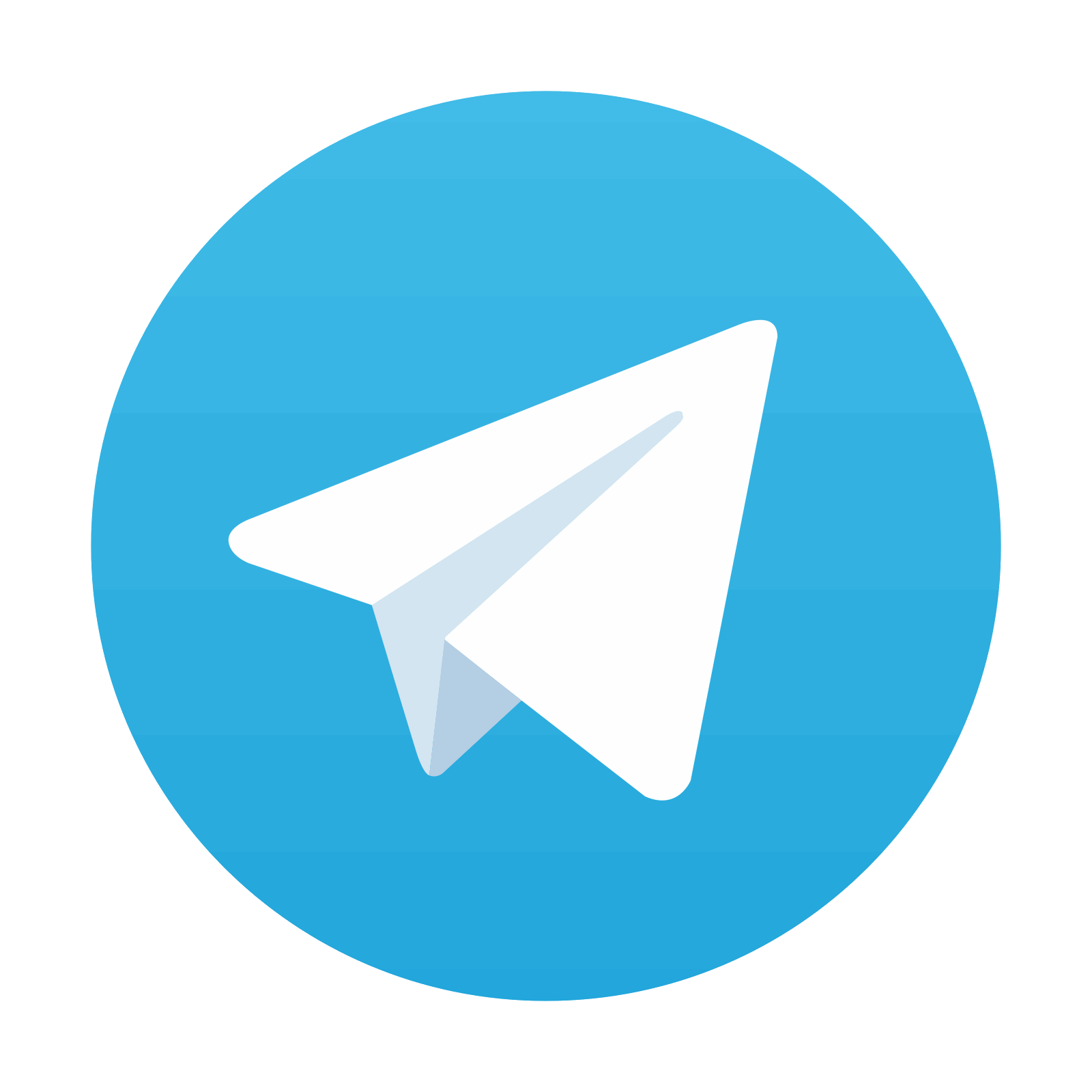
Stay updated, free articles. Join our Telegram channel

Full access? Get Clinical Tree
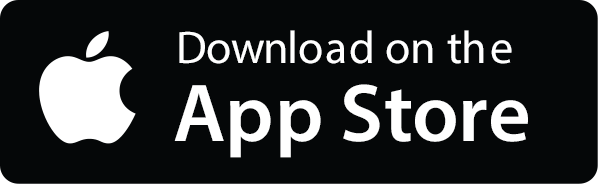
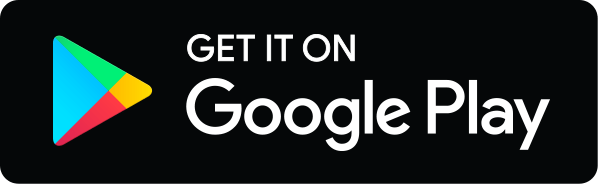
