Background
In patients with asymptomatic aortic stenosis (AS), exercise stress echocardiography (ESE) provides additional prognostic information beyond baseline. The coronary flow velocity reserve (CFVR) is impaired in AS, but its link with exertion is unknown in this setting. The aim of this study was to test the hypothesis that CFVR could predict exercise capacity and abnormal exercise test results in AS.
Methods
Noninvasive CFVR and symptom-limited semisupine ESE were prospectively performed the same day in 43 patients with asymptomatic isolated severe AS (mean age, 68.5 ± 11 years; 26% women; mean aortic valve area, 0.8 ± 0.16 cm 2 ; mean left ventricular ejection fraction, 70 ± 7%). CFVR was performed in the distal part of the left anterior descending coronary artery using intravenous adenosine infusion (140 μg/kg/min over 2 min), and ESE was performed at an initial workload of 25 W with a 20- to 25-W increase at 2-min intervals. An abnormal result on ESE was defined as onset of symptoms at <75% of maximum predicted workload, electrocardiographic ST-segment depression ≥2 mm during exercise, increase of systolic blood pressure < 20 mm Hg or decrease in blood pressure, and complex ventricular arrhythmia. Seventeen patients with isolated severe asymptomatic AS, unable to exercise because of extracardiac conditions, served as a comparative group.
Results
Resting, hyperemic left anterior descending coronary artery flow velocity and CFVR (2.45 ± 0.8 vs 2.4 ± 0.8) were similar between the group unable to perform ESE and the ESE group ( P = NS for all). Compared with patients with normal results on ESE, those with abnormal results on ESE ( n = 22) were older, had higher E/e′ ratios, had higher resting left anterior descending coronary artery flow velocities (39 ± 12 vs 31 ± 8 cm/sec), and had lower CFVR (2.01 ± 0.3 vs 2.85 ± 0.7; P < .01 for all). Furthermore, CFVR was significantly correlated with age, changes in transvalvular pressure gradient and left ventricular ejection fraction with exercise, workload (in watts), and exercise duration ( P < .05 for all). After adjusting for other variables, CFVR remained independently correlated with exercise duration, workload, and abnormal results on ESE ( P < .01 for all). On receiver operating characteristic curve analysis, CFVR < 2.3 was the best cutoff to predict abnormal results on ESE (area under the curve = 0.88 ± 0.06, P < .01).
Conclusions
In patients with asymptomatic severe AS, noninvasive CFVR is correlated with exercise duration and workload, and low CFVR predicts abnormal results on ESE with good accuracy.
Highlights
- •
ESE is very useful for the management of asymptomatic AS but is not often possible for various extra cardiac reasons
- •
Noninvasive coronary flow velocity reserve by transthoracic Doppler echocardiography is independently correlated with exercise capacity and abnormal results on ESE in AS.
- •
The noninvasive coronary flow velocity reserve by transthoracic Doppler echocardiography predicts an abnormal ESE with good accuracy (AUC 0.88 ± 0.06, p < 0.01) in patients with AS.
Managing severe asymptomatic aortic stenosis (AS) with preserved left ventricular (LV) ejection fraction (LVEF) is challenging. Exercise stress testing may help guide management in this setting, but the majority of patients with AS are elderly with several comorbidities, including arthritic conditions and orthopedic limitations, preventing them from achieving a significant workload, and it is therefore difficult to ascribe their limitation to cardiac intolerance. Natriuretic peptides may also be helpful in this setting but in isolation cannot be a determinant in referring a patient to surgery. Therefore, other tools linked to exercise capacity are warranted. The coronary microcirculation is involved in the pathophysiology of AS, as demonstrated several years ago. In patients with AS and without concomitant coronary artery disease (CAD), the coronary flow velocity reserve (CFVR), an index of the coronary microcirculation, is progressively impaired with AS severity. Transthoracic Doppler echocardiography allows the evaluation of noninvasive CFVR with high feasibility in various settings, including AS. We hypothesized that there is a significant relationship between noninvasive CFVR and response to exercise in patients with severe asymptomatic AS and preserved LVEF. Therefore, we conducted a study in asymptomatic patients with severe AS and preserved LVEF able to exercise to test this association. They were referred for exercise stress echocardiography (ESE) and underwent simultaneous assessment of noninvasive CFVR.
Methods
Population
Sixty patients with severe asymptomatic AS (aortic valve area ≤ 1 cm 2 or indexed aortic valve area ≤ 0.6 cm 2 /m 2 ) and preserved LVEF (≥50%) were prospectively enrolled in this two-center study (Compiègne Hospital, Compiègne, France, n = 52; Institut Mutualiste Montsouris, Paris, France, n = 8). Two independent cardiologists blinded to patients’ echocardiographic and biochemical data assessed the absence of symptoms. Patients classified as asymptomatic had to be free of shortness of breath, dyspnea, orthopnea, angina, dizziness, and syncope with exertion. They underwent comprehensive transthoracic Doppler echocardiography and noninvasive assessment of CFVR in the distal part of the left anterior descending coronary artery (LAD) during the same examination. Forty-three patients were able to exercise. They also underwent semisupine ESE the same day. The 17 other patients, unable to exercise because of extracardiac conditions such as arthritic conditions or orthopedic limitations, served as a comparative group. Apart from symptomatic patients ( n = 30 excluded during the study period), exclusion criteria were as follows: significant concomitant valvular disease (at least moderate regurgitation and mitral stenosis, whereas mitral annular calcification was not an exclusion criteria). The quantification of valvular regurgitation was performed using a multiparametric approach including valve morphologic evaluation and measurement of the vena contracta and the effective regurgitant orifice area and regurgitant volume using the proximal isovelocity surface area method. Mitral regurgitation with an effective regurgitant orifice area > 20 mm 2 , regurgitant volume > 30 mL, and aortic regurgitation with an effective regurgitant orifice area > 10 mm 2 , regurgitant volume > 20 mL, or vena contracta width > 3 mm were exclusion criteria ( n = 5). History of CAD (history of myocardial infarction, coronary angioplasty, coronary bypass surgery, or coronary artery stenosis on coronary angiography; n = 5), resting wall motion abnormalities, severe pulmonary disease, and contraindication to adenosine ( n = 1) were also exclusion criteria. Clinical and demographic data were also prospectively assessed. The studies were performed as part of routine clinical care, and all patients gave informed consent for the protocol.
Transthoracic Doppler Echocardiography
Comprehensive echocardiography and CFVR were performed with the same commercially available machine (Vivid E9; GE Healthcare, Little Chalfont, United Kingdom) with an M5S probe. Heart rate and blood pressure were recorded at the same time as echocardiography. All patients were hemodynamically stable. Aortic valve area was measured using the continuity equation according to current guidelines and indexed to body surface area. Transaortic mean pressure gradient was obtained according to the Bernoulli equation. The peak aortic jet velocity was measured using continuous-wave Doppler from multiple acoustic windows to obtain the highest velocity. The time-velocity integral of the LV outflow tract was measured in the apical five-chamber view. Stroke volume was calculated with LV outflow tract area measured from the parasternal long-axis view in systole, at the base of the valve leaflets, from the insertion of the aortic cusp at the level of ventricular septum to the insertion of the aortic cusp at the level of the anterior mitral leaflet. The final value was an average of three beats. LV end-diastolic and end-systolic volumes and left atrial volume were measured from the apical four- and two-chamber views. LVEF was measured according to the modified Simpson rule, and LV mass index was measured according to the American Society of Echocardiography formula. Conventional Doppler parameters were also measured according to a standardized examination, and the final value was an average of three cardiac beats: early (E) and late (A), diastolic transmitral flow velocity, deceleration time of E wave, average of the septal and lateral annular mitral early diastolic (e′), late diastolic (a′), and systolic (S) spectral tissue Doppler velocity, and the E/e′ ratio. Pulmonary artery systolic pressure was calculated as previously described, from the tricuspid regurgitant peak jet velocity and by adding right atrial pressure (estimated from the diameter and the respiratory variation of inferior vena cava diameter). LV rate–pressure product (LVRPP), an index of external cardiac work (EW) and myocardial oxygen consumption, was calculated as (systolic blood pressure + transaortic peak pressure gradient) × heart rate, at rest and during exercise. Another index of EW was also measured including stroke volume in the equation as follows: EW = stroke volume × (mean arterial pressure + transaortic mean pressure gradient) × heart rate × 1.33 × 10 −4 , expressed in joules and calculated at rest and during exercise. All echocardiograms including CFVR measurements were digitized online on hard disks for subsequent offline analysis with the support of a dedicated software package (EchoPAC 7 version 108 for PC; GE Medical Systems, Milwaukee, WI, by one experienced observer blinded to patient data.
Coronary Flow Velocity Reserve
Noninvasive CFVR was performed as previously described using intravenous adenosine infusion (140 μg/kg/min over 2 min). Briefly, the mid-distal part of the LAD was studied using the M5S probe, and the artery was visualized by color Doppler flow mapping guidance in the modified parasternal view. For color Doppler echocardiography, the velocity range was defined as 12 to 16 cm/sec. Blood flow velocity was measured using pulsed-wave Doppler echocardiography, using a sample volume of 3 to 4 mm, placed on the color signal in the distal LAD. The ultrasound beam direction was aligned as closely as possible with the distal LAD flow. No angle correction was performed for the study given that CFVR is the ratio between hyperemic and baseline flow velocity, and it is not affected by the actual flow velocity. However, the angle was kept as small as possible. CFVR was calculated as the ratio of hyperemic to basal peak diastolic flow velocity. Blood flow velocity measurements were performed offline by an experienced investigator blinded to patient data, by contouring the spectral Doppler signals, using the software package of the ultrasound system cited above. Furthermore, diastolic perfusion time was measured at baseline and defined as (duration of the diastolic component of the coronary flow × heart rate). Final values of flow velocity represented an average of three cardiac cycles. We used an intravenous contrast agent (Sonovue; Bracco Imaging, Milan, Italy; one bolus of 0.1 mL, repeated if necessary) in difficult cases to improve visualization of the color Doppler signal and/or to obtain clear spectral Doppler signal in the LAD. The heart rate was monitored continuously during the patient examination, and blood pressure was recorded at baseline and during hyperemia using an automatic arm sphygmomanometer. Intraobserver variability and interobserver variability of CFVR have been reported in patients with AS in a previous report and were 3.6 ± 3.3% and 4.1 ± 4%, respectively.
Exercise Stress Echocardiography
All patients able to perform ESE underwent symptom-limited maximal semisupine bicycle ESE the same day as CFVR evaluation. ESE was performed at an initial workload of 25 W with a 20- to 25-W increase in resistance at 2-min intervals. Patients were asked to maintain a rate of 60 rpm and were encouraged to exercise to exhaustion. Beta-blockers were discontinued on the day of the test. Two-dimensional echocardiography Doppler was performed at baseline and continuously during exercise. Quad-screen images were recorded at baseline, at a low level of exercise, at peak, and at early recovery. Blood pressure was measured every 2 min, and a 12-lead electrocardiogram was continuously monitored. The exercise test was interrupted prematurely in the presence of significant arrhythmias, decrease in systolic blood pressure, or new wall motion abnormalities. Criteria for positive results on ESE were as follows: occurrence of symptoms such as angina, dyspnea, dizziness, limiting breathlessness, or fatigue at low workload (≤75% of maximum predicted workload); ≥2-mm electrocardiographic ST-segment depression; decrease or small increase in systolic blood pressure compared with baseline (<20 mm Hg); and significant ventricular arrhythmias.
Statistical Analysis
Continuous variables are expressed as mean ± SD and categorical variables as percentages. Normal distribution for a variable was assessed using the Kolmogorov-Smirnov test. Unpaired or paired Student’s t test (or the Mann-Whitney and Wilcoxon tests in case of a skewed distribution) the χ 2 test (or Fisher exact test as appropriate) were performed to assess differences according to the variables tested. The relationship between CFVR and resting parameters and those related to exercise was tested with linear and nonlinear correlations, and the best fit was retained. A receiver operating characteristic (ROC) curve was established to assess the best cutoff of CFVR associated with an abnormal exercise test result. Multiple regression analysis was performed to identify independent correlates of exercise capacity. Furthermore, to assess the correlates of an abnormal ESE test result, we performed a LASSO (least absolute shrinkage and selection operator) analysis for the logistic model with the Hpgenselect procedure (SAS version 9.4; SAS Institute, Cary, North Carolina). Considering the small sample size, LASSO analysis is a more flexible method for the number of outcomes necessary per variable in comparison with a multivariate logistic model. Except for the LASSO analysis, statistical analysis was performed using MedCalc for Windows version 15.6.1 (MedCalc Software, Mariakerke, Belgium). P values < .05 were considered to indicate statistical significance.
Results
The baseline characteristics of patients unable to perform ESE, the ESE group, and each subgroup (normal vs abnormal results on ESE) are summarized in Table 1 . Except being older and more frequently women, patients unable to perform ESE had similar characteristics as the ESE group. Coronary flow velocity and hemodynamic measurements at baseline and during adenosine administration are depicted in Table 1 and data related to exercise in Table 2 . CFVR was feasible in all patients, with the help of SonoVue in eight patients (13%). Both tests were well tolerated, and no serious adverse events were noted. The mean CFVR was 2.4 ± 0.7 (range, 1.5–4) in the ESE group and 2.45 ± 0.8 in the group unable to perform ESE ( P = NS). Resting and hyperemic LAD flow velocity, as well as hemodynamic data before and during intravenous adenosine administration, were similar between the group unable to perform ESE and the ESE group ( P = NS for all). Although numerically lower in the group with abnormal results on ESE, resting diastolic perfusion time was not significantly different between groups ( Table 1 , normal vs abnormal results on ESE, P = NS). No wall motion abnormalities were noted during exercise. Figure 1 depicts an example of a patient undergoing ESE.
Unable to perform ESE ( n = 17) | ESE group ( N = 43) | Normal results on ESE ( n = 21) | Abnormal results on ESE ( n = 22) | |
---|---|---|---|---|
Age (y) | 74 ± 11 || | 68.5 ± 11 | 63 ± 12 | 73 ± 9 ∗ |
Women | 8 (47) || | 11 (26) | 4 (19) | 7 (32) |
Body mass index (kg/m 2 ) | 26.8 ± 4.5 | 26.3 ± 4 | 27.3 ± 4.4 | 25.2 ± 3.4 |
Body surface area (m 2 ) | 1.8 ± 0.21 | 1.87 ± 0.2 | 1.9 ± 0.2 | 1.82 ± 0.2 |
Diabetes | 4 (24) | 9 (21) | 2 (10) | 7 (32) |
Dyslipidemia | 7 (41) | 22 (51) | 11 (52) | 11 (50) |
Smoking | 1 (6) | 2 (5) | 2 (9.5) | 0 |
Hypertension | 12 (71) | 27 (63) | 11 (52) | 16 (72) |
LV mass (g/m 2 ) | 110 ± 32 | 113 ± 28 | 111 ± 26 | 115 ± 30 |
LA volume (mL/m 2 ) | 32 ± 13 | 33 ± 11 | 31 ± 11 | 36 ± 11 |
E (cm/sec) | 77 ± 26 | 80 ± 20 | 75 ± 16 | 83 ± 23 |
DTE (msec) | 254 ± 98 | 237 ± 84 | 214 ± 44 | 256 ± 103 |
E/e′ ratio | 14 ± 6 | 13 ± 5.5 | 11 ± 4 | 15 ± 6 ∗ |
LVEF (%) | 70 ± 10 | 70 ± 7 | 71 ± 6 | 69 ± 9 |
PASP (mm Hg) | 35 ± 8 | 32 ± 5 | 32 ± 5 | 33 ± 5 |
Stroke volume (mL/m 2 ) | 43 ± 9 | 47 ± 8 | 48 ± 9 | 46 ± 7 |
Aortic valve area (cm 2 ) | 0.75 ± 0.17 | 0.8 ± 0.16 | 0.82 ± 0.17 | 0.8 ± 0.1 |
Indexed aortic valve area (cm 2 /m 2 ) | 0.42 ± 0.1 | 0.43 ± 0.07 | 0.42 ± 0.07 | 0.44 ± 0.06 |
Mean gradient (mm Hg) | 43 ± 9 | 46 ± 8 | 46 ± 8 | 45 ± 9 |
Peak aortic jet velocity (m/sec) | 4.3 ± 0.4 | 4.3 ± 0.3 | 4.35 ± 0.4 | 4.3 ± 0.4 |
Diastolic perfusion time (sec/min) | 32.5 ± 4 | 32.6 ± 3 | 33.2 ± 3 | 31.9 ± 2.9 |
Adenosine test | ||||
---|---|---|---|---|
At baseline | ||||
Peak LAD flow velocity (cm/sec) | 34.5 ± 9 | 35 ± 11 | 31 ± 8 | 39 ± 12 ∗ |
Heart rate (beats/min) | 72 ± 10 | 72 ± 14 | 67 ± 10 | 75 ± 13 † |
Systolic blood pressure (mm Hg) | 137 ± 25 | 137 ± 15 | 135 ± 14 | 140 ± 15 |
Diastolic blood pressure (mm Hg) | 66 ± 13 | 68 ± 12 | 68 ± 13 | 68 ± 9 |
During hyperemia | ||||
Peak LAD flow velocity (cm/sec) | 82 ± 23 ‡ | 81.5 ± 20 ‡ | 85 ± 17 ‡ | 78 ± 23 ‡ |
Heart rate (beats/min) | 80 ± 11 ‡ | 84 ± 19 ‡ | 85 ± 21 ‡ | 82 ± 19 ‡ |
Systolic blood pressure (mm Hg) | 136 ± 25 | 135 ± 23 | 133 ± 24 | 135 ± 21 |
Diastolic blood pressure (mm Hg) | 61 ± 12 | 62 ± 14 § | 63 ± 15 | 62 ± 11 |
CFVR | 2.45 ± 0.8 | 2.4 ± 0.7 | 2.85 ± 0.7 | 2.01 ± 0.3 ∗ |
Whole group ( N = 43) | Normal results on ESE ( n = 21) | Abnormal results on ESE ( n = 22) | |
---|---|---|---|
Maximum predicted heart rate (%) | 84 ± 11 | 85 ± 9.5 | 83 ± 12 |
Workload (W) | 88 ± 33 | 110 ± 34 | 68 ± 17 ∗ |
Workload (W/kg) | 1.16 ± 0.38 | 1.38 ± 0.4 | 0.96 ± 0.25 ∗ |
Exercise duration (sec) | 417 ± 157 | 520 ± 140 | 319 ± 101 ∗ |
Rest heart rate (beats/min) | 72 ± 13 | 67 ± 10 | 75 ± 13 ∗ |
Exercise heart rate (beats/min) | 129 ± 22 ‡ | 135 ± 18 ‡ | 124 ± 24 ‡ |
Rest systolic blood pressure (mm Hg) | 142 ± 19 | 138 ± 19 | 145 ± 19 |
Exercise systolic blood pressure (mm Hg) | 190 ± 24 ‡ | 191 ± 29 ‡ | 189 ± 18 ‡ |
Rest mean gradient (mm Hg) | 46 ± 8 | 46 ± 8 | 45 ± 9 |
Exercise mean gradient (mm Hg) | 64 ± 13 ‡ | 67 ± 10 ‡ | 60 ± 15 ‡ |
Delta § mean gradient | 18 ± 8 | 21 ± 7 | 15 ± 8 † |
Rest stroke volume (mL) | 88 ± 18 | 92 ± 20 | 85 ± 15 |
Exercise stroke volume (mL) | 95 ± 18 | 100 ± 20 | 88 ± 14 † |
Rest LVRPP (mm Hg · beats/min · 10 3 ) | 15.8 ± 3.5 | 14.4 ± 2.9 | 17 ± 3.5 ∗ |
Exercise LVRPP (mm Hg · beats/min · 10) | 37.2 ± 7.5 ‡ | 40 ± 7 ‡ | 34 ± 7 ∗‡ |
Ratio || LVRPP | 2.45 ± 0.6 | 2.85 ± 0.6 | 2.03 ± 0.4 ∗ |
Rest EW (J) | 117 ± 34 | 116 ± 40 | 117 ± 25 |
Exercise EW (J) | 309 ± 91 ‡ | 346 ± 92 ‡ | 265 ± 74 ∗‡ |
Ratio || EW | 2.86 ± 0.79 | 3.3 ± 0.76 | 2.3 ± 0.4 ∗ |
Rest PASP (mm Hg) | 32 ± 5 | 32 ± 5 | 33 ± 5 |
Exercise PASP (mm Hg) | 63 ± 9 ‡ | 61 ± 10 ‡ | 65 ± 8 ‡ |
Delta § PASP | 32 ± 7 | 30 ± 9 | 32 ± 5 |
Rest LVEF | 70 ± 7 | 71 ± 6 | 69 ± 9 |
Exercise LVEF | 75 ± 9 ‡ | 77 ± 6 ‡ | 71 ± 11 † |
Delta § LVEF | 4.75 ± 4 | 6 ± 3 | 3 ± 5 † |
Delta § LVEF > 4% | 23 (53) | 17 (81) | 6 (27) ∗ |
Rest E/e′ | 13 ± 5.5 | 11 ± 4 | 15 ± 6 ∗ |
Exercise E/e′ | 14 ± 4 | 12.5 ± 4 | 16 ± 5 ∗ |
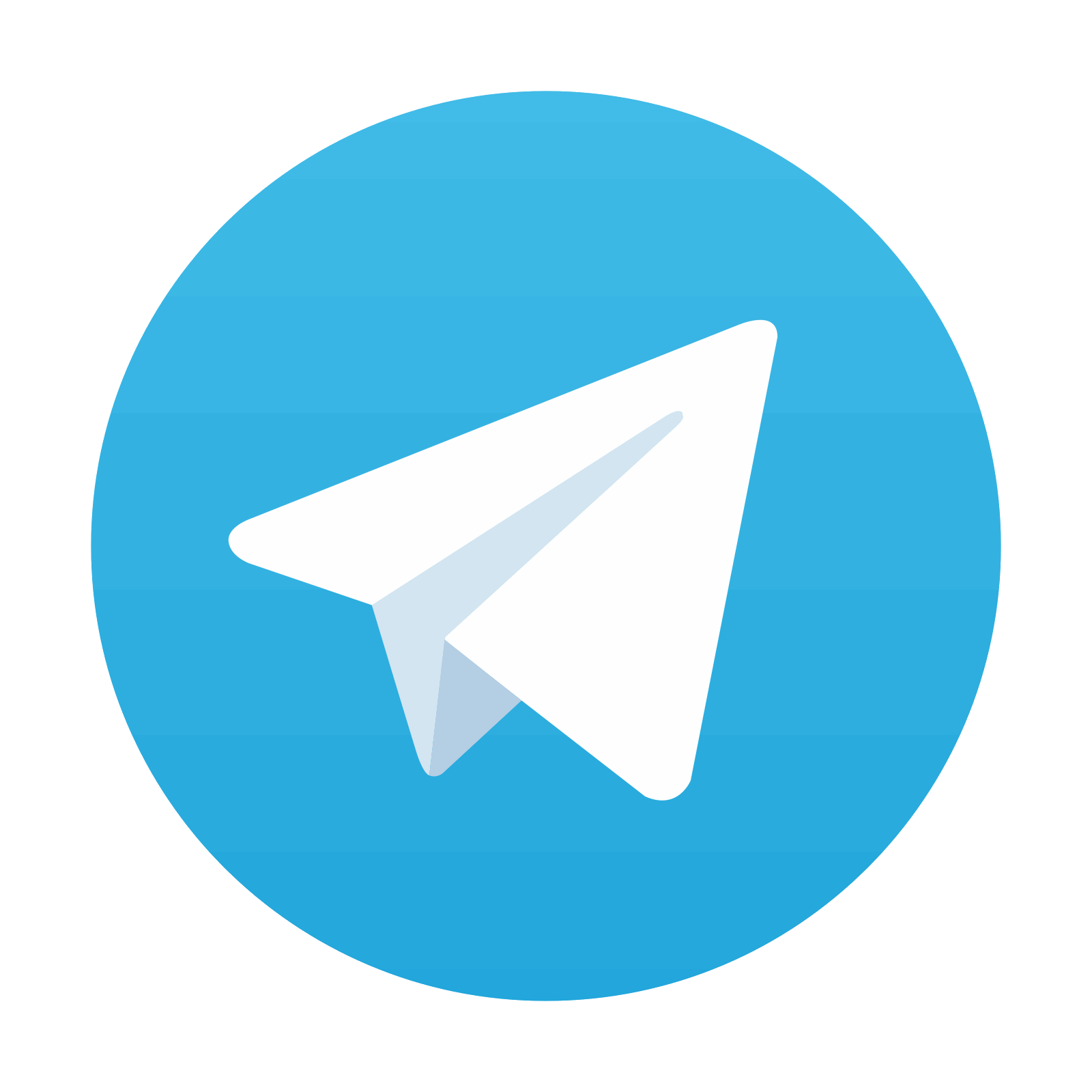
Stay updated, free articles. Join our Telegram channel

Full access? Get Clinical Tree
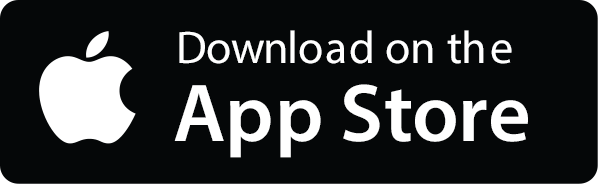
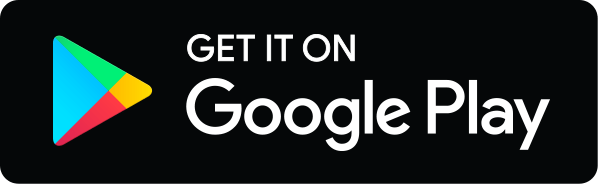
