Background
In adult humans and pig models, strain rate (SR) correlates strongly with invasive measures of contractility but does not demonstrate a force-frequency relationship, which is a fundamental behavior of myocardial contraction. Given the considerable maturational changes of the myocardium from fetal stages to adulthood, extrapolation of adult findings to the young heart may not be appropriate. We sought to evaluate the SR response of the immature heart to increased heart rate (HR) and inotropic stimulation.
Methods
The study consisted of two parts. In part 1, children without obvious structural or functional cardiac abnormalities were evaluated following successful radiofrequency ablation. Echocardiography was performed at baseline HR and then with atrial pacing and isoprenaline infusion titrated to achieve 130% of baseline HR. Left ventricular (LV) speckle tracking–derived SR and tissue Doppler isovolumic acceleration (IVA, a load-independent marker of contractility) were measured. In part 2, young piglets were submitted to atrial pacing at 200, 230, and 260 bpm. Invasive LV dP/dt was assessed, and speckle tracking–derived SR was measured at all stages. Repeated measures analysis of variance was used for comparison with baseline values.
Results
In part 1, the LV SR and IVA (septal and lateral) in 23 children (ages 7.8-17.5 years) increased significantly with pacing and isoprenaline infusion ( P < .001). In part 2, SR and invasive dP/dt increased significantly with increasing HR in young piglets (1-17 days; P < .01 and P < .001, respectively). Both LV SR and dP/dt plateaued at highest HRs concurrent with the decrease in LV end-diastolic dimension from baseline (73.0% ± 9.9% of the baseline value at 260 bpm; P < .001).
Conclusions
SR in children is augmented with chronotropic and inotropic stimulation and in young infant piglets with chronotropic stimulation; in both children and piglets it has a force-frequency relationship, a behavior that is consistent with a measure of contractility.
Highlights
- •
Validation of left ventricular speckle tracking–derived strain rate in young hearts.
- •
Evidence of appropriate strain rate response to chronotropy and inotropy in this age group.
- •
Demonstration of force-frequency relationship, in contrast to adult studies.
A noninvasive, accurate, and reproducible echocardiographic tool for assessing myocardial function is critically important in the diagnosis and clinical management of children with congenital and acquired heart disease. Conventional echocardiographic measures such as fractional shortening (FS) and Simpson’s biplane ejection fraction (EF) are limited by sensitivity to changes in loading conditions and a reliance on geometric assumptions of the ventricle, both factors that are highly variable in congenital heart disease. Annular isovolumic acceleration (IVA) correlates with invasive measures of contractility, is mostly load independent within physiological ranges, and has a positive force-frequency relationship (FFR) ; however, its clinical use is limited by poor interobserver reproducibility except under research conditions. Speckle-tracking echocardiography (STE)-derived global longitudinal strain (GLS) is a reproducible measure that does not rely on geometric assumptions and has been shown to predict patient morbidity and mortality, with increased sensitivity to subclinical myocardial disease. Unfortunately, it too is highly sensitive to changes in ventricular load and provides similar information regarding ventricular ejection performance like FS and EF, rather than myocardial contractility.
More recently, STE-derived strain rate (SR), a measure of the rate of myocardial deformation, has been investigated in adult animal models and patients showing a close relationship to invasive measures of myocardial contractility with relative load independence. However, the finding of heart rate (HR) independence suggests the absence of an FFR, which is surprising and not well explained. FFR and the better known Frank-Starling phenomenon are both intrinsic mechanisms of a cardiac myocyte to regulate myocyte force. While the Frank-Starling mechanism modulates cardiac contractile force through changes in myocardial fiber length, the FFR reflects modulation of the contractile force of cardiac myocytes through a different mechanism. This involves neurohumoral effects on the interplay of calcium-flux modulating proteins in the myocyte membrane, which are influenced by changes in the frequency of stimulation. The exact mechanisms are complex and still not well understood. Moreover, the inability of any measure of cardiac contractility to demonstrate a FFR would raise concerns about its validity.
SR has yet to be validated in pediatric patients. With the known maturational changes of the myocardium from the neonate to adult, we hypothesized that SR response to chronotropy (atrial pacing) and inotropy (isoprenaline infusion) in the immature heart is different from the published findings in adult humans and piglet models.
Methods
This study consisted of two parts. The first was a prospective study that recruited child patients, and the second used a neonatal-young infant equivalent piglet model. Noninvasive and invasive measures of contractility, respectively, were used to investigate SR. The methods and statistical analysis are explained in separate sections below. This study was approved by the human and animal research ethics boards at the University of Alberta Hospital. The animal study design complied with the Canadian Council on Animal Care guidelines.
Clinical Study
We prospectively recruited 23 children (9 males), who underwent an electrophysiology (EP) study with radiofrequency ablation at the Stollery Children’s Hospital for supraventricular arrhythmias. Patients with preoperative echocardiographic findings of abnormal heart structure, a greater than mild myocardial dysfunction, or ventricular dysfunction due to significant dyssynchrony and those not in sinus rhythm or with a pacemaker were not recruited for the study.
All EP studies were performed under general anesthesia and mechanical ventilation. Vascular sheaths were introduced in femoral veins and were then used to position EP catheters in a standard manner for diagnostic and interventional purposes with the use of a right atrial lead for pacing. Following the successful clinical procedure (ablation), the patients were submitted to a standardized provocative testing period that included atrial pacing and inotropic stimulation with isoprenaline infusion. Study echocardiograms were performed using a Vivid 7 ultrasound machine (GE Healthcare, Milwaukee, WI) with a 5- or 7-MHz probe optimized for temporal resolution for STE (frame rate, mean 106 ± 16 Hz). Echocardiograms were performed at baseline HR and repeated following provocative testing, which consisted of (1) atrial pacing to 130% of baseline HR and, separately, (2) isoprenaline infusion titrated to achieve 130% of baseline HR. Echocardiographic measurements were performed offline using EchoPAC BT12 software (GE Healthcare) following standardized guidelines. The variables collected included HR, noninvasive blood pressure, LV dimensions, FS, color TDI–derived IVA, and STE-derived GLS and SR.
Two-dimensional (2D) parasternal long-axis and color TDI data sets in the apical four-chamber view were recorded. The isovolumic contraction period was determined by using M-mode from a parasternal long-axis view to identify mitral valve closure and aortic valve opening. In the apical four-chamber color TDI data set, a sample volume was placed at the septal mitral annulus and separately at the lateral mitral annulus. The software derived a velocity-time curve, and the isovolumic period was zoomed and checked to ensure that at least four frames were contained within the upstroke of the isovolumic velocity curve ( Figure 1 ). Septal and lateral IVA was measured by tracing the slope from zero velocity to the peak of velocity of the isovolumic spike for three consecutive beats by a single observer (S.A.; Figure 1 ). The averaged values at baseline HR, atrial pacing, and isoprenaline infusion were reported. While color TDI was used to measure IVA, ventricular strain and SR measures were not made using color Doppler methods.

Optimized 2D four-chamber views were acquired for measurement of GLS ( Figure 2 ) and SR ( Figure 3 ) using STE at baseline HR, atrial pacing, and isoprenaline infusion. The endocardium was traced, and the onset of the Q wave was set as the beginning of the cardiac cycle. GLS was calculated automatically by the software, and SR was derived and recorded as the peak deformation rate during systole by a single observer (M.A.).


Interobserver reproducibility of IVA, GLS, and SR was evaluated. Efforts were made to blind observers to the conditions, however, this was limited by differences in HR during atrial pacing and inotropic stimulation.
Reproducibility assessment for GLS and SR were performed in 10 patients at baseline, five patients during pacing and five patients during isoprenaline infusion, randomly selected by two observers (M.A. and S.A.). Each observer proceeded to independently select the best cardiac cycle, trace the endocardium, and adjust the region of interest, before the software algorithm for speckle-tracking was applied.
Reproducibility for IVA was performed in 10 randomly selected patients at baseline by two observers (S.A. and K.M.). In an apical four-chamber color-TDI data set, the observers independently placed sample volume at the septal and lateral mitral annulus and independently chose three consecutive beats for analysis.
Neonatal-Infant Piglets
Landrace cross piglets were studied under general anesthesia and mechanical ventilation. Vascular sheaths (5 to 6.5 Fr) were introduced in the common carotids and external jugular veins and were then used for catheter insertion and manipulation. Fluid-filled catheters (3.5 Fr) were positioned in the superior vena cava and the common carotid artery for arterial pressure monitoring. A pacemaker lead (4 Fr) was introduced into the right atrial appendage and connected to an external pacemaker (Medtronic, Minneapolis, MN). A high-fidelity catheter (3.5 or 5 Fr Millar Instruments, Houston, TX) was positioned in the left ventricle (LV) midcavity. Catheter placement was performed under both fluoroscopic and echocardiographic guidance. Blood gas analysis was performed before and immediately after the pacing protocol (iStat system, Abbott Point of Care, Princeton, NJ). Rectal temperature and pulse oximetry were continuously monitored during the experiments.
After instrumentation, a recovery period of 30 minutes was allowed for the piglets to stabilize, defined by less than 10% variation in hemodynamic parameters and normal parameters in an arterial blood gas analysis. The baseline invasive parameters were then recorded with an arterial blood gas, and echocardiography was performed. The measurement dP/dt was generated from the LV pressure curve (derivation of the pressure curve, peak value) automatically by Ponemah Software (Data Sciences International, St. Paul, MN). Max dP/dt is the peak rate of change in pressure in the LV during systole. It was obtained by inserting a high-fidelity Millar pressure catheter through the carotid into the LV. The pressure curves were recorded continuously. The software derived the dP/dt curve, allowing for subsequent offline analysis in order to determine an average peak value over many (20-25) cycles.
Atrial pacing was subsequently started at 200 bpm and progressively increased up to 260 bpm in increments of 10 bpm. The animals were allowed to stabilize for 30 seconds before invasive recording and echocardiograms that were performed at baseline, 200, 230, and 260 bpm. Following completion of the experiments, piglets were euthanized with an overdose of pentobarbital (100 mg/kg intravenously). Data from invasive monitoring were analyzed using Ponemah software. Each data point for aortic blood pressure (systolic, diastolic, and mean) and dP/dt were averaged from 20-25 heart cycles.
Echocardiographic images were acquired in all piglets using a Vivid 7 ultrasound machine (GE Healthcare) with a 10- to 7-MHz probe optimized for temporal resolution (frame rate, mean of 247 ± 7 Hz). Ductus arteriosus closure was confirmed before the beginning of the protocol in the animal subjects. Measurements were done offline using EchoPAC BT12 software (GE Healthcare) following standardized guidelines. The variables collected included mean blood pressure (invasive), Doppler cardiac output (calculated by multiplying the stroke volume [velocity-time integral × LV outflow tract area] by the HR), LV dimensions and calculated FS and LVEF, and STE-derived basal circumferential strain and SR.
Optimized 2D parasternal short-axis views of the atrioventricular valves were acquired for measurement of global circumferential strain (GLS) and SR using STE at baseline HR and during the different atrial paced HR. The endocardium was traced, and the onset of the Q-wave was set as the beginning of the cardiac cycle. GLS was calculated automatically by the software, and SR was derived and recorded as the peak deformation rate during systole.
Observers (E.F.P. and L.M.) performed interobserver reproducibility for circumferential GLS and SR. Twelve cardiac cine loops were randomly selected from four piglets. This consisted of four loops during baseline, three loops during pacing at 200 bpm, three loops during pacing at 230 bpm, and two loops during pacing at 260 bpm. The observers, using the same cardiac loops, then independently traced the endocardium and adjusted the region of interest to optimize tracking. Strain and SR curves were automatically generated by the EchoPAC algorithm, and peak strain and SR were manually measured.
Statistical Analysis
Normality of distribution was tested by D’Agostino and Pearson omnibus normality test. Non-Gaussian distributed data were presented as median [interquartile range]. Paired Wilcoxon signed rank test was used to assess whether the variables differed in children with different conditions. Interobserver variability for SR and IVA was assessed with the intraclass correlation coefficient (ICC). Piglet data were presented as mean ± SD unless specified otherwise. One-way analysis of variance (ANOVA) with repeated measures was used to compare the evolution of parameters through the pacing protocol and applying multiple comparisons with repeated measures tests. Greenhouse-Geisser and variance-homogeneity tests were used for testing ANOVA assumptions. Statistical significance was defined as P < .05. Statistical analysis was performed using SPSS version 19.0 for windows software (SPSS Inc. Chicago, IL).
Results
Clinical Study
Twenty-three children (9 males) participated in the clinical arm of our study (median age, 13 years; range, 7.8-17.5 years). Twelve children had atrioventricular node reentry tachycardia, seven had Wolff-Parkinson-White syndrome, three had atrioventricular reentry tachycardia, and one had permanent junctional reciprocating tachycardia. Twenty-one patients had successful radiofrequency ablation, and two patients with Wolff-Parkinson-White syndrome were deemed not successful. Clinical and echocardiographic findings and response to atrial pacing and inotropic stimulation are summarized in Table 1 . The differences in SR and septal and lateral IVA from baseline to pacing and from baseline to isoprenaline infusion in children are plotted on the graphs in Figures 4 and 5 and demonstrate the relationship to HR of both SR and IVA (septal and lateral) through a plotted graph.
Variables | Baseline | Pacing | Inotropy | P value Baseline vs Pacing | P value Baseline vs Inotropy | P value Pacing vs Inotropy |
---|---|---|---|---|---|---|
HR (bpm) | 88 [76, 99] | 120 [100, 130] | 121 [109, 134] | <.001 | <.001 | NS |
Mean blood pressure (mm Hg) | 55 [50, 63] | 56 [51, 62] | 52 [46, 62] | NS | NS | <.05 |
LV end-diastolic diameter (cm) | 4.1 [3.8, 4.5] | 4.2 [3.8, 4.4] | 4.2 [3.8, 4.8] | NS | <.05 | NS |
LV FS (%) | 32 [26, 35] | 29 [27, 34] | 47 [43, 55] | NS | <.001 | <.001 |
Lateral IVA (m 2 /sec) | 1.2 [0.9, 1.5] | 1.7 [1.3, 2.2] | 2.5 [2.0, 3.1] | <.001 | <.001 | <.001 |
Septal IVA (m 2 /sec) | 1.2 [1.0, 1.6] | 1.9 [1.5, 2.3] | 2.3 [1.9, 2.9] | <.001 | <.001 | <.05 |
LV global longitudinal SR (%/sec) | −0.9 [−1.1, −0.7] | −1.0 [−1.2, −0.9] | −1.9 [−2.1, −1.5] | <.001 | <.001 | <.001 |
LV GLS (%) | −15 [−16, −13] | −15 [−17, −13] | −19 [−21, −19] | NS | <.001 | <.05 |

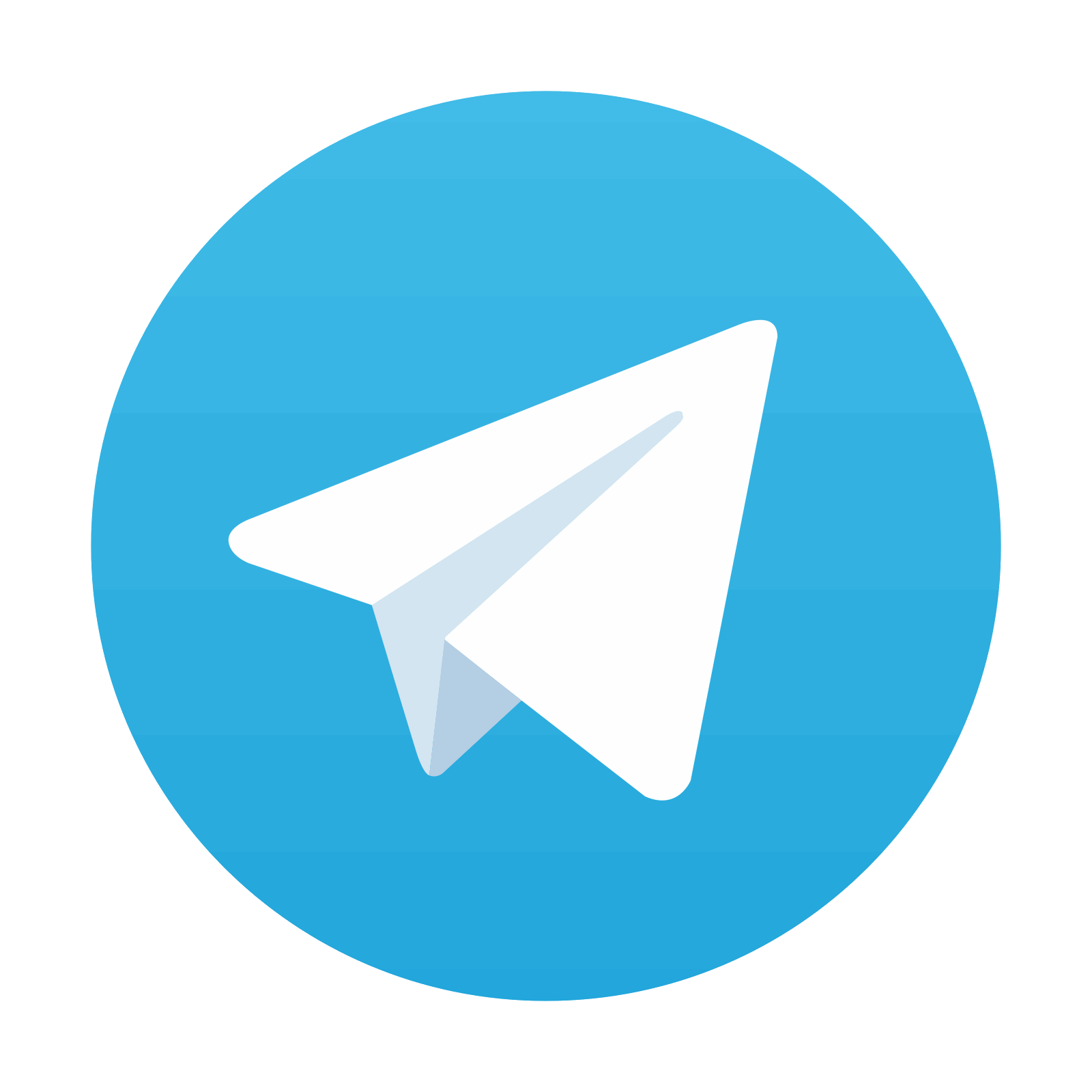
Stay updated, free articles. Join our Telegram channel

Full access? Get Clinical Tree
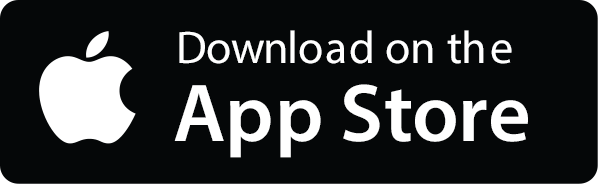
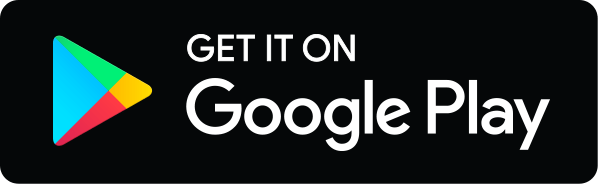
