Chapter 32
Intraoperative Management
Michael P. Lilly, Kapil Gopal
The progressive development and evolution of vascular surgery have been characterized by constant refinement and gradual improvement to optimize the long-term benefit of vascular interventions. The challenge presented by the drive of vascular surgeons to treat critical vascular disease in often severely compromised, older adult patients was a major stimulus to the expansion of knowledge and techniques used in the perioperative period that has occurred over the past several decades. These dramatic advances in anesthetic and intensive care unit (ICU) management have to no small degree facilitated this evolution and contributed greatly to consistent reductions in mortality and morbidity as more and more complex vascular interventions have been applied to sicker patients.
Anesthesia
The term anesthesia refers to the condition in which sensations, principally pain and anxiety, are blocked. The primary use of anesthesia is to allow patients to undergo invasive procedures, including major surgery, without pain or distress. Anesthesia has the indirect benefit of permitting the interventionalist to perform an indicated procedure without the additional impediments associated with an excited, combative, and mobile patient. By addressing the needs of both of the major participants, proper anesthesia allows safe, comfortable, and humane conduct of the delicate, complex, and often protracted interventions that characterize modern surgery.
The fundamental goals of anesthesia are control of pain and thoughtful modulation of neuroendocrine function to assist surgical patients in maintenance of homeostasis in the setting of surgical trauma. Surgeons are well aware that the physiologic impact of our invasive therapies extends beyond the intraprocedural period into the following days and weeks. As such, the supportive treatments through the perioperative period form a continuum, and many therapeutic interventions initiated in the operating room may have ongoing or even greater importance in the postoperative period. Selection of an anesthetic approach for a particular patient must therefore integrate the preexisting status of the patient, the expected impact of the planned intervention, and the predicted needs of the patient during convalescence.
Homeostasis and the Response to Injury
In the mid-1800s, Claude Bernard articulated the concept that organisms must maintain a relatively constant and favorable internal environment to support survival, growth, and reproduction in the setting of a changing and often hostile external world. Homeostasis is a term first used by Walter B. Cannon to describe the dynamic equilibrium created by various regulatory systems though which the body achieves this goal.1 Surgery creates major perturbations of the internal environment and consequently challenges the homeostatic mechanisms of our patients. Surgery (controlled trauma) is a complex physiologic stimulus that may induce pain, hypovolemia, hypoxia, hypothermia, metabolic derangements, anxiety, and a host of other less well-characterized inputs that evoke a well-characterized neuroendocrine response.
Today, we generally consider this response the sum of a series of interacting reflex arcs initiated by stimulation of specific somatic and autonomic afferent sensors, as well as by endocrine and paracrine products of cells at the site of wounding. The goal of each of these specific reflex arcs is to restore the basic homeostatic condition of the organism. For example, hypovolemia from hemorrhage stimulates baroreceptor afferents in the atria and arterial baroreceptor areas of the aortic arch and carotid bifurcation to send signals to the brainstem autonomic cardiovascular areas; these signals result in withdrawal of vagal tone and increased activity of cardiovascular sympathetic afferents, which produces tachycardia, increased myocardial contractility, and increased venous and arterial tone.2 The sum of these effects is an increase in venous return and corresponding increase in cardiac output. The changes in hemodynamics ameliorate the initial disturbance and reduce the stimulus, thereby leading to withdrawal of the reflex as circulatory homeostasis is restored. Similar discrete autonomic reflexes are described for thermoregulation, respiratory control, and glucose homeostasis.3–6 Some of these systems, such as the pituitary-adrenal system, are characterized by more complex regulatory schemes involving feedback and feed-forward input.7–10
A great deal of physiologic research has been conducted to examine each of these reflexes in isolation, but it has become more evident that the idealized model of discrete stimuli and focused responses is rarely seen in clinical medicine. Furthermore, a substantial body of data indicates that the regulatory mechanisms involved in the response to injury interact at multiple levels (sensory, integrative, and effector) to modulate the responsiveness and overall responses of each component of the overall reflex. For example, the complex stimulus of surgery may augment the responses of the classic pituitary-adrenal system to hemorrhage.11–13 Such interactions may operate both across physiologic systems and across time, with depression or augmentation of responses at intervals after earlier (“priming”) stimuli. To further complicate matters, strong evidence suggests that input from the cerebral cortex (rational thought) and limbic cortex (emotion, pain, fear)14 interacts as well, as do inflammatory mediators from the site of injury.15 The essential components of the neuroendocrine response to injury are detailed in several reviews.16–19
General Principles of Anesthesia
The overall goals of anesthesia today are generally viewed through several specific components: analgesia, blocking the conscious sensation of pain; hypnosis, blocking consciousness itself; amnesia, blocking the formation of memory; and relaxation, blocking voluntary motion and somatic motor reflexes, as well as suppressing undesirable autonomic reflexes. Today, each of these goals is assessed separately and is often addressed by specific agents or techniques in a balanced manner to achieve the level of anesthesia necessary for proper conduct of the planned procedure. A breakdown of the levels of anesthesia as developed and described by the American Society of Anesthesiologists (ASA) is presented in Table 32-1.20 The term general anesthesia refers to achieving a loss of consciousness in which subjects are not arousable even by painful stimuli and indicates a sufficient degree of anesthesia to perform major surgical procedures. Patients who under general anesthesia cannot control or protect their airway, may require assisted ventilation, and often have depressed cardiovascular function. Such patients must be managed by skilled anesthesiology professionals who are trained in this particular specialty.
Table 32-1
Continuum of Depth of Sedation: Definition of General Anesthesia and Levels of Sedation/Analgesia
* Minimal sedation (anxiolysis) is a drug-induced state during which patients respond normally to verbal commands. Although cognitive function and coordination may be impaired, ventilatory and cardiovascular functions are unaffected.
† Moderate sedation/analgesia (conscious sedation) is a drug-induced depression of consciousness during which patients respond purposefully¶ to verbal commands, either alone or accompanied by light tactile stimulation. No interventions are required to maintain a patent airway, and spontaneous ventilation is adequate. Cardiovascular function is usually maintained.
‡ Deep sedation/analgesia is a drug-induced depression of consciousness during which patients cannot be easily aroused but respond purposefully¶ after repeated or painful stimulation. The ability to independently maintain ventilatory function may be impaired. Patients may require assistance in maintaining a patent airway, and spontaneous ventilation may be inadequate. Cardiovascular function is usually maintained.
§ General anesthesia is a drug-induced loss of consciousness during which patients are not arousable, even by painful stimulation. The ability to independently maintain ventilatory function is often impaired. Patients frequently require assistance in maintaining a patent airway, and positive pressure ventilation may be required because of depressed spontaneous ventilation or drug-induced depression of neuromuscular function. Cardiovascular function may be impaired.
¶ Reflex withdrawal from a painful stimulus is not considered a purposeful response.
Because sedation is a continuum, it is not always possible to predict how an individual patient will respond. Hence, practitioners intending to produce a given level of sedation should be able to rescue patients whose level of sedation becomes deeper than initially intended. Individuals administering moderate sedation/analgesia (conscious sedation) should be able to rescue patients who enter a state of deep sedation/analgesia, whereas those administering deep sedation/analgesia should be able to rescue patients who enter a state of general anesthesia.
From American Society of Anesthesiologists Task Force on Sedation/Analgesia: Practice guidelines for sedation and analgesia by non-anesthesiologists. Anesthesiology 96:1004, 2002. Developed by the American Society of Anesthesiologists; approved by the ASA House of Delegates October 13, 1999.
The term moderate sedation (formerly referred to as “conscious sedation”) describes a drug-induced depression of consciousness that facilities intervention but does not depress the ability of patients to protect their airway and does not impair independent respiration and normal cardiovascular function. Moderate sedation may be administered by specifically trained individuals with appropriate credentialing. The levels of anesthesia form a spectrum, and patient safety requires careful monitoring of the patient’s responses to the agents used. The conduct of moderate sedation is a potential hazard for patients, and therefore numerous specialty societies, accreditation groups, and regulatory agencies have taken a particular interest in both physician and hospital performance in this area. The Joint Commission has developed standards for health care facilities that conduct moderate sedation,21 and the ASA has published detailed guidelines for both the administration of moderate sedation20 and credentialing of physicians who perform it.22
The balanced approach to general anesthesia was developed in response to the recognition that no single “ideal” anesthetic agent can achieve each of the specific goals of anesthesia with complete safety in all patients throughout the procedural period. The spectrum of activity of every drug extends beyond the specific effect desired by the patient and practitioner. Many anesthetic agents induce disturbances in homeostasis that can impair autonomic homeostatic responses and augment the detrimental impact of surgical trauma. For example, because many volatile inhaled anesthetic agents block reflex control of the cardiovascular system, careful monitoring of cardiac and circulatory function, as well as occasional initiation of additional pharmacotherapy, is required to maintain organ perfusion. The concept of balanced anesthesia is to administer a sequence of drugs with specific actions at safe dosing ranges to achieve the general goals of analgesia, hypnosis, amnesia, and relaxation in a controlled manner with the least risk of undesirable side effects of any particular agent. Because the drugs used in general anesthesia are generally administered systemically by infusion or inhalation, direct drug-to-drug interactions and interactions affecting drug metabolism and clearance are possible. Obviously, this leads to highly specialized and individually tailored polypharmacy.
Many of the goals of anesthesia can be reached without the need for hypnosis or the systemic administration of drugs. Because somatic pain and autonomic afferents are well characterized anatomically, interruption of neural input to the brain can be achieved through temporary neurolysis at either the peripheral or the central (cord) level. This approach can address many, but not all, of the components of the surgical stimulus. Patients may remain anxious, and the systemic effects of the surgical trauma that are not mediated by nerves are unaffected. Such effects include hypovolemia from blood loss or fluid shifts, hypothermia, and release of a host of tissue mediators of inflammation, such as cytokines, prostaglandins, and thrombin, as well as, in certain cases, toxic products of anaerobic metabolism, necrosis, or infection.
Effects, Conduct, and Complications of Anesthesia
A comprehensive review of all relevant scientific and clinical facets of the various modes of anesthesia used in the treatment of vascular conditions is the topic of major texts in anesthesia. Here, the salient aspects of each anesthetic technique most relevant to vascular practitioners are briefly presented, particularly as they relate to current outcomes research. For the impact of anesthetic selection on outcomes in patients with vascular disease, see Anesthesia Technique and Outcomes, later in this chapter.
Local Anesthesia
Local anesthetics produce their effects by interfering with nerve conduction through blockade of neuronal sodium channels.23,24 The inward sodium current is an essential component for propagation of the action potential along nerve fibers. Most local anesthetic agents in use today contain an aromatic ring and are basic and lipid soluble. The agents are made soluble in an acid aqueous vehicle for administration, but in tissue, neutral pH is required to release the basic lipid-soluble component and promote neuronal interaction and effect. This is the theoretical explanation for the diminished effect of local infiltration agents in the relatively acidic environment at sites of inflammation or infection.
Numerous specific agents are available today for local anesthesia. These agents have structural and functional homology but different physiochemical properties that influence selection for specific clinical applications, including potency, rapidity of onset, duration of action, and relative effect on sensory and motor fibers. Table 32-2 lists a number of current agents.25 In clinical practice, most vascular surgeons use lidocaine or bupivacaine. Though less potent than lidocaine, bupivacaine has a longer duration of action that extends well into the postprocedural period and thus may enhance postprocedural comfort.
Table 32-2
Clinical Features of Individual Local Anesthetic Drugs
* Relative to lidocaine.
IVRA, Intravenous regional anesthesia, NA, not available.
From Wildsmith JAW: Clinical use of local anesthetic drugs. In Wildsmith JAW, Armitage EN, McClure JH, editors: Principles and practice of regional anesthesia, London, 2003, Churchill Livingstone, p 65.
Local anesthetics have significant potential for toxicity.26 Systemic toxicity is the result of rapid drug absorption and uptake into the circulation. Beyond the occasional inadvertent intravenous administration of these agents, systemic toxicity may occur as a result of rapid absorption from certain high–blood flow sites, such as mucosal surfaces, peritoneal or pleural spaces, and muscle. Coadministration of a vasoconstrictor (epinephrine) will reduce these effects somewhat. A Bier block, in which a relatively high dose of local anesthetic is administered intravenously into an isolated limb, can deliver a dangerous systemic dose if the tourniquet is prematurely removed. Ester-containing local anesthetics are cleared by plasma cholinesterase rapidly, but amide-based local agents, including lidocaine and bupivacaine, require liver metabolism. Toxic central nervous system effects follow a dose-related progression from vertigo and tinnitus to anxiety and fear and subsequently to tremors, myoclonic jerks, somnolence, seizures, and coma.24 Benzodiazepines may mask the early neurologic signs of systemic toxicity, so particular care is needed in the setting of concomitant moderate sedation. Direct cardiovascular toxicity is seen at levels exceeding the threshold for seizure and is manifested as arrhythmia and myocardial depression.
Moderate Sedation
Moderate sedation can significantly increase patient comfort and the technical ease of invasive procedures. Moderate sedation may be administered by anesthesiology personnel in some settings but is frequently administered by proceduralists themselves with the help of specially trained and credentialed nursing staff. Such practitioners include gastroenterologists, interventional cardiologists, interventional radiologists, interventional nephrologists, pediatricians, emergency medicine physicians, and vascular specialists, among others. Clear knowledge by the practitioner of the agents used, the probable toxicities, and methods of cardiopulmonary rescue is essential for patient safety. The principal hazard of moderate sedation is induction of excessive sedation with a deeper-than-desired level of anesthesia and the potential for hypoventilation and hypoxia. The ASA has developed extensive guidelines for nonanesthesiologists who perform moderate sedation as a part of their practice.20 Only a brief overview is included here.
In preparation, patients should be fasting, and a careful history of allergies, adverse reactions, current medications, and conditions recognized to compromise cardiopulmonary function under sedation (e.g., sleep apnea) should be elicited. A focused physical examination, including vital signs and auscultation of the lungs and heart, along with careful evaluation of the airway, is essential. The facility should have appropriate devices and medications for rescue in the event of oversedation and respiratory compromise. Intraprocedural and postprocedural monitoring is critical to safety. The ASA strongly recommends monitoring of the level of consciousness, oxygenation (pulse oximetry), and arterial pressure (automated oscillometry, every 5 minutes) in all cases, as well as respiration (apnea monitor) and the electrocardiogram in patients undergoing deep sedation and in those with known cardiac disease. Furthermore, it is advised that a specifically trained and designated individual other than the proceduralist be responsible for patient monitoring. Secure vascular access must be obtained and maintained through the procedure and recovery. Most physicians use a combination of sedative/hypnotic and analgesic agents administered in small, incremental doses to achieve the proper balance of effect to complete the procedure in comfort. Table 32-3 lists basic properties of several agents used in modern practice.27 The pharmacology of the agents most commonly used for moderate sedation was recently reviewed.28 Reversal agents (specific antagonists, such as naloxone for opiates and flumazenil for benzodiazepines) must be immediately available. These receptor antagonists may have durations of action shorter than the agents that they are used to block, and therefore continued careful observation of patients is key after any use of rescue agents.
Interest is increasing in the use of dexmedetomidine for procedural sedation. Dexmedetomidine is a highly selective, centrally acting, α2-adrenergic agonist that induces anxiolysis, analgesia, and sedation.28a It has a half-life of 2 to 3 hours. This agent induces sedation with limited respiratory depression and was initially approved for use as a sedative agent in adults needing mechanical ventilation for up to 24 hours.28b Like clonidine, another centrally active α2-agonist, dexmedetomidine has potential sympatholytic effects including hypotension, bradycardia, and decreased circulating norepinephrine, and close hemodynamic monitoring is essential with this agent. These sympatholytic effects have been shown to attenuate the stress response during emergence from general anesthesia for vascular procedures, and dexmedetomidine may be a useful adjunct in the balanced general anesthetic management of vascular patients.28c The broad use of this agent is limited at present by its relatively high cost.
Regional Anesthesia
Regional anesthesia is usually administered by anesthesiologists, often with specific training or interest in peripheral nerves and chronic pain management. Some vascular surgeons perform regional blocks for specific procedures, such as deep and superficial cervical nerve blocks for carotid surgery and ankle blocks for minor foot procedures. The details of administration of these particular blocks can be found elsewhere in this text and in specialty texts.29,30
Spinal/Epidural Anesthesia
Spinal and epidural anesthesia are administered by anesthesiologists, but vascular specialists must be familiar with the advantages and limitations of these approaches, particularly as they affect postoperative management and analgesia. Accordingly, a brief overview of the basic aspects is presented here. Interested readers can refer to many excellent current texts in this field.31–33
Spinal anesthesia is contraindicated in the setting of hemodynamic instability, severe coagulopathy, increased intracranial pressure (and undiagnosed neurologic conditions that could involve increased intracranial pressure), and infection at the injection site. Deformities of the spine increase the difficulty of performing the injection but are not contraindications to this approach. The choice of agent is based primarily on the potency, rapidity of onset, and duration of action of each agent. Factors that are most important in distribution of the agent (and the eventual effect) are the dose, the position of the patient after injection, and the baricity of the solution. Anesthetics for spinal use are prepared in densities different from that of cerebrospinal fluid so that gravity can guide distribution of the agent. Lidocaine and bupivacaine are the most commonly used agents and have durations of action of approximately 60 and 100 minutes, respectively.
Spinal anesthesia produces at least some degree of sympathetic block in most patients. The major impact of this block is loss of arterial and venous tone in the affected areas along with fluid sequestration and hypovolemia. These changes with spinal anesthesia can be significant, and a decrease in systemic vascular resistance of greater than 30% has been reported in older patients with known cardiac disease.34 An indwelling spinal catheter may be placed for continuous or intermittent dosing and may be a good choice for a predictably prolonged surgical procedure. Placement of the spinal catheter requires a larger-bore needle and consequently carries greater risk of post–dural puncture headache. Hypotension is generally treated by prehydration, Trendelenburg positioning, and fluid resuscitation, the sum of which can result in heart failure postoperatively if not managed carefully during the block. Vasopressors or inotropic agents may be required. A properly administered spinal block is not associated with respiratory depression in patients with normal lung function. Patients with chronic obstructive pulmonary disease may rely on the action of accessory muscles of respiration, which may be compromised by a high spinal block. Treatment of a high spinal block consists of cardiovascular and respiratory support with intubation and ventilation in some cases. Unopposed activity of parasympathetic efferents in the setting of sympathetic blockade often leads to increased gut secretions and nausea. These effects are commonly treated with atropine.
Major complications of spinal anesthesia include direct neurologic injury, cauda equina syndrome, arachnoiditis, spinal hematoma, meningitis, post–dural puncture headache, high spinal block with respiratory impairment, and idiopathic cardiovascular collapse. Fortunately, most of these complications are rare, with the exception of post–dural puncture headache, which is attributed to leakage of cerebrospinal fluid and may occur in 25% of patients after spinal anesthesia. The headache is classically relieved by the supine posture and may be associated with cranial nerve symptoms such as diplopia, tinnitus, and nausea. Patients are treated with bed rest, caffeine, hydration, and analgesics.35 Symptoms may persist for 6 weeks. This complication may be treated by the administration of an epidural blood patch, which is thought to seal the meningeal puncture site.36,37 This procedure itself is not without complications, including back pain, fever, nerve root irritation, cranial nerve palsies, and subdural hematoma.
Epidural anesthesia and analgesia in vascular patients involve placement of a catheter in the epidural space at either the lumbar or the thoracic level. Contraindications to epidural catheter placement are similar to those for spinal block, with the exception that recent anticoagulation is not an absolute contraindication. The American Society of Regional Anesthesia and Pain Medicine recently released a consensus statement suggesting that epidural blocks may be performed 4 hours after the last subcutaneous dose of unfractionated heparin and 12 hours after the last subcutaneous dose of low-molecular-weight heparin (LMWH).38 Treatment with nonsteroidal anti-inflammatory drugs or aspirin (or both) is not considered a contraindication if placement is smooth, but authorities recommend discontinuance of clopidogrel 7 days before puncture. The technique of catheter placement is critical to properly enter the epidural space at the appropriate level without injury to the veins of Batson’s plexus. Local anesthetic infused into the epidural space acts on the spinal nerve roots, which are mixed nerves with somatosensory, somatomotor, and autonomic fibers. These component nerve fibers usually have different sensitivity to the blocking effect of anesthetic agents, with the greatest effect on sympathetic autonomic fibers and the least effect on somatic motor nerves. Thus the extent of autonomic, sensory, and motor blockade with a given epidural infusion may differ by several vertebral levels.
The concomitant need for preprocedural, intraprocedural, or postprocedural systemic anticoagulation may limit or complicate the use of spinal or epidural anesthetic techniques in vascular patients. With the proliferation of antithrombotic and antiplatelet agents, the guidelines for use in association with neuraxial anesthesia have become somewhat complex (Table 32-4).38a In general, most guidelines suggest that it is safe practice to consider needle placement (or catheter removal) 4 to 6 hours after discontinuance of unfractionated heparin or 10 to 12 hours after the last dose of LMWH, and systemic heparinization 1 hour after needle or catheter insertion. Generally, elective vascular surgery should be delayed for 12 to 24 hours in the event of a traumatic tap.
Table 32-4
Neuraxial* Anesthesia in the Patients Receiving Thromboprophylaxis
* For patients undergoing deep plexus or peripheral block, follow ASRA recommendations for neuraxial techniques.
† Adapted from the German Society of Anesthesiology and Intensive Care Medicine Consensus guidelines.
‡ Adapted from the Belgian Association for Regional Anesthesia. Working party on anticoagulants and central nerve blocks
§ Adapted from the American College of Chest Physicians.
The major cardiovascular, respiratory, and gastrointestinal complications of epidural anesthesia are similar to those of spinal anesthesia. Accidental dural puncture can lead to post–dural puncture headache, but this problem occurs less frequently with epidural anesthesia than with spinal techniques, which naturally always involve dural puncture. With epidural misplacement, it is possible to inject agents into either the subdural space or the subarachnoid space and potentially result in total spinal anesthesia and even block in the brainstem, which can induce substantial blockade of all cardiopulmonary reflexes, coma, and shock. This complication is extremely rare, but special care must be exercised with high epidurals.
A major advantage of catheter-based epidural anesthesia is the ability to use the catheter for postprocedural analgesia with opioids or local anesthetics, alone or in combination with either physician-controlled or patient-controlled systems. Effective epidural postoperative analgesia obviates the need for systemically administered opioids and the accompanying risk of respiratory depression, excessive sedation, and gastrointestinal side effects. Epidural anesthetic infusions can be maintained in most patients safely for 3 to 4 days.
General Anesthesia
The principles, agents, techniques, and conduct of general anesthesia are beyond the scope of this text. Comprehensive review of these aspects can be found in specialized works.39 Here, only aspects of interest to vascular surgeons are considered, and coverage focuses on data-driven selection of optimal anesthetic approaches as part of the comprehensive team management of periprocedural care in complex vascular patients.
Agents used in general anesthesia, particularly inhaled anesthetics, have profound effects on the heart and circulation. Anesthetic agents induce peripheral vasodilatation and inhibit sympathetic autonomic regulation. Thus the patient has reduced ability to autoregulate the circulation and tissue perfusion and becomes dependent on therapeutic interventions (fluid and inotropes) by the anesthesia team. The loss of tonic vasoconstriction in the periphery also leads to redistribution of blood flow to the skin and loss of thermoregulation with resultant heat loss, a decrease in core temperature, and hypothermia. Similar effects are noted to a lesser degree with local sympathetic blockade induced by spinal and certain regional anesthetic techniques. The data reviewed in this text make it clear that aggressive monitoring and hemodynamic control are essential to achieve the best outcomes. Although data indicate that general anesthesia is associated with relative depression of diaphragmatic function after aortic surgery,40 there is no clear evidence that either endotracheal intubation alone or a reasonable period of positive pressure ventilation directly induces pulmonary complications.41,42
Anesthesia Technique and Outcomes
Because of the precarious general condition of many vascular patients, a great deal of interest has been shown for anesthetic approaches that would reduce the combined physiologic impact of surgical and anesthetic intervention and thus result in better survival of both the patient and the vascular reconstruction. This interest has been keenest in the setting of major open intracavitary vascular reconstruction of the abdominal aorta in high-risk patients with life- or limb-threatening conditions. In this regard it is best to consider the overall anesthetic/analgesic management program through the preoperative, intraoperative, and postoperative phases as a whole rather than focus on particular components in isolation.
Aortic Surgery
The use of epidural approaches to intraoperative anesthesia and postoperative analgesia arose in the 1970s and 1980s as an attractive alternative to standard therapy with balanced general anesthesia and postoperative systemic opioid analgesia. Epidural techniques, generally supplemented with light general anesthesia during the procedure, were applied to high-risk vascular patients with apparent success. A fair number of trials were conducted in this period to compare the relative outcomes of these techniques in high-risk patients.43–47 Unfortunately, these studies produced conflicting results, most likely because of a range of design flaws, including small sample size. Despite these collective shortcomings, a comprehensive meta-analysis combining data garnered from 141 published reports and 9559 total patients suggested a 30% reduction in mortality (odds ratio of 0.7) with the epidural technique (Fig. 32-1).48 Similar findings were reported in a second meta-analysis by Beattie et al in 2001.49 Since then, three major randomized clinical trials have been published that directly address this question and allow valid objective assessment of the relative merits of these different techniques. Each of these reports will be considered in some detail.
Figure 32-1 Effect of neuraxial blockade (NB) on postoperative mortality within 30 days of randomization. Diamonds denote 95% confidence intervals (CI) for odds ratios of combined trial results. The vertical dashed line represents the overall pooled result. The size of shaded boxes is proportional to the number of events. Overall event rates after adjusting for uneven randomization were 113/5811 (1.9%) versus 158/5667 (2.8%). χ2 test for heterogeneity between individual trials, P = .5. SE, Standard error. (From Rodgers A, et al: Reduction of postoperative mortality and morbidity with epidural or spinal anaesthesia: results from overview of randomised trials. BMJ 321:1493, 2000.)
The first study is the Veterans Affairs (VA) Cooperative Study #345, reported by Park et al in 2001.50 This prospective, randomized trial was conducted at 15 VA Medical Centers to compare specific defined outcomes in high-risk patients (ASA class III or IV) undergoing elective major abdominal surgery (gastric, biliary, colonic, and aortic). Patients were randomized to one of two anesthetic schemes: group 1 received balanced general anesthesia with isoflurane or fentanyl and postoperative pain management with systemic morphine or meperidine (intravenous or intramuscular, physician or patient controlled); group 2 received epidural anesthesia with bupivacaine combined with light general anesthesia with isoflurane or fentanyl and postoperative pain management with epidural morphine supplemented with intravenous morphine as needed. A total of 2731 patients were screened and 1021 were randomized. Most of the exclusions were for insufficient risk class, contraindication to epidural anesthesia, and unwillingness of the surgeon to randomize the anesthesia technique. The authors evaluated 10 primary and 7 secondary endpoints, including death, myocardial infarction, congestive heart failure, ventricular tachycardia, pulmonary embolism, respiratory failure, renal failure, and cerebral events. Of the 984 patients who entered the study, approximately 38% underwent major aortic surgery. The study was powered to detect a 50% reduction in relative risk of a projected 15% major complication rate. No differences were noted in the occurrence of any of the primary or secondary endpoints between the two groups. Although epidural catheters were in use for only a mean of 55 hours, pain scores were significantly less in the epidural group. A post hoc subgroup analysis of the aortic patients (n = 374) showed that occurrence of any of the 10 primary outcome events was reduced significantly in patients managed with the epidural technique (22% versus 37%), mostly as a result of a reduction in the rate of myocardial infarction, respiratory failure, and stroke. The data suggest that the two anesthetic approaches are equivalent across the range of major abdominal surgery, with some advantage seen in postoperative pain control in the epidural group. The study suffers from the lack of blinding of the treating physicians, lack of proscribed guidelines for postoperative hemodynamic management, and less-than-optimal analgesic administration in the standard-therapy group.
The second study, a single-institution study performed at Johns Hopkins Medical Center, was also reported in 2001.41 This prospective, randomized, double-blinded trial used a complete block design to assess the effects of both the intraoperative anesthesia technique and postoperative analgesic management in high-risk patients undergoing abdominal vascular surgery. Patients were randomly assigned to one of four groups: (1) general anesthesia with postoperative intravenous patient-controlled analgesia (PCA), (2) general anesthesia with postoperative epidural PCA, (3) epidural anesthesia and supplemental general anesthesia with postoperative intravenous PCA, and (4) epidural anesthesia and supplemental general anesthesia with postoperative epidural PCA. All randomized patients had an epidural catheter placed before surgery (thoracic epidural, T8-T9 or T10-T11, depending on the surgical approach), and the epidural was kept in place for at least 72 hours postoperatively. Enflurane was the inhaled agent used intraoperatively. Fentanyl was used for intravenous PCA, and combined fentanyl/bupivacaine was used for epidural infusion both intraoperatively and postoperatively. The primary endpoint was length of hospital stay, and the study was powered to detect a 20% reduction in an expected 12.7-day stay. Explicit detailed protocols were used for hemodynamic management, fluid administration, transfusion, and extubation. The study was closed prematurely after 168 patients were enrolled, when a preliminary conditional power analysis by the Data Monitoring Committee showed minimal likelihood of detecting a significant difference among the treatment groups if enrollment were to continue. The data showed no differences in the occurrence of death, myocardial infarction, myocardial ischemia, reoperation, pneumonia, and renal failure between the treatments. Similarly, ICU length of stay, time to initiation of diet, time to independent ambulation, and pain scores were not different in the four groups. Epidural PCA was associated with a significantly shorter time to extubation. These data demonstrate that in a relatively idealized, controlled setting with well-designed and well-managed treatment protocols, there are no measurable differences in critical outcomes among these techniques.
The third study to consider is the Multicentre Australian Study of Epidural Anaesthesia (the MASTER Trial), reported by Rigg et al in 2002.51 This randomized controlled trial (RCT) conducted in Australia and five other countries recruited 920 patients between 1995 and 2001. The study was intended to confirm the benefit of epidural anesthesia noted in an earlier Australian trial by Yeager et al47 while using more consistent and modern anesthetic and analgesic technique. High-risk patients defined by a panel of specific indicators who were to undergo elective major abdominal surgery or esophagectomy were eligible for recruitment. Patients were randomized to either control, consisting of balanced general anesthesia with postoperative intravenous opioid analgesia (physician or patient controlled), or epidural procedural anesthesia with postoperative epidural analgesia (combined opioid and local anesthetic, continuous infusion). Detailed protocols were included for the management of each aspect of care throughout the study period.52 There was no masking of treatment in this study. The primary outcome was combined 30-day mortality and morbidity, and the study was powered to detect a 20% decrease in this parameter from an expected 50% value; 77.6% of patients were recruited in Australian centers, and 18% of patients underwent major aortic surgery (repair of abdominal aortic aneurysm [AAA]). The data showed no difference between the two treatments with regard to overall 30-day outcome (Table 32-5).51,52 However, significant differences in pain score and respiratory failure favored the epidural group.
Taken together, these data indicate that selection of the intraoperative anesthetic technique for major abdominal vascular surgery is not a critical determinant of ultimate outcome and that equivalent overall outcomes can be obtained with either a general or an epidural technique, as recently summarized by Norris (Table 32-6).53–57 The suggestion of benefit in the vascular subset of the VA trial50 is counterbalanced by the carefully controlled experiment of Norris et al, which included only vascular cases.41 The epidural technique may provide some benefit in the postoperative phase, principally in terms of improved patient comfort and a reduction in pulmonary complications.58,59 On the other hand, these studies support the greater importance of the implementation of a coordinated comprehensive care plan carried throughout the perioperative period.42
Infrainguinal Reconstructions
Anesthetic technique has also been studied in patients undergoing infrainguinal vascular surgery,60–64 but the data are less extensive and few firm conclusions can be drawn. In particular, no large-scale randomized, prospective, outcome-based studies can be used to guide anesthetic choice in this area. However, some data suggest that epidural anesthesia could have an impact on early infrainguinal bypass graft patency. Christopherson et al reported a prospective, randomized study in which general and epidural anesthesia was compared in 100 patients undergoing elective lower extremity bypass.64 Although the data showed no evidence of an effect of anesthetic method on mortality or major morbidity, these authors found an unexpected dramatic reduction in postoperative graft thrombosis from a high rate of 43% with general anesthesia to 8% with the epidural technique. Additional investigations by the authors suggested that this result could be due to an effect of epidural anesthesia on coagulation and fibrinolysis.65 This concept has been reviewed recently,66 but the data are mixed. An alternative explanation for this observation may be hemodynamic, related to enhancement of graft flow secondary to reduced peripheral resistance in the setting of epidural sympathetic block. This hypothesis gains some support with data from intermittent compression after bypass67 but remains unproven.
Carotid Surgery
The optimal mode of anesthesia for carotid surgery has been a topic of interest for decades, and numerous attempts have been made to definitively determine whether general anesthesia or local/regional techniques are superior.
Rerkasem et al68 reviewed the published work on this question in the Cochrane Database in 2004 and concluded that there were insufficient data at that time to draw clear conclusions of benefit. The General Anesthesia versus Local Anesthesia for Carotid Endarterectomy (GALA) Trial was designed to address this data deficit, and the original report and subsequent publications have provided some useful insights. GALA was a prospective, randomized, multicenter (95 centers in 24 countries, mostly in Europe) intention-to-treat analysis comparing general anesthesia with cervical block.68a A total of 3526 subjects (1753 subjects, general anesthesia; 1773 subjects, local anesthesia) were enrolled between June 1999 and October 2007. The primary outcome variable was composite 30-day stroke and myocardial infarction free survival. No significant difference was observed in the primary outcome between the two arms: 4.8% (84 patients) under general anesthesia and 4.5% (80 patients) under local anesthesia. Additionally, no differences were seen in the length of hospitalization or quality of life postoperatively. A subsequent publication by the GALA investigators suggested that local/regional anesthesia may be more cost-effective in patients in whom either anesthetic method is an option.68b Thus the best anesthetic technique for the most commonly performed vascular operation remains an open question. Given the low rate of major complications with carotid surgery today, it is unlikely that a definitive answer will be forthcoming. This topic is also discussed in Chapter 100.
hemodynamic monitoring
Patients undergoing vascular interventions harbor a host of medical comorbid conditions, and virtually all have at least some degree of cardiac compromise. The principal causes of perioperative mortality and major morbidity in vascular interventions are cardiac and include arrhythmia, myocardial ischemia, and congestive heart failure. Risk of these complications can be reduced by selection of the optimal mode of anesthesia, as discussed earlier. However, proper conduct of the anesthetic course and appropriate monitoring of the response of the patient to the intervention are critical to identify problems and allow both the surgeon and the anesthesiologist to recognize deviations from the expected course and adapt to the changing condition of the patient.
Electrocardiography
Continuous monitoring of the electrical activity of the heart is one of the most basic techniques and is applied throughout the perioperative period in almost all types of interventions. The principal rationale for electrocardiographic (ECG) monitoring is detection of arrhythmias and myocardial ischemia. Arrhythmias, common during vascular interventions, are related primarily to the choice of anesthetic agents, intraoperative variations in blood gases and serum electrolytes, direct autonomic stimulation, activation of cardiovascular reflexes (endotracheal intubation, vagal stimulation, peritoneal traction, sinus nerve), or direct stimulation of the myocardium (central venous cannulation, cardiac retraction). Myocardial ischemia is usually the result of underlying coronary heart disease combined with increased myocardial work secondary to the neuroendocrine responses to surgical trauma and hemodynamic conditions that may reduce global myocardial perfusion (hypovolemia).
A five-electrode system of the four limb leads and a single unipolar precordial lead (generally V5) is standard for most vascular procedures. This configuration allows monitoring of any of the standard six bipolar limb leads, as well as the anterolateral chest wall lead recording the anterior wall of the left ventricle in the distribution of the left anterior descending coronary artery. Leads II and V5 are monitored continuously in most vascular cases. Some evidence suggests that V3 or V4 may have greater sensitivity for detection of myocardial ischemia in this setting.69 Invasive leads (esophageal, endotracheal, or central venous) may have higher sensitivity for detection of arrhythmia and ischemia,70,71 but these are not used in routine clinical practice.
Pulse Oximetry
Pulse oximetry is widely applied in anesthesia practice and throughout the perioperative period today, but evidence supporting its efficacy or impact on outcomes in patients undergoing general anesthesia is scant.72,73 The potential benefits of intraoperative pulse oximetry are a continuous index of tissue perfusion and a reduction in the need for arterial blood gas measurement. Pulse oximetry is prone to a number of artifacts and may be particularly misleading in patients with vascular disease.74 Risk factors for discordant pulse oximetry include ASA physical status III, IV, or V; orthopedic, vascular, or cardiac surgery; and intraoperative hypothermia, hypotension, or hypertension. Furthermore, pulse oximetry cannot detect changes in PaO2 above 100 mm Hg and thus is insensitive to potentially clinically relevant changes in gas exchange in the lungs.
Capnography
Continuous monitoring of the end-tidal CO2 using infrared absorption spectroscopy is the standard of care for the assessment of ventilation during general anesthesia. This information is displayed most commonly as a continuous plot of the partial pressure of CO2 versus time (time capnogram). Exhaled gases can be sampled by either a sensor placed directly within the respiratory circuit (mainstream sensor, typically used in intubated patients) or a sensor placed remotely with gases aspirated via a sampling line (sidestream sensors, frequently used with nasal cannulas or face masks in patients breathing spontaneously). It is important to bear in mind that the gradient between end-tidal CO2 and PaCO2 (normally about 5 mm Hg) may be affected by disease states and that the measurement of end-tidal CO2 using capnography is subject to artifacts. The clinical applications of capnography have been reviewed recently.74a,74b In 2011, the ASA updated its statement on Standards for Basic Anesthetic Monitoring to recommend the addition of continuous monitoring of end-tidal CO2 during both moderate and deep sedation.74c This recommendation, although sensible, has aroused controversy because of the cost of monitoring equipment and the impact of these standards on regulators when applied to non–hospital-based treatment settings, such as dental offices and ambulatory procedural or interventional centers.74d
Arterial Pressure
Arterial pressure is the parameter of cardiovascular function most commonly monitored during anesthesia and surgery. The high incidence of cardiac disease in vascular patients and the dramatic effects of vascular procedures on afterload and blood volume make this parameter especially important in vascular interventions.
Noninvasive Methods
All patients should have periodic measurement of arterial pressure with noninvasive techniques throughout the perioperative period. Today, this is generally accomplished with automated oscillometric devices that use a single cuff and measure the amplitude of oscillations as the cuff is slowly deflated. The pressure at which oscillations are maximal is the mean arterial pressure, and systolic and diastolic pressures are calculated by various proprietary algorithms.75 Such devices correlate well with invasive arterial pressure measurements,76 although the decreased arterial compliance in older adults and in patients with diabetes may lead to overestimation of systolic pressure and underestimation of diastolic pressure. These errors are small and of no clinical significance for intraoperative applications. Automated oscillometry may be less useful in the setting of atrial fibrillation or bradycardia and in patients with severe vasoconstriction.
Invasive Methods
Real-time measurement of arterial pressure is extremely useful in the management of anesthesia during any major procedure with the potential for rapid blood loss or other perturbations of the circulation. This is particularly the case with modern major vascular surgery, which may entail sudden changes in afterload with aortic clamping and release, intentional pharmacologic manipulation of arterial pressure (hypotension during the thoracic endograft procedure, hypertension in occasional carotid cases), and manipulation of critical cardiovascular reflex arcs (with carotid angioplasty, endarterectomy, or both). In clinical practice today, intraarterial pressure is usually measured with a fluid-filled catheter, tubing, and an electromechanical pressure transducer system. Such systems generally have sufficient dynamic response to accurately measure intraarterial pressure, at least for clinical purposes. The accuracy of these systems is limited by the length and compliance of the tubing and the frequent presence of small air bubbles in the tubing. These problems lead to a decrease in the natural frequency and an increase in damping of the system, which typically falsely increase the recorded systolic arterial pressure. More accurate measurement of intraarterial pressure can be obtained with miniaturized transducers mounted on catheters. Such devices have several drawbacks, however, including an inability to recalibrate after insertion, high cost, and poor durability, and therefore are used primarily in research applications. The currently used fluid-filled systems have the ancillary benefit of the ability to repeatedly sample arterial blood, which allows additional monitoring capability.
The arterial cannula is most commonly inserted into the radial artery. Stenosis, thrombosis, and occlusion of the radial artery are frequent complications of cannulation, and ischemia of the hand can result.77 The ulnar artery is the dominant supply of arterial perfusion to the hand in approximately 90% of people.78 Assessment of the adequacy of collateralization through the ulnar artery by way of the Allen test is a critical step before safe use of the radial artery for monitoring pressure. Alternatively, monitoring catheters can be placed in the brachial, axillary, femoral, or lower extremity arteries. Femoral lines can be placed at the time of arterial exposure or cannulation for diagnostic or adjunctive arteriography and can be discontinued at the end of the procedure. Brachial catheters should be avoided because of the poor collateralization around the elbow in the event of thrombosis, and axillary lines may be complicated by axillary sheath hematoma, which can produce devastating neurologic consequences if not promptly identified and treated.
Central Venous/Pulmonary Artery Cannulation
Systemic arterial pressure is a derived variable in the circulation and is a physiologic result of the interaction of cardiac output and peripheral arterial resistance. Cardiac output is the product of heart rate and stroke volume, which is determined by myocardial contractility and left ventricular end diastolic volume (LVEDV). Central venous and pulmonary artery (PA) catheterization is performed to measure pressures that may have a useful relationship to left ventricular filling and thus to cardiac output.
Central venous catheterization is used to measure central venous pressure (CVP) and to theoretically provide a real-time estimate of intravascular volume and venous return, both of which are critical parameters of cardiac function. Unfortunately, CVP is affected by a large number of confounding variables, which limits its value, especially in vascular patients. CVP correlates well with left ventricular filling pressure only in patients with normal cardiac and pulmonary function. The correlations are further weakened with the use of positive pressure ventilation during surgery and by patient positioning. Furthermore, absolute values of CVP have little meaning, and changes in this parameter with intervention are more valuable. The ASA has recently published comprehensive practice guidelines to enhance the safety of central venous catheterization.78a
Some of these shortcomings may be overcome by the use of PA catheters. Basic flow-directed balloon-tipped PA catheters can be used to measure both pulmonary artery diastolic pressure and pulmonary artery wedge pressure (PCWP). These parameters are less affected by right heart events, and each is proportional to left ventricular end diastolic pressure (LVEDP). The left ventricular compliance curve defines the relationship between LVEDP and LVEDV. PA catheters also allow estimation of cardiac output by several different methods and permit sampling of mixed venous blood, which enables calculation of total body oxygen delivery (DO2) and total body oxygen consumption (VO2).
The PA catheter was developed to optimize cardiac function in critically ill patients and has been used extensively in the ICU and throughout the perioperative period in high-risk patients. This practice was the result of an observation by Shoemaker et al that the metrics of systemic perfusion and oxygen delivery (cardiac output and total body oxygen delivery) were closer correlates of survival than were more traditional cardiovascular parameters, such as heart rate, arterial pressure, and CVP.79–81 The PA catheter is the essential tool for implementing the strategy of hemodynamic optimization before elective major vascular surgery.82 This strategy involved admission to the ICU before planned elective high-risk vascular surgery, PA catheterization, fluid loading, and derivation of the Starling curve to develop personalized optimal cardiovascular parameters to be maintained during the perioperative period. This aggressive use of PA catheters was widely adopted with little prospective, outcomes-based data.
In 1996, Cooper et al presented a systematic review of the available published evidence and, among 34 studies reviewed, found only 1 with high-reliability data.83 Disturbing reports were also published suggesting that lack of understanding of cardiac physiology and proper use of PA catheters among ICU practitioners was a significant area of concern and limited the effectiveness of PA catheter–based interventions.84 In a widely recognized large, nonrandomized, prospective cohort study of a mixed medical and surgical ICU population, Connors et al reported higher mortality and cost in ICU patients treated with PA catheters.85 This report included data obtained from 5735 critically ill patients in whom a PA catheter was inserted within 24 hours of admission to the ICU in five teaching hospitals in the United States between 1989 and 1994. In this mixed medical and surgical ICU population, use of a PA catheter was associated with greater mean 30-day mortality (odds ratio, 1.24), longer mean ICU stay (14.8 days with a PA catheter and 13.0 without), and correspondingly greater mean cost ($49,000 with a PA catheter versus $35,700 without). Although this report has been criticized for potential selection bias in the placement of PA catheters, the work stimulated the performance of prospective, randomized studies with specific clinically relevant outcomes.
Since the 1996 review, several large prospective studies have suggested that the use of PA catheters in critically ill patients may offer little objective benefit,86,87 and this conclusion has been further supported by meta-analysis.88,89 A good example of the current state of research in this area is the report by Sandham et al.87 This prospective, randomized study was performed in 19 participating centers in Canada. The study recruited patients 60 years or older with ASA class III or IV risk (87.4% were ASA III) who were scheduled for abdominal, thoracic, major vascular (55.6% of enrolled patients), or hip surgery. The subjects in the standard-treatment group could have a central venous catheter placed (77% did) but not a PA catheter. The catheter group received a PA catheter and had directed intraoperative and ICU management to achieve an oxygen delivery of 550 to 600 mL/min/m2 body surface area, mean arterial pressure of 70 mm Hg, pulmonary artery wedge pressure of 18 mm Hg, heart rate of less than 120 beats/min, and hematocrit higher than 27%. The primary outcome was all-cause in-hospital mortality, and secondary outcomes were 6- and 12-month mortality and in-hospital morbidity. A total of 1994 patients were randomized (52% of those screened). Approximately 5% in each group did not receive the planned therapy, and 2.4% of the standard-therapy group received a PA catheter (crossover). Data analysis was on an intention-to-treat basis. The two groups were well matched in all measured parameters. No significant differences were noted between the groups in in-hospital mortality (7.7% with standard care versus 7.8% with a PA catheter), 6-month survival rate (88.1% with standard care versus 87.4% with a PA catheter), and 12-month survival rate (83.9% with standard care versus 83% with a PA catheter). In-hospital mortality was the same with the exception of a higher rate of pulmonary embolism in the PA catheter group (0.8% versus 0%). The principal physiologic goals for cardiac index and oxygen delivery were reached after surgery in 79% and 62.9% of patents in the PA catheter and standard-care groups, respectively. Data from the in-hospital portion of this study are shown in Table 32-7.87 Data such as these have settled this issue for elective major surgical patients with moderate preoperative risk (ASA III), but PA catheters may still have benefit in procedure-specific or generally higher-risk patients.
Several relatively large prospective studies evaluating the benefit of PA catheterization have been performed specifically to address this question in patients undergoing major vascular surgery,82,87,90–92 but little evidence of benefit, either independently or by meta-analysis, has been demonstrated.89 These data have been summarized recently in a comprehensive evidence-based review93 (Fig. 32-2). In brief, these authors conclude that the evidence is insufficient to recommend preoperative hemodynamic optimization for high-risk surgical patients and that there is no evidence of benefit for the routine use of PA catheters during high-risk general or vascular surgery. Guidelines from both the American College of Cardiology/American Heart Association94 and the ASA95 reflect these data and advise against the routine use of PA catheters in vascular surgery but suggest that these devices may be of some benefit in high-risk subsets. The advent of noninvasive methods for measurement of cardiac output, including transesophageal Doppler estimation of stroke volume, lithium dilution, and pulse contour cardiac output, may facilitate perioperative hemodynamic optimization in selected patients without the need for a PA catheter.96
Transesophageal Echocardiography
Transesophageal echocardiography (TEE) has become a mainstay of the intraoperative management of patients undergoing surgery on the heart and thoracic aorta.97,98 In this application, TEE can be used not only to monitor cardiac function in real time but also to provide information that may modify the conduct or extent of surgery. TEE provides real-time, two-dimensional gray-scale images of the cardiac chambers in a wide spectrum of projections, which allows optimal visualization of most areas of concern. In addition, pulsed, continuous wave, and color-flow Doppler capabilities allow physiologic measurement of flow and global cardiac dynamics. In particular, TEE can provide direct information on ventricular systolic function and its major components. The documented contribution of asymptomatic LV dysfunction to mortality in vascular surgery98a highlights the potential value of direct observation of myocardial function. Preload may be assessed by measurement of left ventricular dimensions at end diastole, generally by using a transgastric, mid–left ventricular short-axis view. Some data indicate that TEE-derived LVEDP correlates better with cardiac output than do PA catheter–derived data (PCWP).99 Myocardial contractility is best assessed in the ejection phase as the ejection fraction, which is calculated from two-dimensional data by standard formulas. Diastolic function (left ventricular relaxation) is assessed by pulsed Doppler examination of flow through the mitral value. Intraoperative TEE allows appropriate diagnosis of hemodynamic changes (e.g., hypovolemia versus reduced ejection fraction in hypotension) and provides prompt feedback on therapeutic interventions. Myocardial ischemia may be detected by early diastolic dysfunction and regional wall motion abnormalities.
Although the utility of intraoperative TEE in the management of major intracavitary vascular surgery has been described since the early report of Gewertz et al in 1987100 and has recently been reassessed,101,102 no published reports to date demonstrate an outcomes-based improvement with this modality. This may be related to the generally poor correlation among TEE findings of myocardial ischemia, ECG data, and eventual cardiac outcome.103,104 The ACC/AHA 2007 Guidelines on Perioperative Cardiovascular Evaluation and Care for Noncardiac Surgery suggest that intraoperative or perioperative TEE is reasonable for investigating acute, life-threatening hemodynamic disturbances.94,102
Neurologic Monitoring
Central Nervous System
Monitoring of central nervous system function is a critical component of strategies to optimize neurologic outcome with interventions on the carotid circulation. Regardless of whether surgery is performed with the patient awake or under anesthesia or with or without routine shunting, these procedures can be completed safely only with a clear assessment of the state of cerebral perfusion or function (or both). Placement of an intracarotid shunt does not guarantee that the shunt is functioning or that perfusion of the brain is satisfactory. The adequacy of cerebral perfusion has been assessed by a wide range of approaches ranging from conscious monitoring of the neurologic responses of the patient to continuous electroencephalography or transcranial Doppler measurements of flow in the middle cerebral artery. This topic is extensive and specialized, and a full discussion can be found in Chapter 100.
Spinal Nervous System
Monitoring of spinal nerve function is useful in procedures that involve possible disruption of perfusion to the spinal cord, most commonly through temporary clamping of thoracic aortic segments or ligation or exclusion of intercostal arteries giving rise to the great radicular artery. Such monitoring is generally accomplished with sensory evoked potentials to test the integrity of the spinal segments. This specialized topic is reviewed in Chapter 135.
Infection Control and Maintenance of Homeostasis
Perioperative Antibiotics
Surgical site infection (SSI) can complicate any invasive procedure. SSI has been estimated to account for at least 25% of all nosocomial infections in hospitals105,106 and to greatly increase mortality, length of hospital stay, and cost.105,107 Kirkland et al performed a 1 : 1 case-control study (225 pairs) in a community hospital in North Carolina and found that patients with SSI had twice the mortality rate (7.8% versus 3.5%), a 60% greater likelihood of requiring ICU care (29% versus 18%), and a six times greater incidence of readmission (41% versus 7%) than did matched controls.108 Unfortunately, these data are not unique.109–111 SSI in the setting of prosthetic vascular reconstruction may have devastating consequences (see Chapter 42).
Most cases of SSI are preventable. The basic principals and techniques of SSI prophylaxis were established in the 1960s112,113 and have been articulated and publicized in numerous reviews and guidelines.114–116 The fundamental requirement for effective SSI prophylaxis is establishment and maintenance of an effective concentration of an antibiotic suitable for the probable organisms in tissue at the site and time of inoculation (wounding). The critical aspect of timing of antibiotic administration is illustrated in the report of Classen et al.117 These investigators collected prospective data from 2847 patients undergoing elective clean or clean-contaminated surgery in a community hospital setting. A total of 1708 patients received SSI prophylaxis. When compared with the group that received antibiotics 2 hours or less before incision (0.6% incidence of SSI), those who were treated early (>2 hours before incision), perioperatively (within 3 hours after incision), and late (3 to 24 hours after incision) had relative risks of SSI of 6.7, 2.4, and 5.8, respectively.
Although surgeons and other health care providers are familiar with these concepts through training and practice, implementation of SSI prophylaxis has been poor.106,118 For example, as recently as 1996, Silver et al reported data from hospitals in New York State indicating that less than half of patients undergoing AAA repair in the sample were treated with perioperative antibiotics.110 The Centers for Disease Control and Prevention (CDC) published formal guidelines in 1999, and in 2002, the Centers for Medicare and Medicaid Services (CMS) and the CDC implemented the National Surgical Infection Project. The goal of the National Surgical Infection Project was to combine the resources and expertise of the CDC National Nosocomial Infection Surveillance System with that of the CMS network of state-based quality improvement organizations. The strategy was to reduce the incidence of SSI through three specific performance measures that would be part of a program of national surveillance and quality improvement. The specific measures are (1) the proportion of patients receiving parenteral SSI prophylaxis within 1 hour of incision, (2) the proportion of patients receiving SSI prophylaxis with an agent consistent with published guidelines, and (3) the proportion of patients whose prophylactic antimicrobial therapy is discontinued within 24 hours of completion of surgery. Compliance with these metrics was limited by inconsistencies among the published recommendations issued by various authorities, and in 2003 the Surgical Infection Prevention Guideline Writers Workgroup met and later released harmonized uniform guidelines.119 In 2004, the National Surgical Infection Project was incorporated into a more comprehensive surgical quality initiative, the Surgical Care Improvement Project (SCIP), and these measures have been added to a number of major quality initiatives and accreditation programs. According to the SCIP guidelines, the currently approved primary antibiotic agents for SSI prophylaxis during vascular procedures are cefazolin and cefuroxime. Vancomycin and clindamycin are appropriate substitutes in the event of β-lactam allergy.
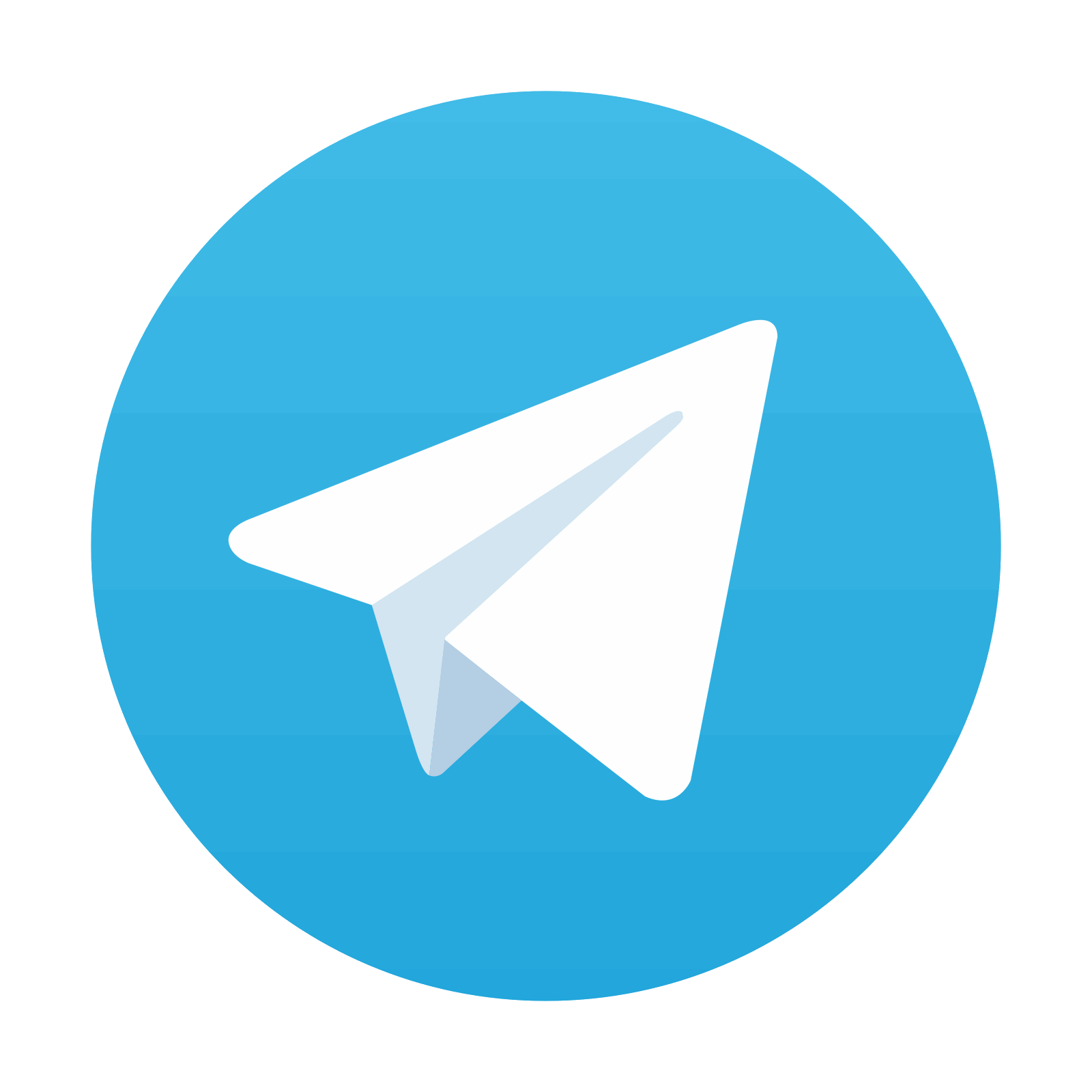
Stay updated, free articles. Join our Telegram channel

Full access? Get Clinical Tree
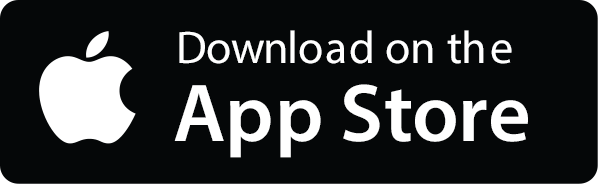
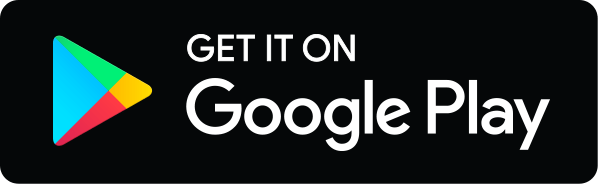