CHAPTER 46 Innovative Therapy and Technology
Lung cancer remains the number one cause of cancer-related deaths in men and women, and the incidence continues to rise with approximately 175,000 new cases diagnosed annually in the United States.1 In this chapter, we review several innovative therapies for the treatment of bronchogenic and metastatic lung tumors. Application of these new therapies continues to evolve, and in most cases, surgical resection remains the mainstay of therapy. However, most patients with non–small cell lung carcinoma (NSCLC) present with advanced disease, and many with early-stage disease are unable to tolerate pulmonary resection because of compromised cardiopulmonary function.2 The new alternatives to traditional surgical treatment allow additional options when surgery is not clearly of benefit or when the patient is not a candidate for traditional resection. Like other recent advances, such as videothoracoscopy, these new less-invasive approaches may allow surgeons to treat patients with thoracic malignant neoplasms even in the setting of marginal pulmonary reserve.3
RADIOFREQUENCY ABLATION
Radiofrequency ablation (RFA) uses high-frequency alternating current to heat and coagulate tissue. RFA systems have three components: (1) a generator, (2) an active electrode that is placed within the tumor, and (3) a dispersive electrode (Bovie pad) placed on the thighs of the patient (Fig. 46-1). As the radiofrequency energy moves from the active electrode to the dispersive electrode and back, ions within the tissue oscillate with the changing direction of the current, resulting in frictional heating of the tissue. As the temperature within the tissue rises to more than 60°C, cell death occurs because of protein denaturation and coagulation necrosis. Moreover, the dispersion of energy beyond the vicinity of the active electrode is minimal, allowing focused delivery of energy to the target lesion with minimal injury to the surrounding normal tissue. The goal of RFA in the treatment of lung tumors is complete coagulation necrosis of the lesion with a small rim of extension into adjacent normal pulmonary parenchyma.
Most experience with RFA has been in the treatment of liver tumors, both as an adjunct to resection and as primary therapy. Because of lower complication rates, RFA has supplanted other less-invasive modalities (e.g., cryotherapy).4,5 In a large international multicenter study of 2320 patients who underwent percutaneous RFA for malignant liver neoplasms, mortality was 0.3%, and the overall complication rate was 7.1%.6
Several centers around the world have published reports demonstrating the safety and feasibility of RFA for lung tumors.7,8 Animal models, to investigate the feasibility of RFA for the treatment of lung tumors, have been used to develop treatment algorithms for humans. In a study by Goldberg and colleagues9 with a rabbit model of lung sarcoma, seven lesions were treated with RFA for 6 minutes at 90°C, and the remaining four tumors were left untreated as controls. The authors noted computed tomography (CT) evidence of coagulation necrosis surrounding the tumor, manifested radiographically by increased opacity enveloping the lesion. This was followed temporally by the development of central tissue attenuation consistent with cavitation. Histologic analysis revealed that at least 95% of the tumor nodules were necrotic, although some rabbits (43%) had residual tumor nests at the periphery of the tumor. Pneumothorax was the only procedure-related complication, occurring in 29% of treated rabbits and 25% of controls. In another study, Miao and colleagues10 implanted VX2 sarcomas into the lungs of 18 rabbits (12 treated and 6 controls). The lesions were then treated with RFA for 60 seconds with a cooled-tip electrode. Absolute tumor eradication was achieved in 33% of the rabbits. A partial response was observed in 41.6% of rabbits that survived longer than 3 months. On histopathologic evaluation, the ablated lesions retained their fundamental tissue architecture with evidence of coagulation necrosis. Surrounding edema and inflammation were noted in the normal adjacent pulmonary parenchyma. One to 3 months after treatment, the ablated tumor became an atrophied nodule of coagulation necrosis within a fibrotic capsule.
The timing and progression of these postablation changes become important issues in evaluating postprocedure treatment response in patients with pulmonary tumors. Some investigators have performed RFA followed by resection to evaluate the efficacy of the ablation procedure. In one multicenter study of 15 patients, ablation was possible in 13 cases.11 In these 13 patients, median tumor kill was 70%, with seven patients achieving 100% ablation. Five of the final six cases exhibited 100% ablation, suggesting mastery of the learning curve for the technique. Nguyen and colleagues12 published the results of an ablate-and-resect study in which vital immunohistochemical stains were used to assess tumor cell viability. In seven tumors studied with this technique, more than 80% nonviability was documented. Three patients (38%) demonstrated 100% nonviability. All three tumors were less than 2 cm in diameter. A single ablation with either a 3- or 3.5-cm active electrode was performed, such that the larger tumors may have been inadequately ablated. More recently, Schneider and colleagues13 reported their series of ablate-and-resect pulmonary metastasectomies. Eighteen patients underwent RFA and subsequent thoracotomy and resection for 18 diverse solid tumor metastases. Lesion size ranged from 0.7 to 2.5 cm. The resected specimens were evaluated for tumor viability with immunohistochemistry. Complete ablation was achieved in 39%. More than 90% ablation was achieved in 50% of the resected lesions, and the authors considered this to be a successful ablation, asserting that subsequent growth of the residual lesion (had it not been resected) was unlikely. An incomplete ablation was documented in the remaining 11%. These studies demonstrate that although RFA can produce effective ablation, 100% tumor cell death is not universally achieved, and resection remains the treatment of choice for those able to tolerate surgical intervention.
Currently, three U.S. Food and Drug Administration–approved devices are available for the performance of RFA: Boston Scientific (Boston, MA), RITA (Mountain View, CA), and Valley Laboratory (Boulder City, CO). The Boston Scientific device is an impedance-based system in which the endpoint of treatment is determined by a significant rise in tissue impedance, indicating an inability of the target lesion to maintain further conduction and, therefore, ablation. The RITA and Valley Laboratory systems are temperature-based devices that elevate the tumor temperature to predetermined lethal levels for a designated time. The Boston Scientific and RITA active probes both consist of an expandable needle system (Fig. 46-2 and 46-3); the Valley Laboratory system consists of either a single needle or three parallel needles (Fig. 46-4) that are placed within the tumor. The Valley Laboratory (VL) electrode consists of a proximal insulated portion and a distal uninsulated active tip. The electrode is irrigated with a continuous infusion of cold saline and for this reason is sometimes referred to as a cool-tip electrode. The dissolved saline ions enhance conduction and therefore, theoretically, decrease the required time to achieve effective ablation.
Two animal studies comparing the relative efficacy of the various probes report disparate results. Pereira and colleagues14 reported significantly better ablation lengths and volumes with the VL cool-tip probes compared with the expandable probes in a pig model of RFA. Conversely, Denys and coworkers15 achieved larger volumes and more spherical ablation zones with the expandable probes compared with the VL cool-tip probe. A recent Japanese report of RFA for treatment of 342 pulmonary tumors in 128 patients cites tumor size greater than 2 cm and use of the single-needle VL probe to be associated with local progression on multivariate analysis. The single-needle VL probe was used for 195 of the ablation procedures, the Boston Scientific probe for 142; five of the procedures were performed with the VL cluster probes.16
Patient Selection
For NSCLC, RFA should be reserved for patients diagnosed as stage I who are believed to be at increased risk for pulmonary resection or for those who refuse surgery. On occasion, RFA may be a reasonable therapy for a medically inoperable patient with more advanced cancer (e.g., satellite nodules) localized to the lung.17 The efficacy of RFA is low for nodules of more than 5 cm in diameter. RFA is also not recommended for lesions abutting the mediastinum.18 Other patients who may be considered for treatment with RFA include those with advanced-stage disease who have responded to definitive radiation therapy and chemotherapy but have a persistent solitary peripheral focus of cancer and those who present with a recurrent isolated cancer after previous lung resection. RFA is also a suitable option for some patients with limited peripheral pulmonary metastases. Like resection, this treatment should be reserved for those patients with a limited number of metastases, disease localized to the chest, or controlled or controllable primary sites and only for those patients who are believed to be at increased operative risk for resection of their pulmonary metastases. In some situations, complete resection of all pulmonary metastases is not possible, and RFA can be used as an adjunct intraoperatively. We have certainly found RFA to be of use in the situation in which a wedge resection of a peripheral nodule was performed and resection of other nodules would have required a lobectomy or pneumonectomy. To preserve pulmonary parenchyma, some of these tumors were treated with intraoperative RFA through open thoracotomy. Table 46-1 outlines suggested selection criteria for the use of RFA.
Table 46–1 Selection Criteria for Radiofrequency Ablation
Inclusion Criteria | Exclusion Criteria |
---|---|
NSCLC stage I or II∗; poor surgical candidate | Tumor abutting hilum or large pulmonary vessel |
NSCLC stage IIIB (satellite nodule in same lobe) or stage IV (nodule in another lobe or lung); poor surgical candidate | Malignant effusion |
Stage IIIA or IV with solitary pulmonary nodule remaining after standard therapies | Pulmonary hypertension |
Pulmonary metastases; primary disease controlled or controllable; poor surgical candidate | More than three tumors in one lung |
Target lesion ≤5 cm | Target lesion >5 cm |
NSCLC, non–small cell lung carcinoma.
∗ Patients with stage II should receive additional therapy because N1 disease will not be treated with radiofrequency ablation.
Operative Technique
The most common method of pulmonary RFA entails percutaneous CT-guided administration. Either general or local anesthesia can be used in performing CT-guided RFA. Our preference has been to use general anesthesia.19 This allows needle deployment, ablation, and biopsies (if required) to be performed in a more controlled manner. In addition, some patients with cardiopulmonary compromise may have difficulty maintaining optimal body position if awake or sedated. Positioning of the patient is extremely important during CT-guided RFA. We prefer to position the patient in such a way that the target lesion is accessed with minimal penetration of normal lung parenchyma. This decreases the risks of hemorrhage and prolonged air leak. Positioning is also important to ensure adequate clearance of the RFA probe within the scanner. The maximum number of lesions that can be ablated in a single setting is debatable. As a general rule, however, ablation of more than three lesions in one setting is not recommended.
Treatment Response
RFA incites an inflammatory response that may persist for up to 3 months, making it difficult to determine radiographically whether the mass represents scar or viable cancer. The mass may initially appear larger on radiographic imaging and then subsequently decrease in size. Ablated lesions may demonstrate central cavitation (Fig. 46-5) or develop bubble lucencies, both of which are radiographic indicators of effective ablation. Other centers have used CT densitometry protocols to evaluate for persistent or recurrent disease.20 Densitometry involves the injection of contrast material and subsequent CT images of the ablated nodule at 0, 45, 180, and 300 seconds after the injection of contrast material. Lesions larger than 9 mm that enhance to 15 Hounsfield units or more after 1 minute are suggestive of cancer. These densitometry techniques are time-consuming and typically valuable only for those patients with single tumor nodules. We have modified the Response Evaluation Criteria in Solid Tumors to evaluate treatment responses objectively (Table 46-2).18 CT scans are obtained at 3-month intervals to assess lesion size and characteristics. Whenever possible, positron emission tomography (PET) scans are also obtained to aid in the determination of tumor response. The American College of Surgeons Oncology Group is accruing patients in a multicenter study evaluating RFA in high-risk patients with stage IA NSCLC. This study (Z4033) will address such issues as response assessment by standardizing the follow-up protocol used by the various study sites. Follow-up assessment will include CT (size criteria and densitometry) and serial PET scans.
Clinical Results
In our initial experience with RFA at the University of Pittsburgh, we treated 33 tumors in 18 patients.18 Tumors included metastatic carcinoma (n = 8), sarcoma (n = 5), and NSCLC (n = 5). The mean age was 60 years (range, 27 to 95 years). Our principal finding was the lack of effectiveness in treating tumors larger than 5 cm. Using the Response Evaluation Criteria in Solid Tumors, we found that a radiographically determined response rate of 66% was seen for tumors of 5 cm or less compared with only 33% in patients with tumors larger than 5 cm. A multicenter study summarizing the results of 493 patients undergoing RFA of pulmonary nodules concluded that RFA is safe, with negligible morbidity and mortality (0.4%), and is associated with a gain in quality of life.21 We have also reported the results of RFA in a series of 18 patients with NSCLC.22 A total of 21 tumors were treated. Most patients (n = 9) had stage I NSCLC. There were four patients with stage IV cancer, including three patients with recurrences after previous lobectomy and one patient with a synchronous liver metastasis that was also treated with RFA. The median tumor diameter was 2.8 cm (range, 1.2 to 4.5 cm). Morbidity was minor in most cases but occurred in 55.6% of patients. The most common complication was pneumothorax (38.9%), which resolved with pigtail catheter drainage within 24 hours in most cases. At a median follow-up of 14 months, 83.3% of the patients were alive. Local progression occurred in 38.1% of the ablated nodules. For stage I cancer, the mean progression-free interval was 17.6 months; the median progression-free interval was not reached. Although this is a small study, these results compare favorably with standard methods of external beam radiation, in which local progression is seen in more than 50% of cases.23
More recently, we updated our experience with RFA for the treatment of stage I NSCLC.24 Nineteen patients underwent RFA during a 3-year period. Median age was 78 years (range, 68 to 88 years). Initial complete response was observed in two patients (10.5%), partial response in 10 (53%), and stable disease in five (26%). Early progression occurred in two patients (10.5%). During follow-up, local progression occurred in eight nodules (42%), and the median time to progression was 27 months. There was no procedure-related mortality, although six deaths occurred during follow-up. The median follow-up in the remaining patients was 23 months. The probability of survival at 1 year was estimated to be 95% (95% confidence interval [CI], 0.68-0.91), and the median survival was not reached.
Lanuti and colleagues25 have recently published their midterm results of a series of 31 nonsurgical patients with NSCLC who underwent a total of 38 percutaneous RFA treatments. There were no 30-day mortalities. Five patients developed pneumothorax; three required chest tubes. Six patients developed pneumonia within 4 weeks of treatment; all resolved with oral antibiotics. There were eight cases of postprocedure pleural effusion. These are typical morbidities. The authors note, however, one patient who developed transient laryngeal nerve injury after ablation of a left upper lobe lesion abutting the mediastinum. We reiterate that RFA should not be applied to central lesions. Tumor size ranged from 0.8 to 4.4 cm. Local recurrence was assessed by CT and PET and found in 31.5% of all treated tumors but in 50% of tumors larger than 3.0 cm, 44.4% of tumors 2.0 to 3.0 cm, and 21.7% of tumors smaller than 2.0 cm. Survival at median follow-up of 17 months was 74%, and overall survival was 30 months.
The RAPTURE study26 was a prospective, intention-to-treat, single-arm, multicenter, international clinical trial designed to validate the feasibility and safety of RFA for pulmonary tumors as well as to assess efficacy; 106 patients with 183 tumors were treated with 137 RFA treatments. All patients were deemed to be nonsurgical candidates. The authors reported a 99% technical success rate. There were no mortalities. Pneumothorax requiring chest tube evacuation developed in 27 patients. Pleural effusions occurred in 15 patients, four requiring drainage. Tumor sizes ranged from 0.5 to 3.4 cm. Pulmonary function was assessed before the procedure and then at 1, 3, 6, and 12 months after the procedure and was not significantly changed. Of the 85 patients who could be assessed for tumor response, 88% exhibited complete response to treatment at 1 year. Local progression was noted in 12%. Overall survival was 70%, and cancer-specific survival was 92% at 1 year.
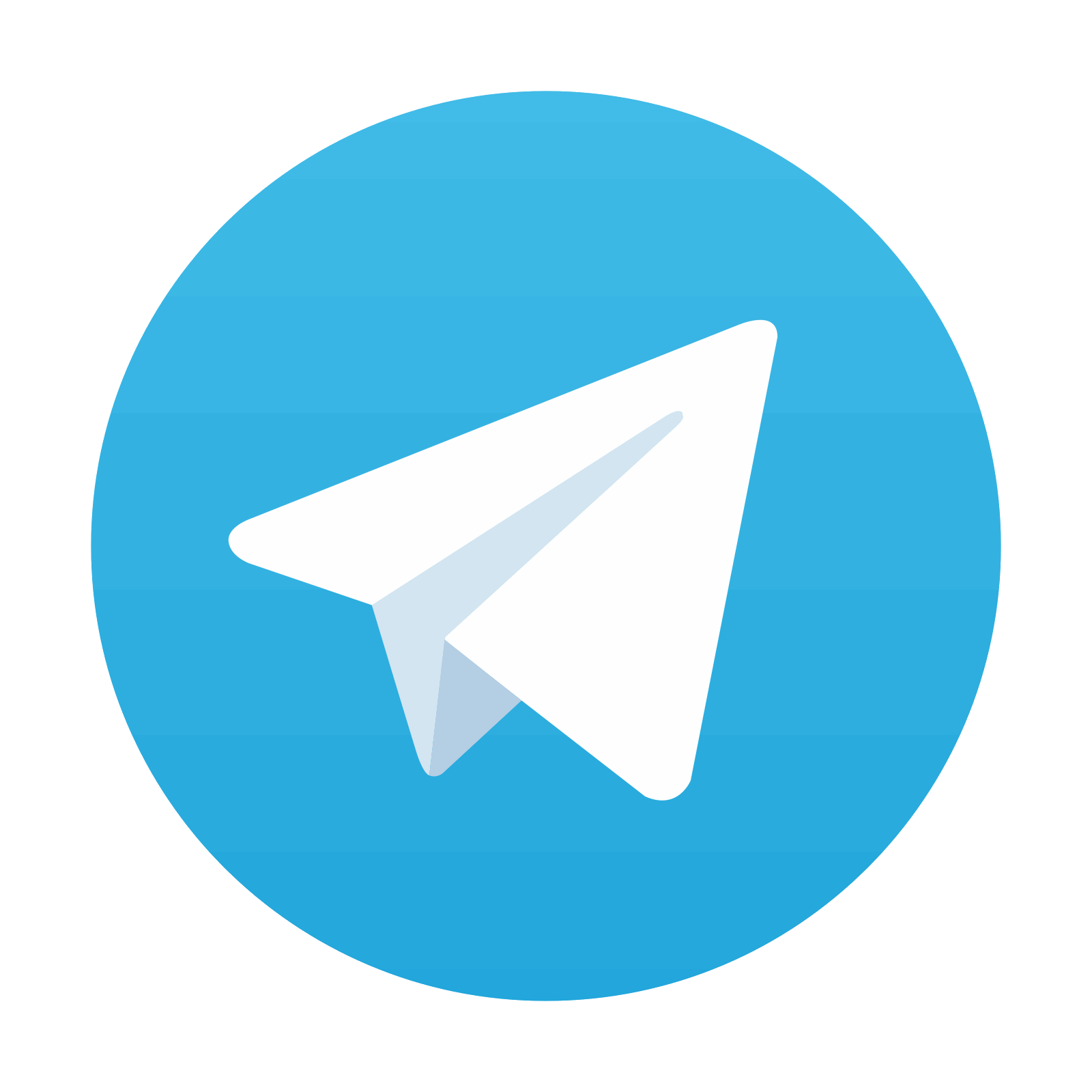
Stay updated, free articles. Join our Telegram channel

Full access? Get Clinical Tree
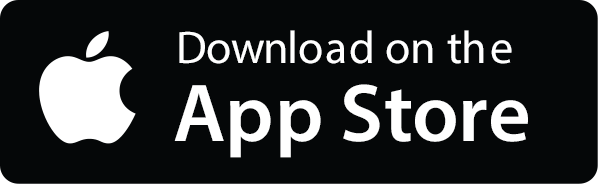
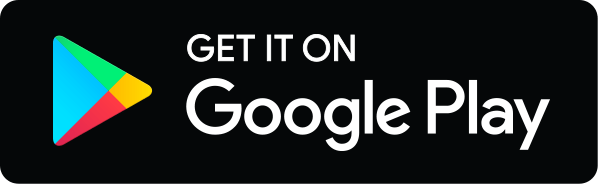