Chapter 11 Influence of Diabetes Mellitus on Vascular Disease and Its Complications
Diabetes mellitus is a heterogeneous collection of syndromes characterized by hyperglycemia. It is described in the Ebers Papyrus, an Egyptian text dating to approximately 1550 bc. Nevertheless, despite being recognized for four millennia, the disease in many ways remains poorly undefined. It is estimated to affect approximately 26 million inhabitants of the United States with total estimated costs in the United States in 2007 of $174 billion. More than 60% of nontraumatic lower-limb amputations occur in people with diabetes.1 With new criteria for diagnosis,2 the prevalence of diabetes may be even higher. Owing to the high prevalence of diabetes mellitus in patients with peripheral arterial disease, screening for this disorder is recommended for all patients in vascular wards who do not already carry the diagnosis.3
IDDM, or the type characterized by low or undetectable insulin secretion and a need for insulin administration to sustain life, is generally accepted as primarily autoimmune in etiology.4 The genetic vulnerabilities and the environmental insults that elicit IDDM are under intense study but remain poorly characterized. The complexity of these interactions is illustrated by the fact that less than 50% of monozygotic twins of a diabetic proband develop IDDM. NIDDM is a highly concordant genetic disease, with nearly 100% of monozygotic twins of a diabetic proband acquiring the disease. The concordance for first- and second-degree relatives increases given the challenge of obesity. NIDDM subjects tend to have insulin levels in the normal to high range yet remain insulin resistant, with an inadequate ability to secrete insulin in a manner sufficient to lower blood glucose into the normal range.5
Clinical practice guidelines are published yearly by the American Diabetes Association.1 These guidelines define diabetes as an AIC level (glycated hemoglobin) greater than 6.5%, a fasting glucose higher than 126 mg/dL, a 2-hour glucose greater than 200 mg/dL on an oral glucose tolerance test (or at random in a symptomatic patient). Prediabetes is defined as a fasting glucose level of 100 to 125 mg/dL.
Persons with either IDDM or NIDDM tend to be at especially high risk for the development of vascular disease, in part because they have glucose toxicity as a result of elevated blood glucose levels, insulin toxicity as a result of elevated insulin levels from peripheral insulin injection (IDDM and some NIDDM) or elevated endogenous insulin secretion (most NIDDM), and a persistent inflammatory state. The nature of these toxicities is described in some detail later. This chapter also emphasizes the importance of minimizing these two toxicities through therapeutic approaches that target normal glucose levels without unduly raising insulin levels or promoting hypoglycemia.6
The recognition of vascular disease as an obligatory concomitant of diabetes mellitus occurred during the twentieth century. Osler noted in 1908 that “the thickening of the arteries in . . . diabetes . . . may be due to the action on the blood vessels of poisons retained within the system.”7 In the 1945 edition of his textbook, 20 years after the use of insulin had become common, Joslin noted that “arteriosclerosis in the form of gangrene of the lower extremities has decreased while at the same time it has increased in the heart as coronary disease and in the brain as apoplexy.”8
It is now generally agreed that diabetes mellitus accelerates the initiation and propagation of vascular disease. At present, 84% of diabetic subjects who live longer than 20 years after diagnosis have some form of vascular disease, and 75% of persons with diabetes die of vascular disease or its complications, primarily myocardial infarction and stroke.9 Thus persons with diabetes, regardless of type, have an increased risk for disease of the large and small vessels. In addition, the distribution of large vessel disease is more diffuse.
Diabetes remains problematic for the vascular surgeon for several reasons. The clinical diagnosis of vascular disease in a person with diabetes may be confounded by the presence of sensorimotor polyneuropathy and multiple sites of disease, which may mask typical pain or pulse patterns. The outcome of surgical intervention tends to be less favorable in persons with diabetes because the hospital stay is generally longer, the risk for infection is greater, and the probability of an adverse outcome is higher than in persons without diabetes. In the 1960s, mortality rates in diabetic patients who had surgery were reported to be 3.6% to 13.2%.10 In 1983, Hjortrup and colleagues11 studied morbidity in diabetic and nondiabetic patients who had major vascular surgery and found that there were no deaths in either group and comparable morbidity. Although their theories were unproved, the authors hypothesized that the improvement in statistical outcome in the intervening 20 years was due to improvements in diabetes care. A recent study of the 25-year cumulative incidence of lower extremity amputations in people with type 1 diabetes would appear to confirm the hypotheses raised previously. The cumulative incidence within the Wisconsin Epidemiologic Study of Diabetic Retinopathy participants (n = 943) was still high at 10.1%. Being male, heavy smoking, hypertension, diabetic retinopathy, neuropathy, and higher hemoglobin (Hb) A1c were independently associated with the incidence of lower extremity amputation. Thus increased attention to the modifiable factors of smoking, blood pressure, and glucose control is warranted.12
There appears to be little doubt that diabetes care enhances the outcome of perioperative infection. From 1990 to 1995, Golden and coworkers13 at Johns Hopkins evaluated 411 adults with diabetes who underwent coronary artery surgery, conducting glucose surveillance six times per day. After adjusting for age, sex, race, underlying comorbidity, acute severity of illness, and length of stay in the surgical intensive care unit, patients with higher mean glucose readings were at increased risk of developing infections. Thus the investigators concluded that in patients with diabetes who undergo coronary artery surgery, postoperative hyperglycemia is an independent predictor of short-term infectious complications.
Cerebrovascular, Cardiovascular, and Peripheral Vascular Disease and Diabetes
A number of studies documented that the glucose level at the time of hospital admission is a predictor of outcome and extent of neurologic deficit in persons with acute stroke. For example, Toni and associates14 attempted to identify predictors and possible pathogenic mechanisms of early neurologic deterioration in patients with acute ischemic strokes and to evaluate their effect on clinical outcome. They studied a continuous series of 152 patients with first-ever ischemic hemispheric strokes who were hospitalized within 5 hours of onset, evaluated according to the Canadian Neurological Scale, and assessed with a computed tomography (CT) scan. The initial subset of 80 patients also underwent angiography. A repeated CT scan or autopsy was performed within 5 to 9 days of a patient’s stroke. Progressing neurologic deficit was defined as a decrease of 1 point or more in the global neurologic scale score during hospitalization compared with the score at entry. Those whose condition deteriorated had been hospitalized earlier and had higher serum glucose levels at admission.
The Oslo Study also found that diabetes and the level of nonfasting glucose predicted the outcome of stroke.15 That study, started in 1972, included 16,209 men aged 40 to 49 years. Of these, 16,172 had no previous history of stroke and 151 were known to have diabetes. Five diabetic and 80 nondiabetic subjects died of stroke during the 18 years of follow-up, giving a rate ratio of 7.87 (95% confidence interval [CI], 2.48 to 19.14) for diabetic subjects. The rate of mortality for all causes in diabetic subjects was more than fivefold that of those who were not diabetic. Nonfasting serum glucose was a predictor of fatal stroke in all participants (diabetic subjects included) without a history of stroke in age-adjusted univariate analysis. The relative risk (RR) was 1.13 (95% CI, 1.03 to 1.25) by an increase of 1 mmol/L (18 mg/dL) of serum glucose, according to results of proportional hazards regression analysis.
Similar observations were made in Scotland, where women were found to be more vulnerable to the effects of glucose than were men.16 Sex-specific CVD, ischemic heart disease, and stroke mortality rates and relative risks for asymptomatic hyperglycemic subjects (top 5%) were compared with those of normoglycemic individuals (bottom 95%) during a mean follow-up of 11.6 years (range, 10 to 14 years) of 4696 men and 5714 women in western Scotland aged 45 to 64 years at entry. Univariate analysis showed that asymptomatic hyperglycemia was associated with increased risk of all causes of CVD, ischemic heart disease, and stroke mortality in both men and women. The degree of this association was greater in women than in men. Using multiple logistic regression analysis to take into account differences in age, systolic and diastolic blood pressure, serum cholesterol, body mass index (BMI), and cigarette smoking, a high causal blood glucose level was still a significant risk factor for CVD mortality in both men and women.
Within the diabetic population, the level of complications such as retinopathy, preexisting nephropathy, and coronary and peripheral vascular disease, in addition to age, is a predictor of outcome.17 In a cohort of 2124 diabetic persons identified at multiphasic health checkups from 1979 through 1985, 56 suffered a nonembolic ischemic stroke during the follow-up period, which extended through 1991. For each case subject, one diabetic control subject, matched by sex and year of birth, was selected from the same cohort of diabetic subjects. The estimated relative risk of stroke in diabetic subjects with retinopathy was 2.8 (95% CI, 1.2 to 6.9). After adjusting for age, sex, smoking, use of insulin, average systolic blood pressure, and average random glucose level, the estimated relative risk was 4.0 (95% CI, 1.0 to 14.5). The relative risk of stroke in diabetic subjects with retinopathy remained elevated after the exclusion of those with complications other than retinopathy.
The Honolulu Heart Program also confirmed a poorer prognosis for vascular disease associated with hyperglycemia.18 This study examined the association between a variety of baseline lifestyle and biologic factors in a middle-aged cohort of Japanese American men and the 20-year incidence rates of total atherosclerotic end points and each of the initial clinical manifestations of this disease, including fatal and nonfatal coronary heart disease, angina pectoris, thromboembolic stroke, and aortic aneurysm. Japanese American men (n = 2710) between the ages of 55 and 64 years at the time of the initial clinical examination (1965 through 1968) who had no evidence of coronary heart disease, cerebrovascular disease, cancer, or aortic aneurysm were studied. Among these men, 602 atherosclerotic events developed during the 23-year follow-up period (1965 through 1988). After adjusting for each of the baseline characteristics examined, significant positive associations between quartile cutoffs of BMI, systolic blood pressure, serum glucose levels, cholesterol, triglycerides, and uric acid, as well as cigarette smoking, and the occurrence of any atherosclerotic end point were noted.
Within the diabetic population, nonfatal or small infarction, especially with multiple occurrences, is a feature of cerebrovascular disease complicating diabetes mellitus and correlates with elevated blood glucose and blood pressure.19 Asymptomatic cerebral infarction is not rare in diabetic subjects and can now be pathologically and clinically evaluated with accuracy using magnetic resonance imaging.
The Wisconsin Epidemiologic Study also confirmed the finding of elevated risk for vascular disease in the diabetic population.20 The association of glycemia with cause-specific mortality in a diabetic population was studied in a cohort design based in a primary care setting. All participants (n = 1210) had diabetes, were taking insulin, and received a diagnosis when they were younger than 30 years. They were compared with a random sample of diabetic persons with a diagnosis at 30 years or older (n = 1780). Thus, both IDDM and NIDDM were studied, although the National Diabetes Data Group criteria for diagnosis were not used.21 Glycosylated hemoglobin levels were obtained at baseline examinations. Median follow-up was 10 years in patients with an earlier onset and 8.3 years in those with a later onset. The main outcome measure was cause-specific mortality determined from death certificates. In the early-onset group, after controlling for other risk factors in proportional hazard models and considering the underlying cause of death, the glycosylated hemoglobin level as an index of average glucose control was significantly associated with mortality from diabetes (hazard ratio [HR] for a 1% change in glycosylated hemoglobin, 1.25; 95% CI, 1.13 to 1.38) and ischemic heart disease (HR, 1.18; 95% CI, 1.00 to 1.40). In the later-onset group, glycosylated hemoglobin was significantly associated with mortality from diabetes (HR, 1.32; 95% CI, 1.21 to 1.43), ischemic heart disease (HR, 1.10; 95% CI, 1.04 to 1.17), and stroke (HR, 1.17; 95% CI, 1.05 to 1.30), but not cancer (HR, 0.99; 95% CI, 0.88 to 1.10). The authors concluded that “these results suggest . . . benefit to the control of glycemia with respect to death due to vascular disease and diabetes.”21
The Copenhagen Stroke Study also found that diabetes is a risk factor for stroke and that diabetes influences the nature of stroke.22 The study evaluated stroke type, stroke severity, prognosis, and the relation between admission glucose levels and stroke severity and mortality. This community-based study included 1135 acute stroke patients, of whom 233 (20%) had diabetes. All patients were evaluated until the end of rehabilitation by weekly assessment of neurologic deficits (Scandinavian Stroke Scale) and functional disabilities (Barthel index). A CT scan was performed in 83% of stroke cases. The diabetic stroke patient was 3.2 years younger than the nondiabetic stroke patient (p < 0.001) and had hypertension more frequently (48% vs. 30%; p < 0.0001). Intracerebral hemorrhages were six times less frequent in diabetic patients (p = 0.002). Initial stroke severity, lesion size, and site were comparable between the two groups; mortality was higher in diabetic patients (24% vs. 17%; p = 0.03), and diabetes independently increased the relative death risk by 1.8 (95% CI, 1.04 to 3.19). Outcome was comparable in surviving patients with and without diabetes, but patients with diabetes recovered more slowly. Mortality increased with higher glucose levels on admission in nondiabetic patients, independent of stroke severity (odds ratio [OR], 1.2 per 1 mmol/L; 95% CI, 1.01 to 1.42; p = 0.04). Thus, diabetes influences stroke in terms of age, subtype, speed of recovery, and mortality. The authors concluded that “the effect of reducing high admission glucose levels in nondiabetic stroke patients should be examined in future trials.”22
A Finnish study, although of short duration, confirmed the excess risk of elderly diabetic women for acute stroke.23 The study examined whether NIDDM, its metabolic control and duration, and insulin level predict stroke. Cardiovascular risk factors, including glucose tolerance, plasma insulin, and glycosylated hemoglobin, were determined in a Finnish cohort of 1298 subjects aged 65 to 74 years, and the effects of these risk factors on the incidence of both fatal and nonfatal stroke was investigated during 3.5 years of follow-up. Of 1298 subjects participating in the baseline study, 1069 did not have diabetes and 229 had NIDDM. During the 3.5-year follow-up, 3.4% (n = 36) of nondiabetic subjects and 6.1% (n = 14) of NIDDM subjects had a nonfatal or fatal stroke. The incidence of stroke was significantly higher in diabetic women compared with nondiabetic women (OR, 2.25; 95% CI, 1.65 to 3.06). In multivariate logistic regression analyses including all study subjects, fasting and 2-hour glucose (p < 0.01 and p < 0.05, respectively), glycosylated Hb A1c (p < 0.01), atrial fibrillation (p < 0.05), hypertension (p < 0.05), and previous stroke (p < 0.01) predicted stroke events. In diabetic subjects, fasting and 2-hour glucose (p < 0.01 and p < 0.05, respectively), glycosylated Hb A1c (p < 0.05), duration of diabetes (p < 0.05), and atrial fibrillation (p < 0.05) were the baseline variables predicting stroke events. Finally, fasting insulin (p < 0.05), hypertension (p < 0.05), and previous stroke (p < 0.01) were associated with stroke incidence in nondiabetic subjects.
The longer-term Honolulu Heart Program confirmed that, for men, diabetes confers extra risk of thromboembolic stroke but not hemorrhagic stroke.24 The goal of this study was to determine whether glucose intolerance and diabetes increase the risk of thromboembolic, hemorrhagic, and total stroke, independent of other risk factors. Among the 7549 Japanese American men aged 45 to 68 years and free of coronary heart disease and stroke from 1965 to 1968, a total of 374 thromboembolic, 128 hemorrhagic, and 36 type-unknown strokes occurred. The incidence of thromboembolic but not hemorrhagic stroke increased with the worsening glucose tolerance category. Compared with the low-normal (glucose <151 mg/dL) group, subjects in the high-normal (151 to 224 mg/dL), asymptomatic high (≥225 mg/dL), and known diabetes groups all had significantly elevated age-adjusted relative risks of thromboembolic stroke. After adjusting for other risk factors, relative risks remained significantly elevated for the asymptomatic high and known diabetes groups (RR, 1.43 and 2.45; 95% CI, 1.00 to 2.04 and 1.73 to 3.47, respectively). Associations were the same in hypertensive and nonhypertensive subjects and similar but slightly stronger in younger (45 to 54 years) than in older (55 to 68 years) men.
The Northern Manhattan Stroke Study found that an admission blood glucose level greater than 140 mg/dL was an important predictor of mortality in stroke.25 Ethanol abuse (RR, 2.5), hypertension requiring discharge medications (RR, 1.6), and elevated blood glucose within 48 hours of index ischemic stroke (RR, 1.2/50 mg/dL) were found to be independent predictors of recurrence.
This review emphasizes that the relationship between glucose and vascular disease appears to hold across various cultures and genetic backgrounds. In a study in Taiwan of 479 NIDDM patients 40 years or older from four community primary care health centers, cholesterol, high-density lipoprotein cholesterol, plasma glucose, and Hb A1c were studied.26 The duration of diabetes was associated with the development of stroke, with an RR of 1.063 for every 1-year increment (p < 0.07). Significant risk factors were serum cholesterol and Hb A1c levels. For every 1-mg/dL increase in mean total cholesterol level, the relative risk of developing vascular disease increased 1.016-fold (p < 0.04). For every 1% (approximately 35 mg/dL) increase in Hb A1c, the relative risk of developing vascular disease increased 1.170-fold (p < 0.01). Female diabetic subjects had a higher relative risk than male subjects did. The risk of CVD is therefore twofold to threefold higher in diabetic than nondiabetic subjects. There is a gender difference; the incidence is twofold higher in diabetic men and threefold times higher in diabetic women.27–30
In the nondiabetic group in the Honolulu Heart Program, there was a dose-response relation between glucose intolerance at baseline and coronary heart disease incidence, coronary heart disease mortality, and total mortality. This risk was independent of other risk factors in this cohort of 8006 middle-aged and older Japanese American men.31 Therefore, even in the normal range, glucose levels predict risk for vascular disease. A more recent study confirms these conclusions.32 The Atherosclerosis Risk in Communities (ARIC) also documented that Hb A1c levels in the normal range predict diabetes incidence, heart disease events, stroke, and death over more than a decade in both African Americans and white adult subjects.
Persons with diabetes have shorter life spans. Approximately 75% of increased mortality in men and 50% in women is caused by CVD. Kleinman and colleagues27 found that the RR of mortality from ischemic heart disease was 2.8 for men and 2.5 for women after controlling for other confounding variables of hypertension, obesity, age, serum cholesterol, and smoking. In a Utah population, CVD accounted for 48% of all-cause mortality in diabetic subjects.28 Diabetic subjects do not have only an increased mortality from acute myocardial infarction; they also have an increased rate of congestive heart failure, cardiogenic shock, and dysrhythmias, not necessarily correlated with the size of the infarct.30,33 It is thought that the increased rate of congestive heart failure is secondary to hypocontractility, which may be due to microvascular disease, the metabolic effects of diabetes leading to cardiomyopathy, and autonomic dysfunction.33
Disease of the large vessels in one area of the vascular tree appears to predict disease in other areas. Associations of vascular disease are also found with perturbations in coagulation and inflammation.34 Heinrich and coworkers investigated the vessel status of coronary and peripheral arteries and those arteries supplying the brain in 929 consecutive male patients admitted to a coronary rehabilitation unit.34 The severity of coronary atherosclerosis was scored using coronary angiography. Changes in extracranial brain vessels and manifest CVD were determined by B-mode ultrasound and Doppler examination. Peripheral arterial disease was diagnosed using baseline and stress oscillography. There was a significant increase in plasma fibrinogen, plasminogen, D-dimer, and C-reactive protein with increasing severity of coronary heart disease. Compared with men who had unaffected arteries, men with three diseased coronary arteries had 58% greater D-dimer concentrations. Patients with cerebrovascular disease and peripheral vascular disease also had significantly higher fibrinogen, D-dimer, and C-reactive protein concentrations.
Many of the vascular lesions may also be asymptomatic, emphasizing the potential role of prophylactic screening for vascular lesions in other parts of the vascular tree when an index lesion is identified in a diabetic subject.34 In a prospective population-based study of Dutch white inhabitants between 50 and 75 years of age, 2484 subjects were screened with respect to glucose tolerance. A group of 173 people with diabetes and a representative age- and sex-stratified sample of 288 nondiabetic subjects were studied in the vascular laboratory. Carotid artery disease was investigated with duplex scanning, and arm and leg artery obstructions were evaluated with real-time frequency analysis of continuous-wave Doppler signals and indirect blood pressure measurements. Comparing diabetic with nondiabetic subjects, the authors found significantly more obstructions of the carotid arteries (8.7% vs. 2.8%), arm arteries (2.3% vs. 0%), and leg arteries (31.8% vs. 18.4%). This was also true only if crural artery obstructions were compared (23.7% vs. 16.0%). More than half the subjects with carotid artery obstructions also had leg artery obstructions.35
The same group investigated the cross-sectional association between peripheral arterial disease and glycemic level, age, sex, and glucose tolerance.36 The prevalence rates of ankle-brachial index (ABI) less than 0.90 were 7.0%, 9.5%, 15.1%, and 20.9% in normal glucose-tolerant, impaired glucose-tolerant, NIDDM, and known diabetic subjects under treatment, respectively (chi-square test for linear trend, p < 0.01). Prevalence rates of any peripheral arterial disease (ABI < 0.90, at least one monophasic or absent Doppler flow curve, or vascular surgery) were 18.1%, 22.4%, 29.2%, and 41.8% in these categories (chi-square test for linear trend, p < 0.0001). Logistic regression analyses showed that any arterial disease was significantly associated with Hb A1c, fasting, and 2-hour postload plasma glucose after correction for cardiovascular risk factors (OR, 1.35, 1.20, and 1.06, respectively; 95% CI, 1.10 to 1.65, 1.06 to 1.36, and 1.01 to 1.12, respectively). These authors did not find an association between insulin levels and vascular disease.
Diabetes-related peripheral vascular disease remains a huge public health problem.37 Although rates of lower extremity amputation and arterial reconstruction declined from 1983 to 1992, by 1996, the rate of major amputation had increased 10.6% since 1979. The earlier 12-year decline was positively correlated with reductions in the prevalence of smoking, hypertension, and heart disease, but not diabetes.38
Diabetic subjects with peripheral vascular disease are more likely to have small vessel disease of the foot, as well as large vessel disease elsewhere.39 These findings may contribute to the higher risk for the development of chronic foot ulcers in diabetic patients with peripheral vascular disease. Other independent predictors of amputation include sensory neuropathy and foot ulcers.40
Doppler studies using the ABI appear to be as useful in diabetic as in nondiabetic populations for identifying vascular disease, as documented by the Cardiovascular Health Study of 5084 participants.41 Risk factors associated with an ABI of less than 1.0 in multivariate analysis included smoking (OR, 2.55), history of diabetes (OR, 3.84), increasing age (OR, 1.54), and nonwhite race (OR, 2.36). In the 3372 participants free of clinical coronary vascular disease, other noninvasive measures of subclinical CVD, including carotid stenosis by duplex scanning, segmental wall motion abnormalities by echocardiogram, and major electrocardiographic abnormalities, were inversely related to the ABI (all p < 0.01). Therefore, the lower the ABI, the greater the increase in CVD risk; however, even those with modest, asymptomatic reductions in the ABI (0.8 to 1.0) had an increased risk of coronary vascular disease. The ABI Collaboration confirmed these earlier observations.42 This metaanalysis of 16 cohort populations comprised 24,955 men and 24,339 women. A low ABI (<0.90) predicted vastly increased risks of 10-year cardiovascular mortality in both genders.
The risk for amputation in diabetic subjects appears to parallel the risk for vascular disease in general.43 A case-control study was conducted among 10,068 patients from a large health maintenance organization at a multiphasic health checkup between 1964 and 1984, with an average length of follow-up after baseline of 13.2 years. Case patients were 150 cohort members with a first nontraumatic lower extremity amputation after baseline. Control subjects were 278 cohort members who did not experience an amputation during follow-up, matched to patients by age, sex, and year of baseline. Level of glucose control (p < 0.0001), duration of diabetes (p = 0.04), and baseline systolic blood pressure (p = 0.004) were independent predictors of amputation, as were microvascular complications (retinopathy, neuropathy, and nephropathy). The observation that type of diabetes (or genetic background) did not predict amputation but that glycemia was predictive lends credence to the “glucose toxicity” hypothesis of vascular risk.
Clinical Studies of Intervention
As noted earlier, there seems to be a consensus that there is a relationship between glucose levels and cardiovascular events that shows a dose response within both normal and diabetic populations.44 There have been several intervention studies that tested the glucose toxicity hypothesis, as well as studies of blood pressure control and lipid control in patients with diabetes mellitus. Table 11-1 summarizes the results of prospective glucose-lowering trials and CVD in people with diabetes mellitus. As can be seen, a significant risk reduction was demonstrated in seven of the eight published trials in type 1 and type 2 diabetes.
A Stockholm study provided convincing evidence that control of glycemia prevents microvascular complications in IDDM.45 The Diabetes Control and Complications Trial (DCCT) clearly confirmed that tight control decreased the incidence of microvascular complications in IDDM.46 The DCCT showed a trend of decreased incidence of CVD, but the result was not statistically significant. The DCCT was not designed primarily to test the hypothesis that blood glucose control would influence the risk for CVD. The patients were too young and it was too early in the course of their diabetes to expect significant cardiovascular event rates. Nevertheless, 17 initial major cardiovascular events were recorded: 14 in the conventional treatment group and 3 in the intensive treatment group.47 Total major cardiovascular and peripheral vascular events numbered 40 in the conventional group, compared with 23 in the intensive group. Thus, with intensive treatment, the risk for cardiac events was reduced by 78%, and the risk for combined cardiac and peripheral vascular events was reduced by 42%. As noted earlier, these risk differences did not achieve the defined limits for statistical significance. In contrast, a metaanalysis published in 1999 clearly shows that intervention with intensive glucose control has a beneficial effect on the incidence of the first and any cardiovascular event in type 1 patients (see Table 11-1).48
As summarized in Table 11-1,41–54 the results in persons with type 2 diabetes are even more convincing. Of particular note is the Diabetes and Insulin Glucose Infusion in Acute Myocardial Infarction study, which documented that acute management with intense insulin treatment at the time of myocardial infarction with subsequent insulin therapy has a significant effect on mortality at 1 year. The 1998 publication of the United Kingdom Prospective Diabetes Study reinforced the clinical goal of obtaining Hb A1c values equal to or less than 7% in these patients.
Earlier studies of peripheral vascular risk in IDDM show that an intensive program of glucose control documented by glycosylated hemoglobin and multiple daily blood glucose self-monitoring measures can reverse lesions of the red blood cell, polymorphonuclear leukocyte, platelet, and fluid phase of coagulation associated with diabetes.55–59 In addition, basement membrane thickening, nerve conduction, and the ABI were found to improve after an 11-month program of intensive glucose control and exercise. In view of the association between the ABI and the risk in diabetic subjects noted earlier, these studies still provide some of the most compelling evidence for programs of glucose control and exercise in persons with diabetes to avoid or even facilitate the reversal of large vessel disease. The goal is to aggressively treat elevated blood glucose and blood pressure and abnormal lipid profiles.
Table 11-2 summarizes the major lipid-lowering trials in patients with type 2 diabetes.60–63 There are three primary randomized trials for primary prevention and an equal number for secondary prevention of CVD. Metaanalysis of these trials shows an overall relative risk reduction of 55% for primary prevention and 29% for secondary prevention. The Scandinavian Simvastatin Survival Study also documented the cost-effectiveness of lipid lowering. In persons with coronary heart disease with normal fasting glucose levels, simvastatin reduced the average cost of CVD-related hospitalization by $3585, which offset 60% of the cost of drug. For those with impaired fasting glucose levels, average CVD-related hospitalization costs were reduced by $4478, which offset 74% of the drug cost. For diabetic subjects, there was a net cost savings of $1801 per subject. Current American Diabetes Association guidelines recommend low-density lipoprotein cholesterol targets of less than 2.59 mmol/L (100 mg/dL) for diabetic subjects with one additional cardiovascular risk factor and an intervention level of 3.36 mmol/L (130 mg/dL), with a target of 2.59 mmol/L for all other subjects with diabetes.56 As shown by the Long-Term Intervention with Pravastatin in Ischemic Disease Study, patients appear to benefit from statin therapy over a wide range of initial lipid levels.
Table 11-3 summarizes the trials of blood pressure lowering in persons with type 2 diabetes.64–66 As emphasized in a 1998 Cochrane Library review, primary intervention trials indicate a treatment benefit for coronary vascular disease, but not for total mortality in people with diabetes.66a For both short- and long-term secondary prevention, there is a benefit for total mortality in persons with diabetes. Most of the published data from randomized, controlled trials of antihypertensive therapy in diabetes for all-cause mortality and CVD outcomes are taken from hypertension trials that are not specific to diabetes.
It has been suggested that it is cost-effective to treat all patients with type 2 diabetes with angiotensin-converting enzyme inhibitors.65 This approach is reinforced by the publication of the Heart Outcomes Prevention Evaluation, a placebo-controlled study of more than 9000 subjects that indicated that ramipril substantially lowers the risk of death, heart attack, stroke, coronary revascularization, heart failure, and complications related to diabetes mellitus in a high-risk group of patients with preexisting vascular disease. The results are remarkable both for the magnitude of the treatment effect (an overall reduction of 22% in the primary outcome of myocardial infarction, stroke, or death from cardiovascular causes) and for the rather small reduction (3.2 mm Hg) in blood pressure. The authors also noted a marked reduction in the incidence of complications related to diabetes and new cases of diabetes in those taking ramipril.
There has been less enthusiasm for calcium channel blockers in persons with diabetes.66 Nevertheless, there is evidence that they can be effective as well, as shown in Table 11-3.
Subsequent trials in patients with type 2 diabetes have been designed to examine the role of intensive glycemic control on cardiovascular outcomes have focused on optimal targets of Hb A1c, including levels less than 7%. The Veterans Affairs Diabetes Trial (VADT showed significant reductions in albuminuria with median Hb A1c of 6.9% compared with standard glycemic control in retinopathy and neuropathy.67,68 The Action in Diabetes and Vascular Disease Preterax and Diamicron Modified Release Controlled Evaluation (ADVANCE) study of intensive versus standard glycemic control in type 2 diabetes found a statistically significant reduction in albuminuria with a target Hb Q1c of 6.5%.69 The Action to Control Cardiovascular Risk in Diabetes (ACCORD) trial showed lower rates of microvascular complications in the intensive arm compared with the standard arm.70,71 Early reports from ACCORD of a potential increase in mortality in the intensive care subgroup that could not meet target A1c goals have generated controversies that have yet to be resolved. In the interim, an Hb A1c target of 7% remains the consensus.72
Evidence for the Influence of Glucose on the Pathophysiology of Vascular Disease
Hyperglycemia is associated with vascular disease, as documented earlier. The reasons for this association remain speculative. Box 11-1 documents some of the hypothesized means by which glucose might influence pathologic vascular changes. These factors are discussed here in some detail.
Box 11-1 Glucose Toxicity Hypothesis
Hyperglycemia Initiates or Propagates Vascular Disease by Multiple Mechanisms
• Glycation of proteins and genetic material leading to dysfunctional or toxic products
• Interference with the fluid, vascular, and platelet phases of coagulation
• Perturbations in oxidation-reduction pathways
• Production of abnormal lipid metabolism
• Vascular volume shifts associated with changes in glycemia, or intracellular osmotic shifts associated with alternative metabolic pathways invoked when glucose is elevated, are toxic to the vascular tree
• Abnormal insulin or proinsulin levels in response to hyperglycemia contribute to vascular disease
• Perturbations in the immune system, including lymphokine production and polymorphonuclear leukocyte function, contribute to vascular disease
Glycation and Advanced Glycation End Products, or Early and Late Maillard Reactions
In 1976, it became clear that a minor hemoglobin component, Hb A1c, resulted from a posttranslational modification of hemoglobin A by glucose and that there was a clinical relationship between Hb A1c and fasting plasma glucose, the peak on the glucose tolerance test, the area under the curve on the glucose tolerance test, and mean glucose levels over the preceding weeks.73–76 It soon became apparent that an improvement in ambient blood glucose levels resulted in correction of Hb A1c levels and that these nonenzymatic glycosylation reactions might provide a hypothesis that could explain a number of the pathologic sequelae of diabetes mellitus via toxicity arising from glucose adduct formation with proteins or nucleic acids.77
As early as 1912, Maillard78 suggested that the chemical reactions that now bear his name might play a role in the pathologic changes associated with diabetes mellitus. The ability of reducing sugars to react with the amino groups of proteins is now widely recognized, as is the natural occurrence of many nonenzymatically glycosylated proteins.
The initial step (or early Maillard reaction) involves the condensation of an amino moiety with the aldehyde form of a particular sugar. Only a small fraction of most common sugars is normally present in the aldehyde form.78,79 A number of transformations are possible after the addition of an amine to a sugar carbonyl group. Considerable evidence supports the involvement of an Amadori-type rearrangement for the adduct of glucose with the N-terminal of the β chain of hemoglobin. The labile Schiff base aldimine adduct is transformed into a relatively stable ketoamine adduct via the Amadori rearrangement.
Because hemoglobin circulates in its red blood cell for approximately 120 days, there is little opportunity in this cell for late Maillard reactions, or nonenzymatic browning, to occur. In these late Maillard reactions, the Amadori product is degraded into deoxyglucosones that react again with free amino groups to form chromophores, fluorophores, and protein cross-links.80,81 In tissues that are longer lived, these reactions may be important mediators of diabetic changes as well as the aging process. Although the structure of a large number of nonenzymatic browning products has been elucidated, few have been obtained under physiologic conditions, thus making detection in vivo difficult and their pathologic role uncertain.82 Box 11-2 summarizes some of the observations and hypotheses whereby glycation might promote pathologic changes in persons with elevated blood glucose.
Box 11-2
Hypotheses Regarding the Potential Role of Nonenzymatic Glycation and Browning in the Pathologic Changes Associated with Diabetes Mellitus
V. Potentiation of other diseases of postsynthetic protein modification
The Maillard reaction is ubiquitous in nature. The accumulation of advanced glycation end products (AGEs) in tissues in the human body has been implicated in the complications of diabetes, aging, and renal failure. The links between these reactions and the pathogenesis of nephropathy, macroangiopathy, microangiopathy, and cataracts in diabetic subjects are increasingly strong.83,84 There is a growing body of evidence supporting a connection between circulating and tissue-accumulated AGEs, their receptors, and diabetic complications.
Coagulation Factors
Platelet
The platelet, when obtained from patients with diabetes mellitus, has long been recognized as showing abnormal behavior in in vitro and in vivo studies.58,59 In general, the correction of hyperglycemia is associated with an improvement in platelet behavior and release. The potential role of the platelet in vascular disease in general is discussed elsewhere in this book. The lesion of the platelet associated with hyperglycemia appears to be related to a hypersensitivity to stimuli. Thus, platelet aggregation in vitro may occur spontaneously by stirring in plasma obtained from persons with Hb A1c greater than 10%, with concomitant release of vasoactive substances including serotonin, adenosine diphosphate, and prostaglandins. The increased functional properties of diabetic platelets result in part from the primary release of larger platelets with enhanced thromboxane formation capacity and increased numbers of the functional glycoprotein (GP) receptors GP Ib and GP IIb/IIIa, which are synthesized in megakaryocytes.85 Insulin exerts an antiaggregating effect, but that effect is diminished in obese patients and in subjects with NIDDM.86 Increased platelet aggregation to arachidonic acid has also been linked to reduced antioxidant properties seen in persons with diabetes.87
Platelet-rich or fibrin clots are less amenable to lysis in patients with diabetes than in controls.88 Furthermore, the release of platelet plasminogen activator inhibitor-1 (PAI-1) in whole blood has been found to be increased in NIDDM subjects.89 PAI-1 levels have been noted to decrease with the lowering of blood glucose in NIDDM90; therefore the platelet contributes not only to a prethrombotic state in persons with diabetes, but also to problems of clot lysis in hyperglycemic subjects.
The Evaluation of Platelet IIb/IIIa Inhibitor for Stenting Trial substudy is the most extensive evaluation of stenting and platelet IIb/IIIa blockade in persons with diabetes and provides additional evidence for the role of the platelet in the morbidity and mortality of heart disease and the associated processes in diabetes.91 The trial involved 491 diabetic patients who were divided into three groups: the first group received both a stent and abciximab, the second group underwent balloon angioplasty and also received the drug, and the third group had a stent implanted but received only a placebo. The reblockage rate was cut in half in the patients who received both the stent and the drug. Those patients had an 8.1% reblockage rate in the 6 months after the procedure, which was about half that of the other two groups. Ongoing trials of eptifibatide and tirofiban should help to determine whether platelet IIb/IIIa receptor blockers should be used routinely to reduce restenosis after stenting in diabetic subjects, as well as the role of activated platelets and endothelium in pathologic conditions. These agents will become more attractive, in part because of the report of thrombotic thrombocytopenic purpura associated with ticlopidine in the setting of coronary artery stents.92
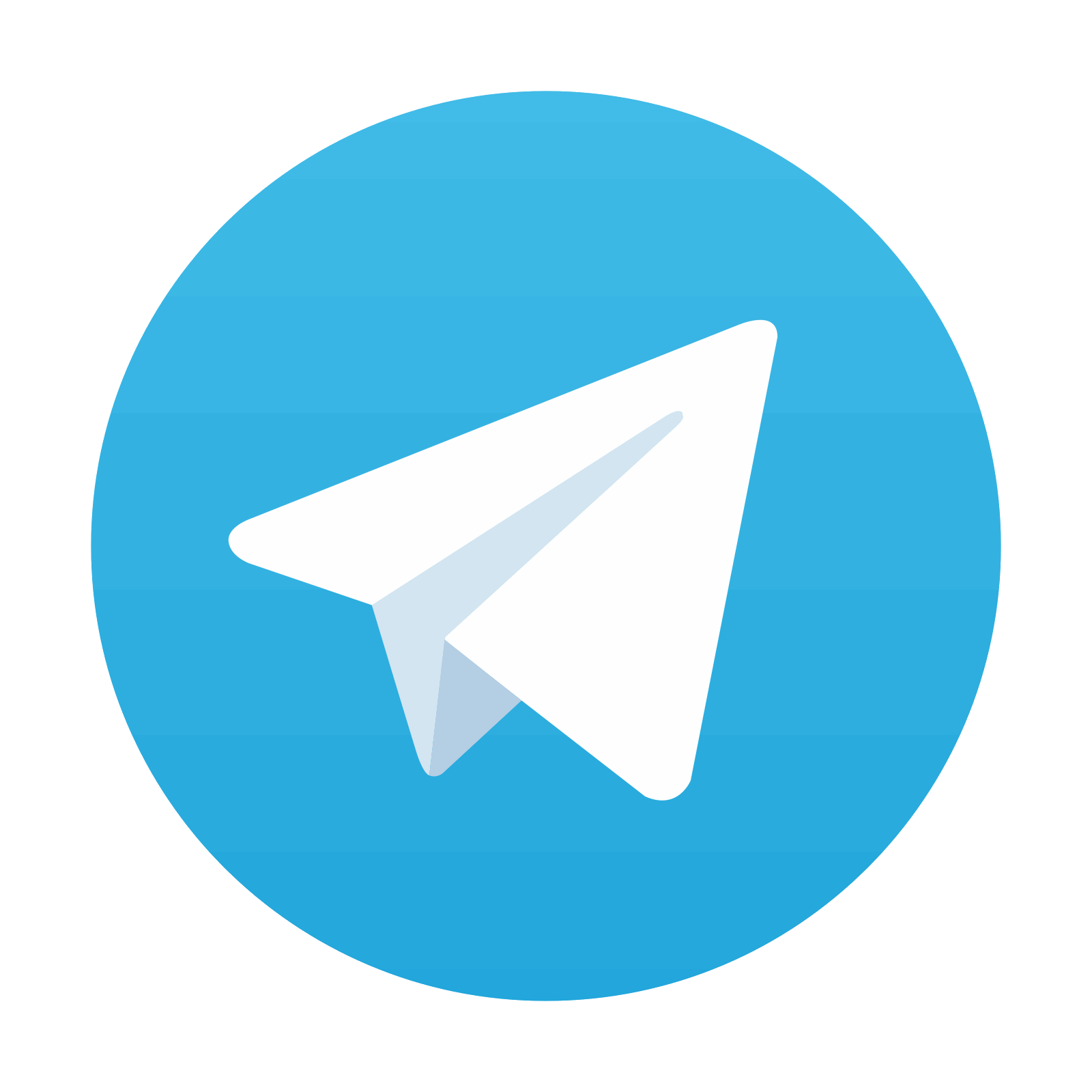
Stay updated, free articles. Join our Telegram channel

Full access? Get Clinical Tree
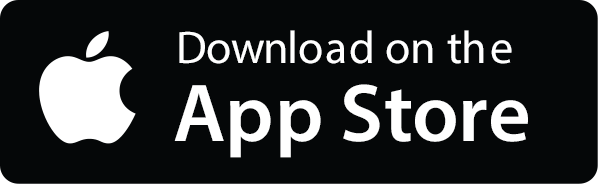
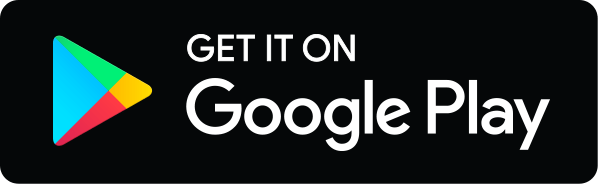