The impact of left ventricular (LV) dyssynchrony after acute myocardial infarction (AMI) on LV ejection fraction (EF) is unknown. One hundred twenty-nine patients with a first ST-elevation AMI (58 ± 11 years, 78% men) and QRS duration <120 ms were included. All patients underwent primary percutaneous coronary intervention. Real-time 3-dimensional echocardiography and myocardial contrast echocardiography were performed to assess LV function, LV dyssynchrony, and infarct size. LV dyssynchrony was defined as the SD of the time to reach the minimum systolic volume for 16 LV segments, expressed in percent cardiac cycle (systolic dyssynchrony index [SDI]). Myocardial perfusion at myocardial contrast echocardiography was scored (1 = normal/homogenous; 2 = decreased/patchy; 3 = minimal/absent) using a 16-segment model; a myocardial perfusion index, expressing infarct size, was derived by summing segmental contrast scores and dividing by the number of segments. SDI in patients with AMI was 5.24 ± 2.23% compared to 2.02 ± 0.70% of controls (p <0.001). Patients with AMI and LVEF <45% had significantly higher SDI compared to patients with LVEF ≥45% (4.29 ± 1.44 vs 6.95 ± 2.40, p <0.001). At multivariate analysis, SDI was independently related to LVEF; in addition, the impact of SDI on LV systolic function was incremental to infarct size and anterior location of AMI (F change 16.9, p <0.001). In conclusion, LV synchronicity is significantly impaired soon after AMI. LV dyssynchrony is related to LVEF and has an additional detrimental effect on LV function, beyond infarct size and the anterior location of AMI.
Advances in echocardiographic techniques, i.e., tissue Doppler echocardiography, speckle-tracking echocardiography, and real-time 3-dimensional echocardiography (RT3DE) have recently demonstrated an impaired left ventricular (LV) synchronicity in patients with acute myocardial infarction (AMI). In the setting of chronic heart failure, LV dyssynchrony is a phenomenon extensively described and related to impaired LV systolic function and poor prognosis. In the setting of AMI, however, it is unclear whether LV dyssynchrony is independently associated with LV ejection fraction (EF). Moreover, the detrimental effect of LV dyssynchrony on LV systolic function in addition to other variables remains unknown. Accordingly, the aim of the present study was twofold: (1) to assess the decrease in LV synchronicity after AMI (compared to normal values) and (2) to explore the relation between this decrease in LV synchronicity and LV systolic function. In particular, the effect of LV dyssynchrony on LVEF in addition to other variables was assessed.
Methods
The population consisted of 159 consecutive patients admitted to the coronary care unit because of a first ST-segment elevation AMI. Patients with a QRS complex duration >120 ms were excluded from the study.
The diagnosis of AMI was made based on typical electrocardiographic changes and/or ischemic chest pain associated with increased cardiac biomarkers. All patients underwent immediate coronary angiography and primary percutaneous coronary intervention (PCI). The infarct-related artery was identified by the site of coronary occlusion during coronary angiography and electrocardiographic criteria. During PCI, the final Thrombolysis In Myocardial Infarction flow was assessed. In addition, time from onset of symptoms to first balloon dilatation (symptoms-to-balloon time) was determined.
Using the electrocardiograms acquired on admission and 1 hour after PCI, the ST-segment resolution was assessed, as previously described. The sum of ST-segment elevations was measured 60 ms after the J point in leads I, aVL, and V 1 to V 6 for anterior AMI and leads II, III, aVF, V 5 , and V 6 for nonanterior AMI. Percent resolution of ST-segment elevation from before to after PCI was then calculated.
RT3DE was performed 48 hours after PCI to assess global LV systolic function and LV dyssynchrony; immediately after RT3DE, myocardial contrast echocardiography was performed to assess infarct size. These echocardiographic examinations are part of the routine, comprehensive assessment of patients presenting with AMI in our clinics.
In addition, 30 subjects without evidence of structural heart disease and without known risk factors for coronary artery disease, matched for age, gender, and body surface area, who underwent RT3DE, were included as a control group. These subjects were derived from the echocardiographic database and were clinically referred for echocardiographic evaluation because of atypical chest pain, palpitations, or syncope without murmur.
To determine impairment of LV synchronicity after AMI, patient data were compared to data from controls. In addition, the relation between LV systolic function and other clinical and echocardiographic variables (including LV dyssynchrony, infarct size, and infarct location) was evaluated.
Patients underwent imaging in the left lateral decubitus position with a commercially available system (Vivid 7, GE Healthcare, Horten, Norway) equipped with a 3V phased array transducer (2.5 MHz). Apical full volume 3D datasets were acquired in harmonic mode, integrating, during a brief breath-hold, 8 R-wave–triggered subvolumes into a larger pyramidal volume (90° by 90°) with a complete capture of the left ventricle. The 3D datasets were digitally stored for off-line analysis.
Off-line analysis was performed by an observer who had no knowledge of patients’ identity and myocardial contrast echocardiographic results. Dedicated software (4D LV-Analysis; TomTec, Munich, Germany) was used. The algorithm used by the software to calculate LV end-diastolic volume, LV end-systolic volume, and LVEF is described in detail elsewhere. Briefly, a semiautomated method for the detection of the apical 4-chamber view and the 60° and 120° incremental views and for the tracing of the endocardial border in the entire 3D dataset (including LV trabeculations and papillary muscles within the LV volume) were used. Subsequently, a final reconstruction of the LV model was generated and LV volumes and LVEF were obtained. In addition, the same LV model was used for the assessment of LV dyssynchrony, as previously described. Briefly, the LV model was automatically divided in 16 pyramidal subvolumes (6 basal segments, 6 mid segments, and 4 apical segments) based around a nonfixed central point. For each volumetric segment, the time–volume curve for the entire cardiac cycle was derived and the time taken to reach the minimum systolic volume was calculated. The SD of the time taken to reach the minimum systolic volume expressed as percent cardiac cycle (systolic dyssynchrony index [SDI]) was then calculated as a marker of global LV dyssynchrony.
Immediately after RT3DE, myocardial contrast echocardiography was performed to evaluate myocardial perfusion, to assess infarct size after AMI. The same ultrasound system equipped with a 3.5-MHz transducer was used. The 3 standard apical views were acquired using a low-power technique (mechanical index 0.10 to 0.26). Background gains were set so that minimal tissue signal was seen, and the focus was set at the level of the mitral valve. Luminity (Bristol-Myers Squibb Pharma, Brussels, Belgium) was used as contrast agent. Each patient received an infusion of 1.3 ml of echocardiographic contrast diluted in 0.9% sodium chloride solution 50 ml through a 20-gauge intravenous catheter in a proximal forearm vein. Infusion rate was initially set at 4.0 ml/min and then titrated to achieve optimal myocardial enhancement without attenuation artifacts. Machine settings were optimized to obtain the best possible myocardial opacification with minimal attenuation. At least 15 cardiac cycles after high mechanical index (1.7) microbubble destruction were stored in cine-loop format.
Analysis of myocardial contrast echocardiograms was performed off-line using EchoPAC 7.0.0 (GE Healthcare). To evaluate myocardial perfusion, the left ventricle was divided according to the 16-segment model of the American Society of Echocardiography. A semiquantitative scoring system was used to assess contrast intensity after microbubble destruction: (1) normal/homogenous opacification; (2) decreased/patchy opacification; (3) minimal or absent contrast opacification. A myocardial perfusion index, expressing infarct size, was derived by adding contrast scores of all segments and dividing by the total number of segments.
Continuous variables are expressed as mean ± SD, when normally distributed, and as median and interquartile range, when not normally distributed. Categorical data are presented as absolute numbers and percentages.
Differences in continuous variables were assessed using Student’s t test or Mann-Whitney U test, if appropriate. Chi-square test or Fisher’s exact test, if appropriate, was computed to assess differences in categorical variables.
Univariate and multivariate regression analyses were performed to evaluate the relation between LVEF in patients with AMI and the characteristics of age, gender, coronary risk factors, infarct location, multivessel disease, Thrombolysis In Myocardial Infarction flow grade 3 after PCI, symptoms-to-balloon time, QRS duration, ST-segment resolution, peak troponin T, LV dyssynchrony (expressed as SDI), and infarct size (expressed as myocardial perfusion index). Only variables with a p value <0.1 at univariate analysis were entered as covariates in the multivariate model. To determine the potential incremental value of SDI over the other variables, the R 2 of the multivariate model was compared to the R 2 of the same model without SDI.
A p value <0.05 was considered statistically significant. Statistical analysis was performed using SPSS 15.0 (SPSS, Inc., Chicago, Illinois).
Results
Reliable real-time 3-dimensional echocardiographic and myocardial contrast echocardiographic data were obtained in 129 patients; consequently, 30 patients were excluded from further analysis. All control subjects had reliable real-time 3-dimensional echocardiographic data.
Clinical and echocardiographic characteristics of patients with AMI are listed in Table 1 . Sixty patients (46%) had an anterior AMI; obstructive multivessel disease (i.e., >1 vessel with luminal narrowing ≥70%) was present in 46 patients (36%).
Variable | Control Subjects | Patients With AMI | p Value |
---|---|---|---|
(n = 30) | (n = 129) | ||
Age (years) | 57 ± 11 | 58 ± 11 | 0.53 |
Men | 23 (77%) | 100 (78%) | 0.92 |
Body surface area (m 2 ) | 1.96 ± 0.58 | 1.98 ± 0.22 | 0.76 |
Diabetes mellitus | — | 14 (11%) | — |
Family history of coronary artery disease ⁎ | — | 49 (38%) | — |
Hypercholesterolemia † | — | 19 (15%) | — |
Hypertension ‡ | — | 47 (36%) | — |
Current or previous smoker | — | 76 (59%) | — |
Anterior wall acute myocardial infarction | — | 60 (46%) | — |
Infarct-related coronary artery | |||
Left anterior descending | — | 60 (46%) | — |
Left circumflex | — | 22 (18%) | — |
Right | — | 46 (36%) | — |
Multivessel coronary disease | — | 46 (36%) | — |
Thrombolysis In Myocardial Infarction flow grade 3 | 109 (85%) | — | |
Symptoms-to-balloon time (minutes) | — | 177 (134–219) | — |
QRS duration (ms) | 96 ± 13 | ||
ST-segment resolution (%) | — | 62 ± 31 | — |
Peak troponin T (μg/L) | — | 3.10 (1.52–6.89) | — |
Left ventricular end-diastolic volume (ml) | 90 ± 23 | 110 ± 30 | 0.01 |
Left ventricular end-systolic volume (ml) | 35 ± 14 | 60 ± 23 | <0.001 |
Left ventricular ejection fraction (%) | 64 ± 6 | 46 ± 9 | <0.001 |
Systolic dyssynchrony index (%) | 2.02 ± 0.70 | 5.24 ± 2.23 | <0.001 |
Myocardial perfusion index | — | 1.25 (1.09–1.50) | — |
⁎ Defined when close relatives had premature coronary artery disease (men <55 years old and women <65 years old).
† Defined as total cholesterol level ≥240 mg/dl.
‡ Defined as systolic blood pressure ≥140 mm Hg and/or diastolic blood pressure ≥90 mm Hg.
Compared to control subjects, patients with AMI had significantly lower LVEF (64 ± 6% vs 46 ± 9%, p <0.001) and significantly higher SDI (2.02 ± 0.70% vs 5.24 ± 2.23%, p <0.001). The observed impairment of LV synchronicity in patients with AMI is shown in Figure 1 .
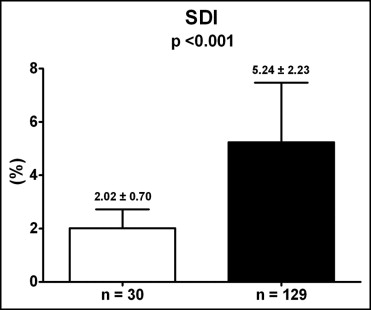
Patients with AMI were subdivided into 2 groups according to LV systolic function (LVEF ≥45%, n = 83; LVEF <45%, n = 46). Clinical and echocardiographic characteristics of these 2 groups are presented in Table 2 . Patients with LVEF <45% had more frequently multivessel disease (p = 0.032), less frequently Thrombolysis In Myocardial Infarction flow grade 3 after primary PCI (p = 0.049) and higher peak troponin T (p = 0.006) compared to patients with LVEF ≥45% ( Table 2 ).
Variable | LVEF ≥45% | LVEF <45% | p Value |
---|---|---|---|
(n = 83) | (n = 46) | ||
Age (years) | 57 ± 11 | 60 ± 11 | 0.090 |
Men | 60 (72%) | 40 (87%) | 0.056 |
Diabetes mellitus | 9 (11%) | 5 (11%) | 1.00 |
Family history of coronary artery disease | 31 (37%) | 18 (39%) | 0.84 |
Hypercholesterolemia | 12 (15%) | 7 (15%) | 0.91 |
Hypertension | 35 (42%) | 12 (26%) | 0.069 |
Current or previous smoker | 47 (57%) | 29 (63%) | 0.48 |
Anterior wall acute myocardial infarction | 34 (41%) | 26 (57%) | 0.090 |
Multivessel coronary disease | 24 (29%) | 22 (48%) | 0.032 |
Thrombolysis In Myocardial Infarction flow grade 3 | 74 (89%) | 35 (76%) | 0.049 |
Symptoms-to-balloon time (minutes) | 170 (127–215) | 184 (150–231) | 0.16 |
QRS duration (ms) | 94 ± 13 | 99 ± 13 | 0.027 |
ST-segment resolution (%) | 65 ± 31 | 56 ± 30 | 0.13 |
Peak troponin T (μg/l) | 2.69 (1.23–5.39) | 4.82 (1.85–11.02) | 0.006 |
Left ventricular end-diastolic volume (ml) | 105 ± 25 | 118 ± 38 | 0.042 |
Left ventricular end-systolic volume (ml) | 51 ± 14 | 75 ± 28 | <0.001 |
Left ventricular ejection fraction (%) | 51 ± 5 | 37 ± 6 | <0.001 |
Systolic dyssynchrony index (%) | 4.29 ± 1.44 | 6.95 ± 2.40 | <0.001 |
Myocardial perfusion index | 1.19 (1.00–1.38) | 1.56 (1.23–1.81) | <0.001 |
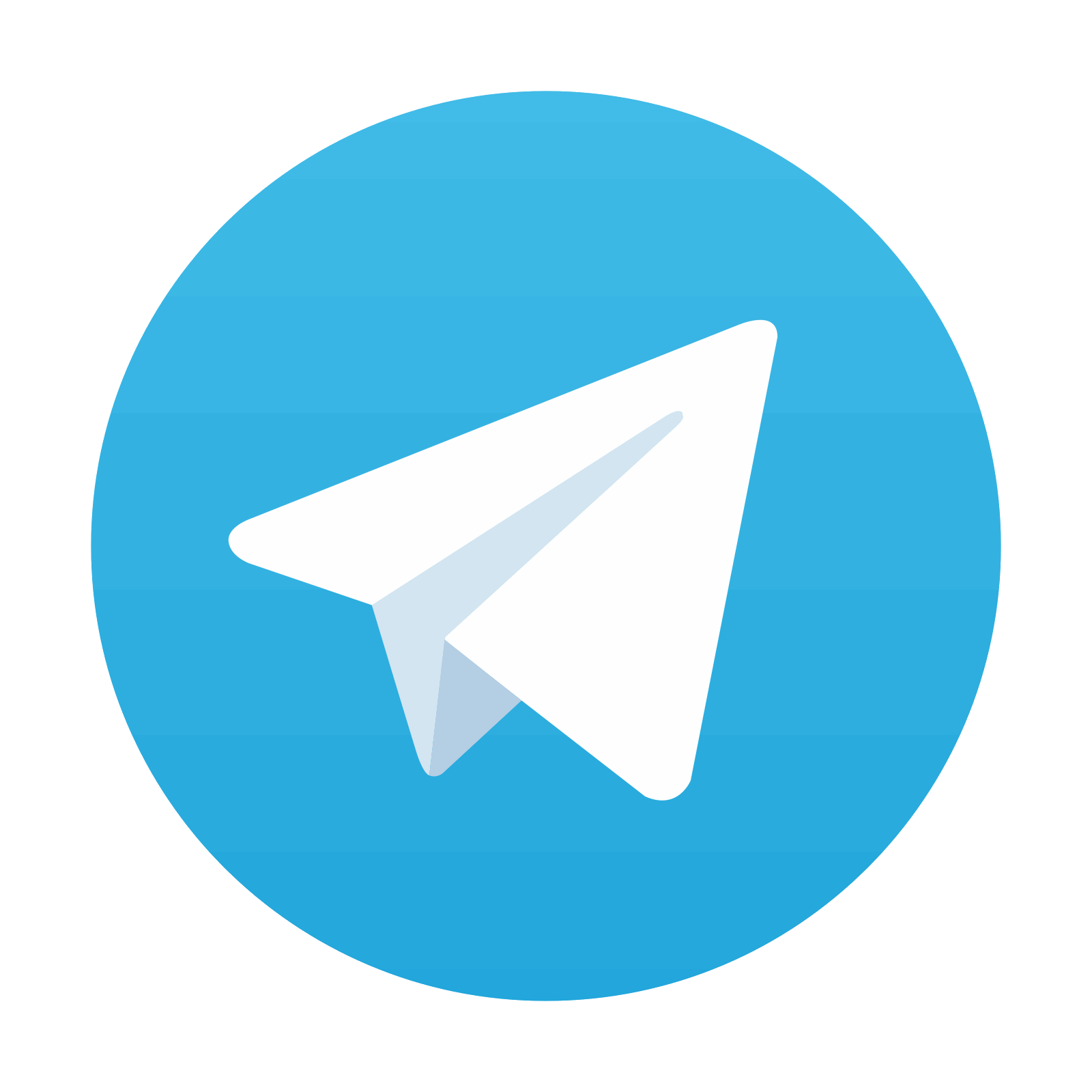
Stay updated, free articles. Join our Telegram channel

Full access? Get Clinical Tree
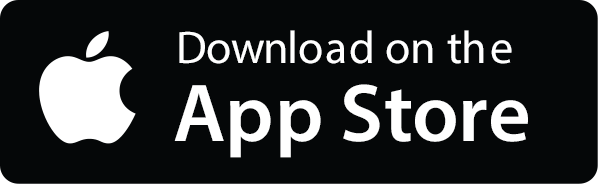
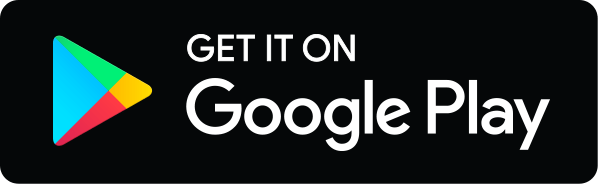
