Background
To understand better the mechanism of left ventricular (LV) remodeling related to hypertension, it is important to evaluate LV function in relation to the changes in loading conditions. The aim of this study was to investigate changes in conventional ventricular-arterial coupling indexes, LV strain, and a new index reflecting regional myocardial work assessed noninvasively at rest and during isometric exercise in a random sample including participants with normal blood pressure and those with hypertension.
Methods
A total of 148 participants (53.4% women; mean age, 52.0 years; 39.2% with hypertension) underwent simultaneous echocardiographic and arterial data acquisition at rest and during increased afterload (handgrip exercise). End-systolic pressure was determined from the carotid pulse wave. Arterial elastance (Ea) and LV elastance (Ees) were calculated as end-systolic pressure/stroke volume and end-systolic pressure/end-systolic volume. Doppler tissue imaging and two-dimensional speckle tracking were used to derive LV longitudinal strain. Regional myocardial work (ejection work density [EWD]) was the area of the pressure-strain loop during ejection.
Results
At rest, with adjustments applied, Ees (3.06 vs 3.71 mm Hg/mL, P = .0003), Ea/Ees (0.54 vs 0.47, P = .002) and EWD (670 vs 802 Pa/m 2 , P = .0001) differed significantly between participants with normal blood pressure and those with hypertension. During handgrip exercise, Ea and Ea/Ees significantly increased ( P < .0001) in both groups. Doppler tissue imaging and two-dimensional LV strain decreased in participants with hypertension ( P ≤ .008). Only in subjects with normal blood pressure EWD significantly increased (+14.7%, P = .0009).
Conclusions
Although patients with hypertension compared with those with normal blood pressure have increased LV systolic stiffness and regional myocardial work to match arterial load at rest, they might have diminished cardiac reserve to increase myocardial performance, as estimated by EWD during isometric exercise.
The interaction of the heart with the systemic vasculature, ventricular-arterial coupling (VAC), is a key determinant of cardiovascular performance. The capacity of the body to augment cardiac output, regulate systemic blood pressure (BP) and respond appropriately to elevations in heart rate and preload and afterload depends on the properties of both the heart and the vasculature into which the left ventricle ejects blood. Hypertension is associated with the stiffening of the large arteries and left ventricle. Because a high arterial pressure opposes left ventricular (LV) ejection, it might lead in the short term to a reduction of LV stroke volume, which is compensated for by shifting the LV pump function to a higher energy level (the Frank-Starling mechanism) and by activating an autoregulation mechanism (the Anrep response). Thus, the heart responds to the greater afterload with an increase in LV stiffness. In the long term, this chronically increased cardiac loading leads to LV remodeling (concentric hypertrophy), increases LV oxygen requirements, and eventually causes LV failure. Moreover, to expand stiffened arteries, the heart needs to generate greater forces. Pulse-wave velocity also increases in stiffening arteries, leading to an early return of the reflected wave, which in turn augments late systolic LV load. Further damage to the left ventricle might be caused by increasing time required for systole and shortening of diastole.
Matching the properties of the left ventricle to those of the arteries might preserve cardiac mechanic efficiency at rest, but not necessarily during LV loading. Measurement of VAC generally requires invasive registration of LV pressures and LV volumes, recorded over a wide range of LV loads. Effective arterial elastance (Ea), commonly known as the ratio of LV end-systolic pressure (ESP) to stroke volume (SV), reflects the net arterial load imposed on the left ventricle. LV end-systolic elastance (Ees) provides an estimate of LV performance and is calculated by measuring the slope of the relations between ESP and end-systolic volume (ESV) registered over a range of LV loads. The Ea/Ees ratio is commonly used as an index of the interaction between the left ventricle and the arterial system. Some investigators have proven the feasibility of a noninvasive assessment of VAC in patients with hypertension. For instance, Osranek et al. measured LV volumes by echocardiography and estimated central aortic pressure from a tonometrically recorded pulse-wave signal at the radial artery at rest and during handgrip exercise.
Additional information about LV performance can also be derived from an assessment of myocardial deformation (strain). On the basis of Doppler tissue imaging (DTI) and two-dimensional (2D) speckle tracking, regional myocardial strain curves can be calculated. Moreover, strain in combination with simultaneously measured LV pressure can be used to estimate regional myocardial work. Thus, using the relation between regional LV deformation (strain) and loading, a measure of intrinsic myocardial performance can be obtained.
To understand better the mechanism of LV remodeling related to hypertension, it is important to evaluate LV function in relation to the changes in the loading conditions in subjects with normal BP and those with hypertension. Few studies have examined noninvasively the changes in VAC components during exercise in patients with hypertension. To our knowledge, no population study thus far has described changes in LV myocardial deformation (strain) and/or regional myocardial work derived from simultaneously obtained estimates of LV ESP and strain under different loading conditions. In the present study, we investigated changes in conventional VAC indexes, LV longitudinal strain, and a new index reflecting regional myocardial work assessed noninvasively at rest and during isometric exercise in a random sample including participants with normal BP and those with hypertension.
Methods
Study Participants
Study participants were from the Flemish Study on Environment, Genes and Health Outcomes (FLEMENGHO), consisting of a random population sample stratified by sex and age from a geographically defined area in northern Belgium. Seven municipalities gave listings of all inhabitants sorted by address. Households, defined as subjects living at the same address, were the sampling unit. We numbered households consecutively and generated a random-number list using SAS (SAS Institute Inc., Cary, NC). Households with numbers matching the list were invited. The ethics committee of the University of Leuven approved the study, and participants provided informed consent. FLEMENGHO participants were repeatedly visited at home and examined at a local examination center. At each contact, standardized and validated questionnaires were administered to collect detailed information about each participant’s personal and familial medical history, use of medications, and lifestyle. In 2009 and 2010, we reinvited 215 former FLEMENGHO participants for follow-up examinations at our field center, including echocardiography and the isometric exercise test (participation rate, 81.3%).
We excluded 26 subjects from statistical analysis because of myocardial infarction or coronary revascularization ( n = 6), moderate to severe valvular abnormalities ( n = 9), atrial fibrillation ( n = 5), or symptomatic heart failure ( n = 6). We excluded a further 41 subject, because DTI studies or 2D echocardiograms ( n = 15) or carotid artery pressure waves ( n = 21) were of insufficient quality, as well as participants who did not comply with the study protocol ( n = 5). Thus, the number of participants statistically analyzed totaled 148.
Echocardiography
Participants refrained from smoking, heavy exercise, and drinking alcohol or caffeine-containing beverages for ≥3 hours before echocardiography.
Data Acquisition
One experienced physician (T.K.) performed the ultrasound examinations according to a standardized protocol as published elsewhere, using a Vivid 7 Pro (GE Vingmed Ultrasound AS, Horten, Norway) interfaced with a 2.5-MHz phased-array probe. With the subject in the partial left decubitus position and breathing normally, the observer obtained images from the parasternal long and short axes and from the apical four-chamber and two-chamber and long-axis views. All recordings included at least five cardiac cycles and were digitally stored for offline analysis.
Using DTI, the observer recorded low-velocity, high-intensity myocardial signals at a high frame rate (>190 frames/sec), while adjusting the imaging angle to ensure parallel alignment of the ultrasound beam with the myocardial segment of interest. The Nyquist limit was set as low as possible avoiding aliasing.
Offline Analysis
The same observer (T.K.) analyzed recorded images, averaging three heart cycles for statistical analysis, using a workstation running EchoPAC version 4.0.4 (GE Vingmed Ultrasound AS). LV ESV and end-diastolic volume were measured offline from the apical four-chamber and two-chamber views, using the standard biplane Simpson’s method.
On the basis of color Doppler myocardial motion data, one-dimensional longitudinal regional strain rate and strain curves were calculated by comparing local myocardial velocity profiles, using dedicated software. The SPEQLE package (version 4.6.2) allows M-mode tracking of the myocardium to ensure that the sample volume is maintained in the same anatomic position within myocardial image throughout the cardiac cycle. We positioned the sampling volume in the septal, lateral, inferior, and posterior walls at the level of the posterior chordae tendineae. To compute end-systolic strain, hereafter referred to as strain, we averaged three consecutive measurements and used their absolute values. We calculated the longitudinal strain rate of each interrogated LV wall by measuring the spatial velocity gradient over a computation area of 15 mm. Strain curves were obtained by integrating the mean strain rate profile over time. The beginning and ending of the ejection phase were determined from the simultaneously recorded electrocardiogram and the continuous-wave Doppler velocity trace at the level of the aortic valve. We used lateral averaging of 5 beams/pixel.
For measurement of 2D strain, the endocardial borders were manually traced at the end-systolic frame of the 2D images from the three long-axis views. The 2D strain software (Q-analysis; GE Vingmed Ultrasound AS) automatically tracks myocardial speckle motion, creating basal, mid, and apical regions of interest.
Carotid Pressure Waveform Analysis
During the echocardiographic examination, an experienced technician registered the carotid arterial waveform signal at the right side by applanation tonometry (TY-306; Fukuda Denshi, Tokyo, Japan). Brachial systolic and diastolic BP measured during the echocardiographic examination at rest and during the handgrip test with a validated Omron 705IT device (Omron Corporation, Tokyo, Japan) were used to calibrate the carotid pressure wave. ESP was determined from the carotid pressure wave at the incisura reflecting aortic valve closure.
Study Protocol
All participants underwent simultaneous echocardiographic and arterial data acquisition at rest and during an increase of LV afterload induced by isometric handgrip exercise. Maximal grip-force capacity was determined before the echocardiographic examination. Each participant performed three maximal voluntary left forearm contractions with a Stoelting handgrip dynamometer (Stoelting, Wood Dale, IL). The force of these three contractions was averaged. During the isometric exercise, each subject squeezed the dynamometer with the left arm at a submaximal target of 40% until fatigue. The last set of continuously recorded 2D and DTI echocardiographic data was used to derive exercise values.
Data Analysis
Ea and Ees were calculated as ESP/SV and ESP/ESV, respectively. The Ea/Ees ratio represents the VAC index. DTI strain and the carotid pressure curves were exported to SPEQLE software for further analysis. From the DTI strain curve and the simultaneously recorded pressure waveforms at the carotid artery, the regional myocardial work density was calculated as a quantitative measure of regional LV performance as previously described. Hereto, the instantaneous pressures were plotted against the instantaneous strain values ( Figure 1 ) with indications of different mechanical phases of the cardiac cycle (i.e., onset of the cycle, isovolumic contraction, ejection, isovolumic relaxation, and early and late filling phases) as derived from pulse-wave Doppler tracings through the aortic and mitral valves. Because pressure recordings obtained in the carotid artery are only representative of LV pressures while the aortic valve is opened, ejection work density (EWD) was calculated as the area of the pressure-strain loop during the ejection phase because this area represents the cumulative work done by the muscle to instantaneously shorten a given amount (i.e., change in strain) at a given instantaneous resistance (i.e., pressure). Postprocessing was achieved within a MATLAB environment (The MathWorks Inc., Natick, MA).

Reproducibility
To determine the interobserver variability of LV DTI and 2D strain and EWD, two observers analyzed twice the LV deformation curves. Absolute and relative differences between two observers were calculated according to Bland and Altman’s method as ( x 1 − x 2 ) versus averaged and (100 × [ x 1 − x 2 ]/averaged) versus averaged, respectively. For DTI longitudinal strain, the absolute and relative differences were −0.29% (95% limits of agreement [LA], −3.73% to 3.14%) and −1.38% (95% LA, −16.8% to 14.1%), respectively. The absolute and relative differences for 2D strain across 37 pairwise readings were −0.30% (95% LA, −1.72% to 2.32%) and −1.42% (95% LA, −11.7% to 8.87%), respectively. The absolute and relative differences for EWD measurement across 34 pairwise readings were −14.5 Pa/m 2 (95% LA, −82.3 to 53.4 Pa/m 2 ) and −2.01% (95% LA, −11.6% to 7.54%), respectively. We also calculated intraclass correlation coefficients with 95% confidence intervals (CIs) while taking into account the bias across pairwise readings. The interobserver intraclass correlation coefficients for longitudinal DTI strain, 2D strain, and EWD were 0.87 (95% CI, 0.82 to 0.91), 0.89 (95% CI, 0.80 to 0.94), and 0.98 (95% CI, 0.97 to 0.99), respectively.
Other Measurements
At the examination center, trained study nurses administered a questionnaire to collect detailed information on each subject’s medical history, smoking and drinking habits, and intake of medications. The conventional BP was the average of five consecutive auscultatory readings obtained at the examination center with the subject in the seated position. Hypertension was defined as systolic BP ≥140 mm Hg or diastolic BP ≥90 mm Hg or the use of antihypertensive drugs. Body mass index was calculated as weight in kilograms divided by the square of height in meters.
Statistical Methods
For database management and statistical analysis, we used SAS version 9.1. The central tendency and the spread of the data are reported as mean ± SD. We compared means and proportions using t tests and χ 2 tests, respectively. Significance was set at P < .05 in two-sided tests. We applied a generalization of the standard linear model, as implemented in the PROC MIXED procedure of SAS, to investigate the associations between changes in LV volumes, VAC components, and LV strain and changes in ESP during handgrip exercise, while accounting for covariates such as sex, baseline values at rest, and age. We expressed multivariate-adjusted effect sizes for 10 mm Hg changes in LV ESP during handgrip exercise.
Results
Characteristics of Participants
The 148 participants included 79 women (53.4%) and 58 patients with hypertension (39.2%), of whom 34 (23.0%) were on one or more antihypertensive drugs (β-blockers, n = 15; diuretics, n = 11; calcium channel blockers, n = 11; angiotensin-converting enzyme inhibitors or angiotensin receptor blockers, n = 11). Table 1 shows the clinical characteristics of the study participants by hypertension status. Patients with hypertension were older and had higher body mass indexes compared with those with normal BP ( Table 1 ). Mean handgrip force was similar in both groups, averaging 13.1 ± 4.3 kg over 286 ± 57 sec. As expected, LV mass index and relative wall thickness ( Table 1 ) were significantly greater in patients with hypertension than those with normal BP. Participants with hypertension also had lower transmitral E/A ratios, mitral annular e′ and e′/a′ ratios, but higher E/e′ ratios ( P < .0001). With adjustments applied for sex, age, and body mass index, only E/e′ ratio remained significantly different ( P = .0075) according to hypertensive status, indicative of worse diastolic function (higher LV diastolic filling pressures) in patients with hypertension.
Characteristic | Normal BP ( n = 90) | Hypertension ( n = 58) | P |
---|---|---|---|
Anthropometrics | |||
Age (y) | 44.6 ± 15.2 | 57.7 ± 10.6 | <.0001 |
Women | 50 (55.6%) | 29 (50.0%) | .17 |
Height (cm) | 171.6 ± 9.7 | 169.1 ± 9.7 | .13 |
Weight (kg) | 76.4 ± 15.4 | 79.1 ± 12.7 | .27 |
Body mass index (kg/m 2 ) | 25.8 ± 3.7 | 27.6 ± 3.0 | .003 |
Body surface area (m 2 ) | 1.89 ± 0.22 | 1.90 ± 0.19 | .77 |
Questionnaire data | |||
Treated for hypertension | — | 34 (58.6%) | — |
Handgrip test performance | |||
Handgrip force (kg) | 13.1 ± 4.3 | 13.0 ± 4.2 | .93 |
Test duration (sec) | 288 ± 63 | 281 ± 47 | .45 |
Echocardiographic data | |||
LV mass index (g/m 2 ) | 88.0 ± 19.2 | 99.5 ± 21.4 | .0006 |
Relative wall thickness | 0.36 ± 0.047 | 0.40 ± 0.052 | <.0001 |
Transmitral E/A ratio | 1.45 ± 0.54 | 1.05 ± 0.27 | <.0001 |
Mitral annular e′ (cm/sec) | 12.2 ± 3.20 | 9.36 ± 2.26 | <.0001 |
DTI e′/a′ ratio | 1.57 ± 0.76 | 0.96 ± 0.35 | <.0001 |
E/e′ ratio | 6.38 ± 1.36 | 7.83 ± 1.86 | <.0001 |
VAC Components and LV Longitudinal Strain at Rest in Participants with Hypertension and Those with Normal BP
Table 2 shows the hemodynamic and VAC characteristics and LV longitudinal strain by hypertension status at rest and during handgrip exercise. As expected, at rest, systolic and diastolic BP and ESP were significantly higher in patients with hypertension than in subjects with normal BP ( P < .0001). ESV ( P = .04) but not SV ( P = .10) was lower in patients with hypertension ( Table 2 ). The Ea/Ees ratio was 16.4% lower ( P < .0001) in patients with hypertension. Examining the components of this ratio, both Ea ( P = .02) and Ees ( P < .0001) were higher in patients with hypertension compared with subjects with normal BP. With adjustments applied for sex, age, and body mass index, only Ees (3.06 vs 3.71 mm Hg/mL, P = .0003) and Ea/Ees ratio (0.54 vs 0.47, P = .002) differed significantly according to hypertension status.
Variable | Normal BP ( n = 90) | Hypertension ( n = 58) | P (normal BP vs hypertension) | |||||||
---|---|---|---|---|---|---|---|---|---|---|
Rest | Handgrip exercise | Δ | P | Rest | Handgrip exercise | Δ | P | Rest | Handgrip exercise | |
Hemodynamic | ||||||||||
Heart rate (beats/min) | 58.0 ± 8.5 | 65.3 ± 10.0 | 7.3 ± 5.3 | <.0001 | 55.9 ± 7.3 | 62.6 ± 11.1 | 6.7 ± 6.6 | <.0001 | .13 | .13 |
Brachial systolic BP (mm Hg) | 109.9 ± 12.3 | 127.7 ± 15.4 | 17.8 ± 13.3 | <.0001 | 127.5 ± 17.2 | 144.8 ± 17.5 | 17.3 ± 15.0 | <.0001 | <.0001 | <.0001 |
Brachial diastolic BP (mm Hg) | 63.6 ± 8.7 | 76.0 ± 10.8 | 12.4 ± 10.4 | <.0001 | 72.3 ± 12.2 | 81.8 ± 11.7 | 9.6 ± 11.9 | <.0001 | <.0001 | .002 |
End-systolic BP (mm Hg) | 94.7 ± 12.1 | 108.4 ± 14.6 | 13.7 ± 11.7 | <.0001 | 112.0 ± 15.5 | 122.4 ± 13.5 | 10.4 ± 13.5 | <.0001 | <.0001 | <.0001 |
VAC components | ||||||||||
End-diastolic volume (mL) | 99.3 ± 22.6 | 102.5 ± 24.0 | 3.2 ± 7.4 | .0001 | 100.8 ± 21.8 | 101.6 ± 21.8 | 0.83 ± 8.1 | .45 | .88 | .83 |
ESV (mL) | 34.9 ± 10.0 | 38.7 ± 11.0 | 3.7 ± 4.7 | <.0001 | 31.8 ± 10.1 | 35.1 ± 11.3 | 3.3 ± 4.5 | <.0001 | .04 | .07 |
SV (mL) | 64.4 ± 14.5 | 63.9 ± 15.0 | −0.48 ± 7.0 | .51 | 69.0 ± 14.2 | 66.4 ± 13.0 | −2.6 ± 8.7 | .03 | .10 | .31 |
Ea (mm Hg/mL) | 1.55 ± 0.40 | 1.80 ± 0.49 | 0.25 ± 0.29 | <.0001 | 1.69 ± 0.40 | 1.90 ± 0.38 | 0.21 ± 0.26 | <.0001 | .02 | .16 |
Ees (mm Hg/mL) | 2.99 ± 1.11 | 3.06 ± 1.06 | 0.07 ± 0.44 | .14 | 3.87 ± 1.33 | 3.83 ± 1.24 | −0.04 ± 0.73 | .70 | <.0001 | .0002 |
Ea/Ees ratio | 0.55 ± 0.12 | 0.61 ± 0.13 | 0.06 ± 0.10 | <.0001 | 0.47 ± 0.12 | 0.53 ± 0.13 | 0.06 ± 0.10 | <.0001 | <.0001 | .0004 |
LV strain | ||||||||||
TDI strain | ||||||||||
Basal-mid strain (%) | 20.8 ± 2.54 | 21.1 ± 2.95 | 0.31 ± 2.64 | .27 | 21.2 ± 2.67 | 19.6 ± 3.02 | −1.6 ± 3.0 | .0002 | .39 | .004 |
EWD (Pa/m 2 ) | 652 ± 174 | 748 ± 203 | 96 ± 190 | <.0001 | 859 ± 231 | 839 ± 246 | −20 ± 217 | .48 | <.0001 | .02 |
2D speckle-tracking strain | ||||||||||
Basal-mid strain (%) | 20.0 ± 2.35 | 19.6 ± 2.63 | −0.39 ± 2.0 | .07 | 20.7 ± 1.98 | 19.8 ± 2.40 | −0.87 ± 2.3 | .009 | .07 | .59 |
Apical strain (%) | 23.2 ± 2.61 | 22.3 ± 3.01 | −0.94 ± 3.2 | .007 | 24.3 ± 3.41 | 23.0 ± 3.59 | −1.36 ± 4.0 | .01 | .03 | .22 |
Averaged strain (%) | 21.2 ± 1.77 | 20.6 ± 2.39 | −0.61 ± 2.1 | .007 | 21.7 ± 1.79 | 21.0 ± 2.02 | −0.67 ± 1.9 | .01 | .13 | .30 |
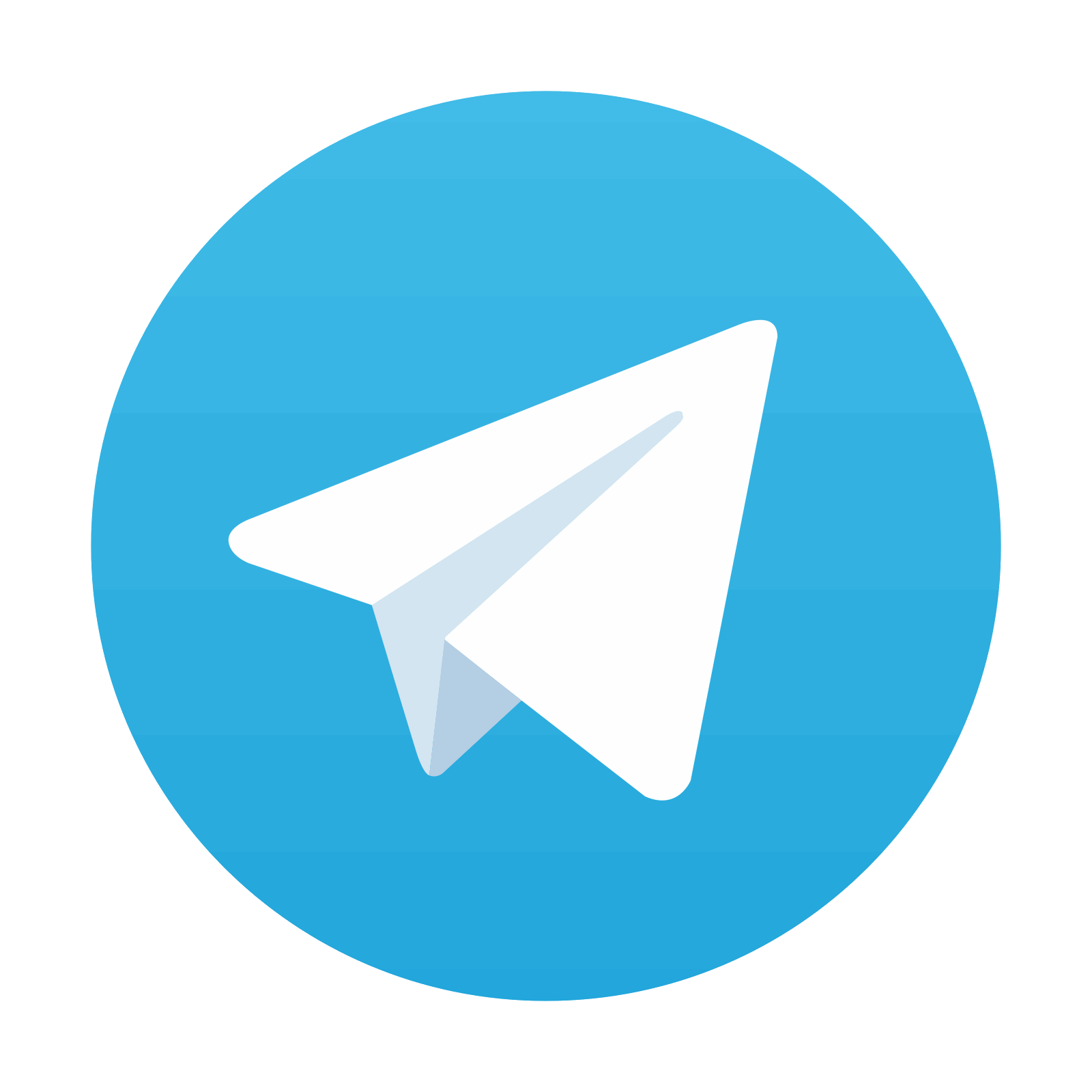
Stay updated, free articles. Join our Telegram channel

Full access? Get Clinical Tree
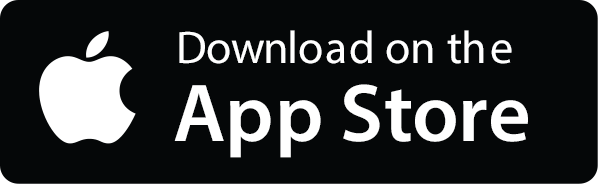
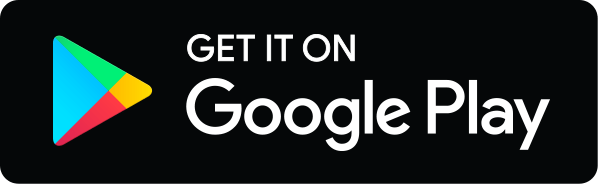
