Imaging Techniques in Nuclear Cardiology
Manuel D. Cerqueira
Myocardial perfusion imaging (MPI) using radionuclide techniques with electrocardiographically (ECG)-gated single photon emission computed tomography (SPECT) is the most commonly performed diagnostic test for assessing the presence of critical coronary artery stenosis and determining prognosis. It provides diagnostic and prognostic clinical benefit in the initial evaluation of patients with suspected, but unproved coronary artery disease (CAD) and in those patients where a diagnosis of CAD has been established and information on prognosis or risk is required (1). Although radionuclide techniques can also be used to assess function, metabolism, innervation, and infarction, these techniques are seldom used in clinical practice. Positron emission tomography (PET) has superior imaging properties and capabilities over SPECT, but the cost and limited availability of cyclotron or generator produced radioactive radiotracers has limited widespread utilization. Currently there are more than 8 million cardiac SPECT but fewer than 50,000 cardiac PET procedures preformed annually in the United States.
Since the last publication of this textbook, new American College of Cardiology (ACC)/American Heart Association (AHA)/American Society of Nuclear Cardiology (ASNC) Guidelines for the Clinical Use of Cardiac Radionuclide Imaging have been published and are included in this section (1). In addition, a new process for assessing the appropriateness of radionuclide imaging was undertaken and published as the ACC/ ASNC Appropriateness Criteria for Single-Photon Emission computed Tomography Myocardial Perfusion Imaging (SPECT MPI) (2). This chapter reviews the basic physiologic basis for SPECT MPI, radionuclides, protocols, exercise and pharmacologic stress, image interpretation, and the application of these techniques based on the guidelines in important clinical settings.
Basis of Myocardial Perfusion Imaging
During stress, blood flow increases through normal coronary arteries. In vessels with significant luminal stenosis, variously defined as anywhere from 50% to 80% luminal cross-sectional narrowing, or abnormal functional flow reserve, there is no increase, the increase is less or occasionally there may even be a decrease in coronary artery blood flow. This diminished coronary flow reserve is not an all-or-none phenomenon; rather, flow reserve decreases gradually as the severity of the coronary stenosis progresses. The most severe stenosis, greater than 85%, typically have no flow reserve. Given this framework, the use of radionuclides requires a measurement of flow in the resting state and after some maneuver, usually exercise or pharmacologic stress, that increases coronary blood flow and results in flow heterogeneity between normal vessels and those with anatomic narrowing or physiologic impairment.
Radiotracers and Protocols
The three commonly used radiotracers are thallium-201 (Tl-201) and the 2 technetium-99m (Tc-99m) labeled compounds, sestamibi, and tetrofosmin. The properties of these tracers are listed in Table 53.1.
Radiotracers
Thallium-201
Tl-201 was the first radiotracer used for clinical MPI. It is a K+ analog that is transported across cell membranes by the Na/K ATPase system (3). It has an initial myocardial uptake that is proportional to blood flow over a wide physiologic range and depends on the presence of viable myocardial cells. Following the very rapid and high initial uptake, Tl-201 reequilibrates with the lower concentration in the blood in a time-dependent fashion. This is called washout or redistribution and, like the initial uptake of Tl-201, is directly proportional to blood flow to the area and the presence of functioning myocytes. In the typical imaging sequence using Tl-201 (Figure 53.1), the patient has a stress study with dynamic exercise or pharmacologic
stress. Within 10 to 15 minutes of completion of stress the patient is imaged and this is followed 2.5 to 4 hours later by a redistribution set of images. Areas of myocardium supplied by normal coronary arteries have a high and uniform uptake on both the stress and redistribution images. Areas of infarction have very low or absent uptake on the initial stress images; there are a diminished number of myocytes and there is no change on the redistribution images. Following stress, there is an increase in blood flow and those areas of myocardium beyond a flow-limiting critical anatomic or physiologic coronary stenosis have low uptake of Tl-201 relative to the areas of myocardium with normal coronary blood supply. Areas of ischemia and infarction look identical on the poststress images. However, with ischemia the redistribution images show improvement in the ischemic areas and this allows the differentiation to be made between infarct and ischemic myocardium.
stress. Within 10 to 15 minutes of completion of stress the patient is imaged and this is followed 2.5 to 4 hours later by a redistribution set of images. Areas of myocardium supplied by normal coronary arteries have a high and uniform uptake on both the stress and redistribution images. Areas of infarction have very low or absent uptake on the initial stress images; there are a diminished number of myocytes and there is no change on the redistribution images. Following stress, there is an increase in blood flow and those areas of myocardium beyond a flow-limiting critical anatomic or physiologic coronary stenosis have low uptake of Tl-201 relative to the areas of myocardium with normal coronary blood supply. Areas of ischemia and infarction look identical on the poststress images. However, with ischemia the redistribution images show improvement in the ischemic areas and this allows the differentiation to be made between infarct and ischemic myocardium.
TABLE 53.1 Comparison of Thallium-201, Sestamibi, and Tetrofosmin for MPI | ||||||||||||||||||||||||||||||||||||||||||||||||||||||||||||||||||||||||||||
---|---|---|---|---|---|---|---|---|---|---|---|---|---|---|---|---|---|---|---|---|---|---|---|---|---|---|---|---|---|---|---|---|---|---|---|---|---|---|---|---|---|---|---|---|---|---|---|---|---|---|---|---|---|---|---|---|---|---|---|---|---|---|---|---|---|---|---|---|---|---|---|---|---|---|---|---|
|
One of the major advantages of using Tl-201 is that it is also an excellent marker of myocardial viability (4). The high and nearly linear initial myocardial uptake following stress makes Tl-201 a marker of myocardial blood flow. The uptake and redistribution over time is a marker of the K+ blood pool and of viable myocardium. In areas where blood flow is severely reduced following stress, there may be very low uptake of Tl-201 initially despite the presence of living myocytes. Even the 3- to 4-hour redistribution images may still be abnormal. However, over time, if there are viable myocytes with an intact Na/K ATPase system, Tl-201 is eventually taken up and these areas are clearly identified as hibernating myocardium and not infarction.
Assessment of viability is especially important in patients with congestive heart failure, known cardiomyopathies, or recent myocardial infarction. In patients where myocardial viability assessment is of critical clinical importance and PET is not available, several approaches using Tl-201 are shown in Figure 53.1 that can be used to further improve on the accuracy of the regular stress/redistribution sequence. These approaches include a rest injection followed 3 to 4 hours later by redistribution images; reinjection of Tl-201 before the redistribution imaging in a stress redistribution sequence; or delayed imaging at 18 to 24 hours in the typical stress/redistribution sequence.
Tc-99m Radiotracers
Tc-99m tracers were developed to overcome limitations of Tl-201. There are two Tc-99m tracers used clinically: Tc-99m sestamibi (Cardiolite) and Tc-99m tetrofosmin (Myoview). The monoenergetic140 keV higher energy of Tc-99m versus the 69-83 keV of Tl-201 results in improved resolution with less overall attenuation and scatter during imaging. Tc-99m has a half-life of 6 hours in comparison to the 72 hours of Tl-201. The shorter half-life allows administration of a higher Tc-99m dose resulting in high-count images. As an example, 4 mCi of Tl-201 are administered as compared to 30 mCi of the Tc-99m
radiotracers. The higher counts obtained with a Tc-99m radiopharmaceutical and the lack of redistribution allows ECG-triggered gated acquisition, ECG-gated SPECT, for assessment of function. The minimal redistribution of Tc-99m tracers following injection allows greater flexibility as to when acquisition can be performed; as soon as 10 minutes or as long as 4 hours following stress. Patients can be injected in remote locations of the hospital, such as in the emergency department for patients presenting with chest pain and a nondiagnostic ECG. They can be safely and conveniently transported for imaging when they are stable. The improved imaging characteristics of Tc-99m tracers are especially beneficial in women and obese patients where soft tissue attenuation has been a traditional problem for Tl-201. This frequently resulted in false-positive interpretations and a low specificity.
radiotracers. The higher counts obtained with a Tc-99m radiopharmaceutical and the lack of redistribution allows ECG-triggered gated acquisition, ECG-gated SPECT, for assessment of function. The minimal redistribution of Tc-99m tracers following injection allows greater flexibility as to when acquisition can be performed; as soon as 10 minutes or as long as 4 hours following stress. Patients can be injected in remote locations of the hospital, such as in the emergency department for patients presenting with chest pain and a nondiagnostic ECG. They can be safely and conveniently transported for imaging when they are stable. The improved imaging characteristics of Tc-99m tracers are especially beneficial in women and obese patients where soft tissue attenuation has been a traditional problem for Tl-201. This frequently resulted in false-positive interpretations and a low specificity.
Tc-99m Sestamibi and Tetrofosmin
Both Tc-99m sestamibi and tetrofosmin are monovalent hydrophilic cations, which makes them very lipophilic and facilitates entry into cells. Uptake depends on blood flow, plasma- and mitochondrial-derived membrane electrochemical gradients, cellular pH, and intact energy pathways (3). Chemical or structural conversion of the molecule is not required, and inhibitors of Na+, K+, and Ca2+ transport do not prevent uptake or retention in myocytes. Once inside the myocyte, they remain trapped with the greatest concentration in the mitochondria and there is minimal washout. Following intravenous injection they are cleared from blood by the liver, concentrated in the gallbladder and excreted through the common bile duct into the gastrointestinal (GI) tract. The initial high liver uptake does not allow the inferior wall to be seen clearly early postinjection and requires waiting 30 to 60 minutes following resting or pharmacologic stress injection to obtain adequate visualization. Clearance postexercise is rapid and image acquisition can be started as soon as 10 to 20 minutes following injection.
Tc-99m Radiotracer Imaging Protocol Options
The clearance characteristics of Tc-99m sestamibi and tetrofosmin are sufficiently similar that recommendations for imaging are identical with some variation between injection time and when to image owing to differences in liver clearance (5). The following wait time intervals between injection and starting imaging are recommended: 10 to 20 minutes following exercise stress and 30 to 60 minutes after rest or pharmacologic stress.
There are several protocol options for Tc-99m radiotracer imaging: a 2-day protocol, a 1-day split-dose protocol using either a stress/rest or rest/stress sequence, and a dual isotope approach using Tl-201 at rest and a Tc-99m radiotracer with exercise or pharmacologic stress. Diagnostic accuracy results reported in the literature did not report significant differences between the various protocol options or between these agents and Tl-201 (1).
Two-Day Protocol
The sequence for a 2-day imaging protocol (Figure 53.2) allows the administration of the maximal dose of Tc-99m—20 to 30 mCi—for both the rest and stress studies and results in high counts and better image quality. It is mandatory in obese patients, and allows a true rest ejection fraction (EF) to be calculated as well as poststress measurement, which provides additional value. Although this is the best way to perform Tc-99m MPI, it is inconvenient for most patients to make two visits and there is a delay in providing referring physicians with the needed clinical information.
The advantage of doing the stress study on the first day is that if it is normal, the resting study is not needed. This approach minimizes delay in getting results to the referring physician, patient inconvenience is minimal, and total study cost and radiation exposure are lower. Unfortunately, in obese patients with soft tissue attenuation and low counts, there are usually enough areas in question owing to attenuation that the resting study is usually required the following day. Gating and attenuation correction improve specificity and decrease the number of patients needing to return for a rest study (6,7).
One-Day Studies
For logistical reasons, stress and rest studies are usually performed using a 1-day protocol as shown in Figures 53.3, 53.4, 53.5, and 53.6 for exercise and pharmacologic stress. This requires administration of a low dose, one third of the total dose or 8 to 12 mCi, for the first study and a larger dose, two thirds of the total dose or 20 to 30 mCi, for the second study and waiting as long as possible between studies, usually 1.5 to 2.5 hours, to allow for physical decay of Tc-99m. Using this approach, when the second set of images area are acquired, there is relatively little contamination from the first injection. The timing and sequence for a 1-day stress/rest Tc-99m radiotracer study is shown in Figure 53.3. In this sequence, the lower Tc-99m radiotracer dose is administered when myocardial blood flow is the highest, during stress, and background activity in the subdiaphragmatic area is low owing to a decrease in mesenteric blood flow. If the stress study is normal, the rest study is not needed. If the stress is abnormal, the rest study, using a higher dose, can be started almost immediately.
![]() FIGURE 53.3. Time course for a 1-day exercise stress/rest protocol for use with Tc-99m radiotracers. When the initial stress study is normal, the resting study is optional. |
![]() FIGURE 53.5. Time course for a separate acquisition dual isotope study using Tl-201 at rest and Tc-99m radiotracer tracer for the stress study. |
The 1-day rest/stress sequence (Figure 53.4) is the most frequently performed protocol. The disadvantage of this sequence is that the low Tc-99m tracer dose is given during the rest study when blood flow is lowest and there is less myocardial uptake. The higher stress dose, administered when blood flow is maximal, gives higher counts and better quality images. The gated poststress image in patients with extensive ischemia may give a lower EF, owing to residual myocardial stunning, then a true resting EF (8). In patients with ischemia, a lower poststress EF is a better predictor of higher cardiac death and infarction rate then the size and severity of the defect alone (9,10).
Separate Acquisition Dual Isotope Thallium-201/Tc99 Radiotracer Protocol
The timing and sequence for a Tl-201/Tc-99m radiotracer dual isotope separate acquisition protocol is displayed in Figure 53.7. For the resting Tl-201 study, the patient should be fasting and a dose of 3 to 4 mCi of Tl-201 injected. Imaging should be delayed for 10 to 15 minutes. After acquiring these images, the patient is stressed and injected with 20 to 30 mCi of a Tc-99m tracer. The separate acquisition approach takes advantage of the two distinct and separate energy levels of Tl-201 and Tc-99m. During the second acquisition, the γ-camera selectively acquires data centering on the higher energy Tc-99m window. Although, there is residual Tl-201 present from the resting study, the lower energy Tl-201 photons are excluded by the higher Tc-99m window. The small amount of higher energy Tl-201 decay that is incorrectly registered in the Tc-99m window has a negligible effect on the images.
In situations where critical management decisions need to be made regarding myocardial hibernation, a rest–4 hour redistribution Tl-201 study should be performed prior to performing the stress study (11). Patients can also be injected with Tl-201 the evening before imaging to give an approximate 12-hour Tl-201 acquisition the following morning. This is an excellent method for the identification of myocardial viability. When the stress study has already been performed and questions of viability remain, delayed Tl-201 imaging cannot be performed on the same day because of the downscatter of the Tc-99m into the Tl-201 energy window. Patients can return the next day for imaging when decay of the Tc-99m has occurred and the distribution of Tl-201 can be imaged to assess viability.
Pharmacologic Stress Agents and Protocols
All MPI studies should be done using dynamic exercise stress whenever possible (12). Dynamic exercise stress provides important information for diagnosis and management that is not available from pharmacologic stress agents. Pharmacologic stress is reserved for patients who are unable to exercise or are unable to achieve maximal exercise as determined by the age-adjusted heart rate or by reaching an ischemic endpoint on the bases of symptoms or ECG changes. Sensitivity of MPI decreases in patients who achieve less than 85% of the predicted heart rate (13). If the myocardial perfusion images are normal, it cannot be certain if they are truly normal or owing to an inadequate level of stress achieved during submaximal exercise. Alternatively, if there is ischemia, it is never certain if the full extent of ischemia was detected or only the most severe areas of coronary artery obstruction. Either way, uncertainty remains and a second stress test using a pharmacologic agent must be performed.
The pharmacologic stress agents and their characteristics are listed in Table 53.2. They can be classified into two categories: adenosine and dipyridamole, which are vasodilators, and dobutamine, which is an adrenergic stimulant. The vasodilators produce maximal coronary hyperemia creating flow heterogeneity by causing a greater increase in blood flow in normal coronary arteries then in arteries with flow-limiting stenosis. Dobutamine increases blood flow, heart rate, and blood pressure and may create true ischemia.
![]() FIGURE 53.7. Protocol for adenosine pharmacologic stress testing using a 1-day Tc-99m imaging sequence. |
![]() FIGURE 53.8. Protocol for dipyridamole pharmacologic stress testing using a 1-day Tc-99m imaging sequence. |
Vasodilators
Mechanism of Action
As shown in Figure 53.8, adenosine is produced inside many cell types where it has no effect on blood flow. It is actively transported into the extracellular space, where it is capable of binding and stimulating a series of adenosine (A) receptors. Inactivation of extracellular adenosine is less than 10 seconds via reuptake by the same transport system and active metabolism. Dipyridamole blocks this reuptake mechanism and increases extracellular concentration of endogenously produced adenosine. Adenosine nonselectively stimulates all A receptors. The A2a receptors, present on vascular smooth muscle and endothelial cells, enhances arterial wall smooth muscle dilation, and increases coronary blood flow. Adenosine also stimulates the A1, A2b, and A3 receptors, which cause other responses that in the context of inducing hyperemia for radionuclide imaging, are considered side effects.
Caffeine and methyl xanthenes bind to the adenosine receptors without causing stimulatory or inhibitory effects, but effectively block binding and stimulation by adenosine. If they are present when adenosine is administered, the vasodilatory effects are blocked; flow heterogeneity is not created and in patients with CAD results in a false-negative test. This blocking effect, however, is helpful when side effects are present and administration of aminophylline blocks the adenosine receptors and reverses the effects.
Side Effects
Following adenosine or dipyridamole infusion, there is a 10-mm Hg decrease in systolic and diastolic blood pressure (14,15). The most common cardiac side effects with dipyridamole include chest pain (20%), ECG changes (16%), and ST depression (7.5%). With adenosine, the cardiac side effects included chest pain (35%), atrioventricular (AV) nodal block (7.6%), and ST-T wave changes (5.7%). The AV nodal block was transient in all patients. The majority of reported chest pain is not ischemic, but due to nonselective stimulation of bradykinin pain receptors by adenosine. Patients with ECG changes consistent with ischemia are felt to have a higher dependence on collateral vessel blood flow, which may be decreased owing to coronary steal associated with a marked increase in coronary flow in normal vessels during vasodilation and a resultant decrease in collateral flow. Side effects occurred in 50% of patients receiving dipyridamole and aminophylline reversal was required in 12% (16). At some institutions, it is standard practice to reverse the effects of dipyridamole in all patients using aminophylline. The effective half-life of dipyridamole is 30 minutes, which is longer than the half-life of aminophylline and this means additional doses may be required to prevent recurrence of side effects. During adenosine infusion, side effects included flushing in 36.5%, shortness of breath or dyspnea in 35.2%, GI discomfort in 14.6%, headache in 14.2%, and neck discomfort in 11.6%. The number of patients reporting any side effect was 80% (14). Aminophylline was seldom required (0.8%) following adenosine administration because of the ultrashort half-life of less than 10 seconds, which means side effects resolve almost immediately following termination of infusion.
TABLE 53.2 Physical Properties of Adenosine, Dipyridamole, and Dobutamine | ||||||||||||||||||||||||
---|---|---|---|---|---|---|---|---|---|---|---|---|---|---|---|---|---|---|---|---|---|---|---|---|
|
Safety of Pharmacologic Stress Testing
Dipyridamole and adenosine have been used extensively in the United States and have an excellent overall safety record. Although general side effects are common, cardiac and severe adverse events are rare. When considering that patients referred for pharmacologic stress testing are generally more debilitated, overall safety is comparable to that reported for conventional dynamic exercise stress testing (14,15). Severe adverse complications such as death and myocardial infarction in association with pharmacologic stress testing are uncommon. In a retrospective review of 73,806 patients from 59 centers in 19 countries, there were 9 reported cardiac deaths and 13 nonfatal myocardial infarctions using intravenous dipyridamole (15). In a prospective registry that included 9,256 patients receiving intravenous adenosine, there were no deaths; 1 patient had a myocardial infarction (14).
Efficacy
The reported diagnostic accuracy using adenosine or dipyridamole has been shown to be comparable to exercise. In early studies performed mostly using planar Tl-201 imaging in which each patient was studied twice with exercise and dipyridamole, sensitivity was in the 80% range and specificity
in the range of 90%. There were no differences between the two forms of stress (17). The published studies looking at the accuracy of adenosine stress did not in general perform stress twice and used predominately SPECT imaging with Tl-201 or the Tc-99m tracers. The average sensitivity for pharmacologic stress with vasodilators was 87% and the specificity was 74% (17). Based on extensive publications and practical clinical experience in everyday use, the accuracy of vasodilator pharmacologic stress is accepted as being equivalent to exercise stress.
in the range of 90%. There were no differences between the two forms of stress (17). The published studies looking at the accuracy of adenosine stress did not in general perform stress twice and used predominately SPECT imaging with Tl-201 or the Tc-99m tracers. The average sensitivity for pharmacologic stress with vasodilators was 87% and the specificity was 74% (17). Based on extensive publications and practical clinical experience in everyday use, the accuracy of vasodilator pharmacologic stress is accepted as being equivalent to exercise stress.
Adenosine and Dipyridamole Pharmacologic Stress Protocols
Figures 53.5 and 53.6 demonstrate the protocols for using adenosine and pharmacologic stress in combination with a 1-day Tc-99m radiotracer. Patients should fast a minimum of 8 hours and medications and foods containing caffeine should be stopped for 12 to 24 hours before testing. Methylxanthines and dipyridamole need to be stopped for 48 hours prior to testing because they inhibit the vasodilatory effects of the adenosine and dipyridamole and patients taking dipyridamole can have a severe response to adenosine. Patients with second-degree AV nodal block (Mobitz type II) or third-degree AV nodal block without a functioning pacemaker should not receive adenosine because of the potential AV node blocking effects. Patients with a prolonged PR interval or Wenckebach can be tested safely. The use of vasodilator stress is recommended in all patients with a left bundle branch block (LBBB); the use of exercise leads to a higher rate of false-positive studies due to septal defects (5). This is felt to be mediated by a decrease in blood flow through septal perforators at high heart rates, which is not present at rest.
Adenosine is infused at a rate of 140 μg/kg for minute for 4 or 6 minutes with the radiotracer injected at minutes 2 or 3 and the infusion continued a minimum of 1 minute to allow maximal extraction, especially of the Tc-99m tracers which have a lower extraction fraction then Tl-201 across the coronary bed (Figure 53.8). If the patient has evidence of ischemia by symptoms or profound ECG changes, the radiotracer can be administered earlier, but the infusion must be maintained a minimum of 1 minute to allow radiotracer extraction at the high coronary blood flow rates. The ultrashort half-life of adenosine (<10 seconds) quickly reverses side effects and intravenous aminophylline is rarely required. Performing low-level exercise during adenosine infusion decreases side effects, improves image quality, and is recommended for all patients capable of walking on a treadmill.
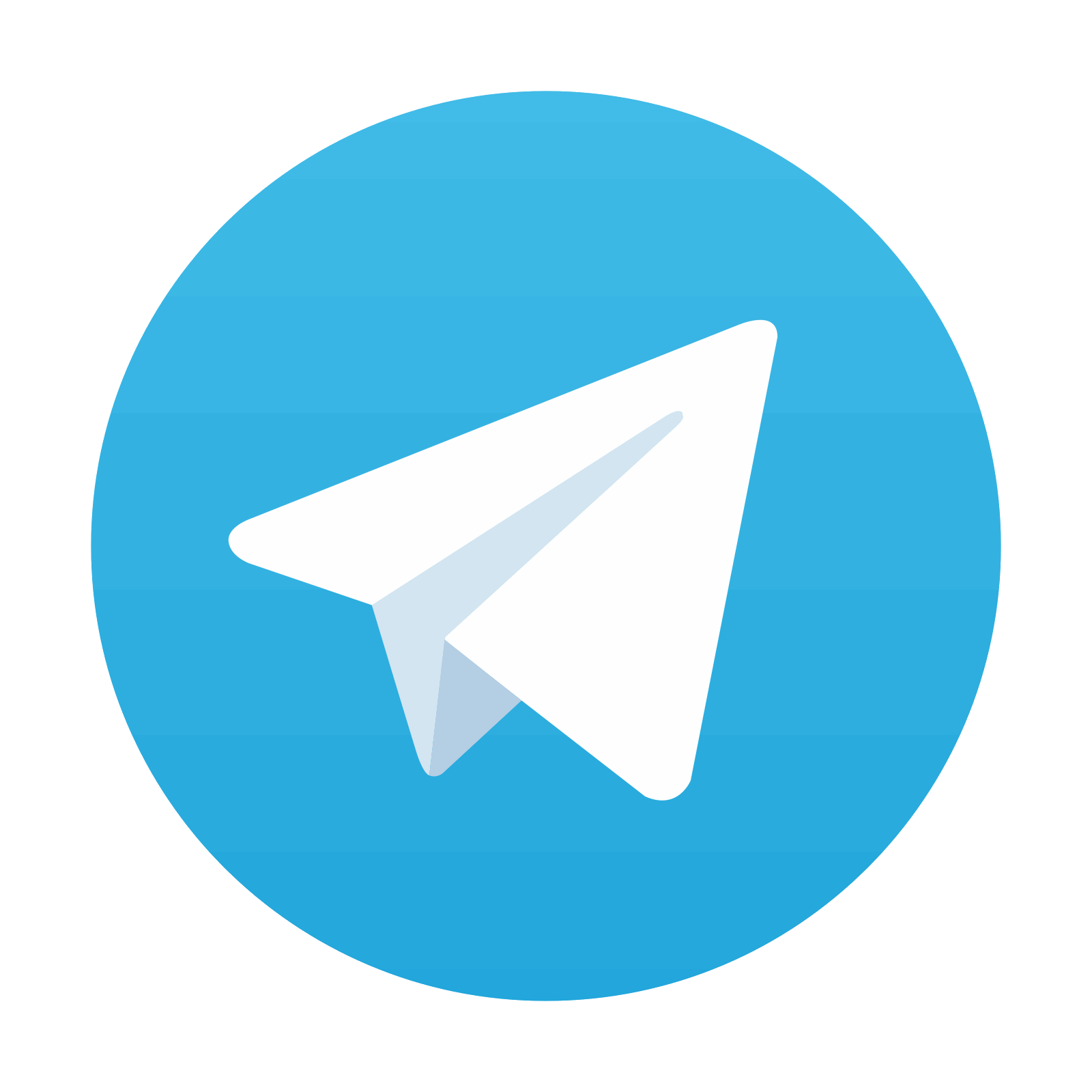
Stay updated, free articles. Join our Telegram channel

Full access? Get Clinical Tree
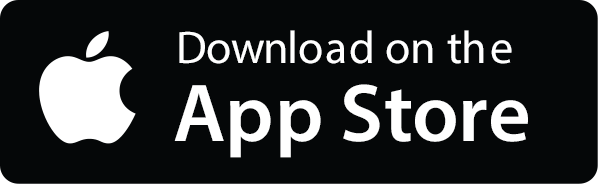
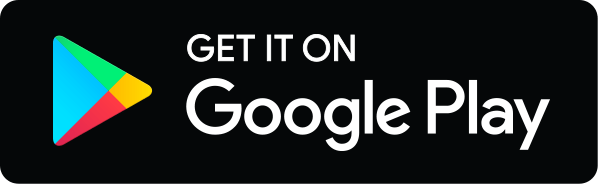
