Introduction
The term interstitial lung disease (ILD), in general, implies the clinical manifestation of inflammatory-fibrotic infiltration of the alveolar walls (septa) resulting in profound effects on the capillary endothelium and the alveolar epithelial lining cells ( Fig. 63-1 ). Under normal conditions, small numbers of interstitial macrophages, fibroblasts, and myofibroblasts reside within the interstitium. Other components of the interstitium include the matrix proteins of the lung, consisting of collagen-related macromolecules and the noncollagenous proteins such as fibronectin and laminin.

In many of ILDs, interstitial fibrosis follows injury to the gas-exchanging units. This injury increases alveolar permeability, enabling the serum contents to enter the alveolar spaces resulting in airspace abnormalities in addition to the interstitial changes. Fibroblastic proliferation and excessive collagen deposition, the histologic hallmarks of ILD, either result directly from the initial injury, from the inflammatory cell response that releases proinflammatory and profibrotic cytokines, or from the regenerative and reparative processes taking place at the epithelial and endothelial surfaces. Moreover, fibroblastic proliferation and collagen accumulation also takes place within airway and alveolar lumina and walls of small airways (alveolar ducts, respiratory bronchioles, and terminal bronchioles).
ILDs include many entities that injure the lung parenchyma, producing diseases with similar clinical, radiographic, and physiologic features. Some develop in the setting of other conditions, such as connective tissue disease; others are idiopathic. The primary goal of the clinician is to determine the cause or underlying disease whenever possible and, if not, at least a diagnosis that allows prognostication and a management plan.
Clinical and Histologic Classification of Interstitial Lung Disease
The exact prevalence and incidence of ILDs are unknown. Studies suggest a prevalence of 81/100,000 for men compared with 67/100,000 for women. Similarly, the overall incidence of ILD is slightly more common in men (32/100,000/yr) than in women (26/100,000/yr) and increases with age. For example, among men and women 75 years of age or older, the prevalence of idiopathic pulmonary fibrosis (IPF) was 250/100,000 and the incidence was 160/100,000/yr.
ILDs can be classified according to clinical, histopathologic, or radiologic parameters. A clinical classification of ILDs is shown in Table 63-1 . Although the diagnosis of ILD due to occupational exposure, a medication, or a connective tissue disease may be obvious, primary and idiopathic ILDs can be difficult to diagnose on clinical grounds alone. This chapter presents an approach for the evaluation of these patients with emphasis on idiopathic interstitial pneumonias (IIPs). An alternative method of histopathologic classification depends on the pattern of injury found on lung biopsy. As seen in Table 63-2 , dissimilar clinical entities may result in similar histologic appearances.
IDIOPATHIC FIBROTIC DISORDERS |
|
CONNECTIVE TISSUE DISEASE–ASSOCIATED ILDs |
|
TREATMENT-RELATED OR DRUG-INDUCED ILDs |
|
HEREDITARY ILDs |
|
OTHER CAUSES of ILD |
|
PRIMARY (UNCLASSIFIED) ILDs |
|
OCCUPATIONAL AND ENVIRONMENTAL ILDs |
Inorganic |
|
Organic (hypersensitivity pneumonitis) |
|
Histologic Patterns | Clinical Associations |
---|---|
Usual interstitial pneumonia | Idiopathic pulmonary fibrosis; connective tissue diseases (uncommon); asbestosis; hypersensitivity pneumonitis; chronic aspiration pneumonia; chronic radiation pneumonitis; Hermansky-Pudlak syndrome; neurofibromatosis |
Nonspecific interstitial pneumonia | Idiopathic; connective tissue diseases; drugs; hypersensitivity pneumonitis; resolving diffuse alveolar damage; AIDS; infections |
Diffuse alveolar damage | Acute interstitial pneumonia (Hamman-Rich syndrome); acute respiratory distress syndrome; drugs (cytotoxic agents, heroin, cocaine, paraquat, ethchlorvynol, aspirin); toxic gas inhalation; radiation therapy; oxygen toxicity; connective tissue disease; infections ( Legionella, Mycoplasma, viral) |
Organizing pneumonia | Cryptogenic organizing pneumonia; organizing stage of diffuse alveolar damage; organizing infections (e.g . , influenza) as part of diffuse alveolar hemorrhage; drugs (amiodarone, cocaine); infections; connective tissue diseases; hypersensitivity pneumonitis; eosinophilic pneumonia; granulomatosis with polyangiitis (Wegener) |
Desquamative interstitial pneumonia/respiratory bronchiolitis | Cigarette smoking; idiopathic; connective tissue diseases; primary pulmonary Langerhans cell histiocytosis; asbestosis; hard-metal pneumoconiosis (cobalt); Gaucher disease; Niemann-Pick disease; Hermansky-Pudlak syndrome; drugs (nitrofurantoin, amiodarone) |
Lymphocytic interstitial pneumonia | Idiopathic; hypogammaglobulinemia; autoimmune diseases, including Hashimoto thyroiditis, lupus erythematosus, primary biliary cirrhosis, Sjögren syndrome, myasthenia gravis, chronic active hepatitis; AIDS; allogeneic bone marrow transplantation |
Eosinophilic pneumonia | Idiopathic acute and chronic; tropical filarial eosinophilia; parasitic infections; allergic bronchopulmonary aspergillosis; eosinophilic granulomatosis with polyangiitis (Churg-Strauss syndrome); hypereosinophilic syndrome; AIDS |
Alveolar proteinosis | Pulmonary alveolar proteinosis; acute silicosis; aluminum dust; AIDS; myeloproliferative disorder |
Diffuse alveolar hemorrhage (with capillaritis) | Granulomatosis with polyangiitis (Wegener); microscopic polyangiitis; systemic lupus erythematosus; polymyositis; scleroderma; rheumatoid arthritis; mixed connective tissue disease; lung transplantation; drugs (retinoic acid, propylthiouracil, dilantin); Behçet disease; cryoglobulinemia; Henoch-Schönlein purpura; pauci-immune glomerulonephritis; immune complex glomerulonephritis |
Amyloid deposition | Primary amyloidosis; multiple myeloma; lymphocytic interstitial pneumonia, lymphoma |
Granuloma | Sarcoidosis; hypersensitivity pneumonitis; pulmonary Langerhans cell histiocytosis; intravenous talcosis; berylliosis; lymphocytic interstitial pneumonia; infections |
The number of ILDs is daunting, but they are linked by many common features: clinical presentation, radiographic appearance, physiologic abnormalities, and, in some instances, histologic findings. Nevertheless, a specific diagnosis can be made in many patients from the results of a careful history and certain laboratory tests. Bronchoscopy with bronchoalveolar lavage (BAL) and, often, transbronchial biopsy are useful in the diagnosis of some causes of interstitial infiltration. Thoracoscopic or open-lung biopsy is required for a definitive diagnosis of the remaining cases. Further information about many of the specific members of the interstitial disease family in this section is found in Chapter 65 , Chapter 66 , Chapter 67 , Chapter 68 . Additional discussion of other causes of ILD is found elsewhere in the book: the pneumoconioses in Chapter 73 , hypersensitivity pneumonitis in Chapter 64 , and drug-induced pulmonary disease in Chapter 71 .
Clinical, Radiologic, and Physiologic Features of Interstitial Lung Diseases
The hallmarks of an ILD are progressive dyspnea and cough, an abnormal chest radiograph, and impaired pulmonary function tests. However, 5% to 10% of symptomatic patients eventually diagnosed as having ILD have normal chest radiographs at the time of presentation. There are also dyspneic patients with or without abnormal chest radiographs in whom routine pulmonary function tests (flows, volumes, and diffusing capacity) are normal. Some patients with ILD (e.g., sarcoidosis) on chest imaging may have no associated symptoms or pulmonary function abnormalities. Exercise testing, which stresses the cardiopulmonary system and measures gas exchange, may unmask abnormalities in these situations. Furthermore, high-resolution computed tomography (HRCT) scans and BAL can detect abnormalities in the presence of normal radiographs and physiologic tests in patients at high risk for development of ILD, such as those with connective tissue disease, asbestos exposure, or hypersensitivity pneumonitis, and those taking drugs known to injure the lung.
Past History
ILD developing in a patient with an established connective tissue disease is obvious; however, there are patients in whom the lung disease precedes the more typical manifestations of the associated systemic disease by months to several years.
Occupational and Environmental History
The occupational and environmental history is of obvious importance. It should be thorough and detailed because a long latency period may occasionally exist between exposure and the appearance of clinical impairment and disability. The exposure may have been of short duration but high intensity. Hypersensitivity pneumonitis, which can manifest either as recurrent acute or subacute pneumonitis or as an insidious form with slowly progressive dyspnea, must be excluded (see Chapter 64 ). A growing list of occupational and environmental antigens can cause granulomatous pneumonitis.
Drug History
A review of the medications used in the recent and distant past is important. Uncommonly, lung disease may appear weeks to years after the drug has been discontinued (see Chapter 71 ). Aspiration (often silent) of gastric contents because of gastroesophageal reflux (GER) can lead to the insidious development of ILD, as can the nocturnal use of oily nose drops or use of mineral oil as a laxative.
Smoking History
Determining any history of tobacco use is important. More than 90% of patients with pulmonary Langerhans cell histiocytosis (PLCH) of the lung are smokers at the time of diagnosis; this is also true for respiratory bronchiolitis. Of patients with Goodpasture syndrome who smoked, 100% had diffuse alveolar hemorrhage, whereas only 20% of a nonsmoking group had pulmonary disease in addition to the renal involvement. Tobacco use also appears to enhance the development of interstitial fibrosis in an asbestos-exposed population. The risk of asbestosis in exposed smokers was 13 times that in a nonsmoking asbestos-exposed cohort. Most patients with IPF have a history of tobacco abuse. Conversely, hypersensitivity pneumonitis infrequently appears in the active smoker. Also, the incidence of sarcoidosis is lower in smokers.
Family History
Familial associations (with an autosomal dominant pattern) have been identified in cases of IPF, sarcoidosis, tuberous sclerosis, and neurofibromatosis; Niemann-Pick disease, Gaucher disease, and Hermansky-Pudlak syndrome are inherited in an autosomal recessive fashion (see “ Familial Pulmonary Fibrosis ” later).
Gender
There are ILDs with a sex predilection. Lymphangioleiomyomatosis arises almost exclusively in women. In addition, many connective tissue diseases more commonly affect women. Occupational causes are more likely in men. However, transmission of inorganic dust, either from clothes or by living in the vicinity of a manufacturing or mining facility, has resulted in asbestosis and berylliosis in women, children, and men not employed in the industry.
Symptoms
Progressive dyspnea is usually the most common symptom, but cough can be prominent, particularly in those patients with lymphangitic carcinomatosis in which the bronchial lymphatic channels are infiltrated.
Cough is a prominent symptom in ILDs, especially those that affect small airways or are bronchiolocentric in location, such as sarcoidosis, respiratory bronchiolitis, organizing pneumonia, PLCH, and hypersensitivity pneumonitis. In some ILDs such as IPF, cough can be debilitating.
Wheezing, an unusual symptom in ILD, has been reported with lymphangitic carcinomatosis, chronic eosinophilic pneumonia, respiratory bronchiolitis, and hypersensitivity pneumonitis.
Substernal chest pain, an unusual complaint for most ILDs, is frequent in sarcoidosis. Pleuritic-type chest pain may accompany connective tissue and drug-related ILDs. The sudden appearance of chest pain due to a pneumothorax can be the presenting manifestation or complicate preexisting PLCH, lymphangioleiomyomatosis, tuberous sclerosis, or neurofibromatosis.
Hemoptysis is typical for the diffuse alveolar hemorrhage syndromes, lymphangioleiomyomatosis, pulmonary veno-occlusive disease, and long-standing mitral valve disease. However, alveolar hemorrhage may be present without hemoptysis. Hemoptysis in a patient with an established ILD raises the possibility of a complicating malignancy.
Symptoms in patients with ILD are present for months to years and progress at varying rates. Several interstitial reactions are acute (days to several weeks). These are often confused with atypical pneumonias because they cause diffuse radiographic opacities and may be associated with fever. Included are acute interstitial pneumonia (AIP; Hamman-Rich syndrome), acute eosinophilic pneumonia, some cases of hypersensitivity pneumonitis, occasionally drug-related ILDs, some cases of organizing pneumonia, the diffuse alveolar hemorrhage syndromes, and the acute immunologic pneumonias seen with connective tissue diseases.
Physical Findings
The most typical physical finding is bibasilar inspiratory crackles. Crackles are less likely in the granulomatous diseases. Bilateral inspiratory crackles may also be present in a symptomatic patient with a negative chest radiograph. Clubbing of the digits, which in most cases indicates advanced fibrotic disease, is a common finding in patients with the idiopathic or familial forms of pulmonary fibrosis. However, the appearance of digital clubbing in a patient with an established case of ILD could indicate an underlying bronchogenic carcinoma. With advanced fibrosis causing chronic hypoxemia, clinical signs of pulmonary hypertension and cor pulmonale appear. Attention to potential extrapulmonary physical findings or other manifestations may reveal a specific diagnosis ( Table 63-3 ).
Finding | Examples |
---|---|
Systemic hypertension | Connective tissue disease; neurofibromatosis; some diffuse alveolar hemorrhage syndromes |
Erythema nodosum | Sarcoidosis; connective tissue disease; Behçet syndrome |
Maculopapular rash | Drug-induced; amyloidosis; lipoidosis; connective tissue diseases; Gaucher disease |
Heliotrope rash | Dermatomyositis |
Albinism | Hermansky-Pudlak syndrome |
Discoid lupus | Systemic lupus erythematosus |
Neurofibroma | Neurofibromatosis |
Telangiectasia | Scleroderma |
Raynaud phenomenon | Connective tissue disease |
Cutaneous vasculitis | Systemic vasculitides; connective tissue disease |
Subcutaneous nodules | Neurofibromatosis; rheumatoid arthritis |
Calcinosis | Dermatomyositis; scleroderma; amyloidosis |
Uveitis | Sarcoidosis; Behçet syndrome; ankylosing spondylitis |
Scleritis | Systemic vasculitis; systemic lupus erythematosus; scleroderma; relapsing polychondritis; sarcoidosis |
Keratoconjunctivitis sicca | Lymphocytic interstitial pneumonia (Sjögren syndrome) |
Salivary gland enlargement | Sarcoidosis, lymphocytic interstitial pneumonia (Sjögren syndrome) |
Peripheral lymphadenopathy | Sarcoidosis; lymphangitic carcinomatosis; lymphocytic interstitial pneumonia; lymphoma |
Hepatosplenomegaly | Sarcoidosis; pulmonary Langerhans cell histiocytosis; connective tissue disease; amyloidosis; lymphocytic interstitial pneumonia |
Pericarditis | Radiation pneumonitis; connective tissue disease; systemic vasculitis |
Myositis | Connective tissue disease; drugs (L-tryptophan) |
Bone involvement | Pulmonary Langerhans cell histiocytosis; sarcoidosis; Gaucher disease; lymphangitic carcinomatosis |
Arthritis | Connective tissue disease; systemic vasculitis; sarcoidosis |
Diabetes insipidus | Pulmonary Langerhans cell histiocytosis; sarcoidosis |
Glomerulonephritis | Systemic vasculitis; connective tissue disease; Goodpasture syndrome; sarcoidosis |
Nephrotic syndrome | Amyloidosis; drug-induced (gold, penicillamine); systemic lupus erythematosus |
Renal mass | Lymphangioleiomyomatosis; tuberous sclerosis |
Radiologic Features
Although standard chest radiography is not as sensitive for detection of ILD as the HRCT scan, it is the logical starting point and the initial way of identifying and defining disease.
Chest Radiography
Ziskind and coworkers classified diffuse lung diseases according to the pattern on chest radiographs—alveolar filling and primarily interstitial patterns (reticular or nodular). Although computed tomography (CT) scanning has supplanted the chest radiograph in the assessment of diffuse parenchymal lung diseases, it is still useful to recognize these radiographic patterns.
Alveolar Filling Pattern.
Alveolar filling ( Table 63-4 ) is recognized by a homogeneous opacity that can be diffuse or patchy and is characterized by confluent nodules with ill-defined outer borders, air bronchograms, and obliteration or silhouetting of normal structures such as the diaphragm, heart, and intrapulmonary blood vessels ( Fig. 63-2 ). Another feature occasionally seen with alveolar filling is the air alveologram, which represents small areas of uninvolved lung in an area of incomplete consolidation, manifesting on the chest radiograph ( Fig. 63-3 ) as small lucent areas within areas on increased lung opacity due to consolidation. So-called “acinar rosettes,” also referred to as “acinar nodules” or “air space nodules,” may be seen when alveolar filling is present and represent centrilobular or peribronchial nodules, rather than actual opacification of individual acini. Several have associated hilar adenopathy (e.g., sarcoidosis, pulmonary lymphoma). In patients with alveolar proteinosis, sparing of the lung parenchyma immediately adjacent to the diaphragm is seen. In chronic eosinophilic pneumonia, the pattern has been referred to as the radiographic negative of pulmonary edema because the alveolar opacities are most prominent in the periphery. A similar alveolar pattern has also been reported in some patients with organizing pneumonia ( Fig. 63-4 ).
Alveolar proteinosis (proteinaceous fluid) |
Adenocarcinoma with lepidic pattern or mucinous type (formerly called bronchoalveolar carcinoma) (malignant cells) |
Bronchioloalveolar metastases (malignant cells from pancreas, breast) |
Pulmonary lymphoma (malignant lymphocytes) |
Lymphocytic interstitial pneumonia (lymphoplasmacytic cells) |
Alveolar sarcoid (lymphocyte-macrophage alveolitis or confluent granuloma) |
Desquamative interstitial pneumonia (macrophages) |
Diffuse alveolar hemorrhage (red blood cells; hemosiderin-filled macrophages) |
Eosinophilic pneumonia (eosinophils, macrophages; lymphocytes) |
Alveolar microlithiasis (calcium-phosphate microliths) |
Organizing pneumonia (Masson bodies) |
Exogenous lipoid pneumonia (lipid-filled macrophages) |
Acute hypersensitivity pneumonia (lymphoplasmacytic cells) |



Interstitial Pattern.
Radiographic interstitial opacities become apparent when the interstitial compartment becomes infiltrated and widened by inflammatory cells, excessive collagen, granulomatous inflammation, or smooth muscle proliferation. In other instances, malignant cells or amyloid deposits expand this compartment. These opacities appear as nodules, linear reticular opacities, or a combination of linear shadows and nodules (“reticulonodular” opacities).
Nodular Pattern.
Nodules of varying size characterize granulomatous lung diseases. Miliary nodules accompany infectious granulomas and noninfectious granulomas (e.g., sarcoidosis, PLCH, and hypersensitivity pneumonitis) and some malignant diseases (e.g., melanoma, hypernephroma, and lymphoma) ( Fig. 63-5 ). However, linear opacities possibly representing an underlying cellular interstitial infiltrate are also visible.

Linear or Reticular.
Linear or reticular interstitial changes are seen in most ILDs ( Fig. 63-6 ). It is typical for many ILDs (e.g., IPF, the connective tissue diseases, asbestosis, cytotoxic drug–induced disorders) to have the greatest concentration of the reticular opacities in the lower lung zones. The term radiographic honeycomb lung refers to a reticular and cystic pattern that correlates with the histologic “honeycombing.” These small cystic structures, which are best seen in the lower and peripheral lung zones, indicate an underlying advanced fibrotic change ( Fig. 63-7 ).


A criticism of the alveolar-interstitial classification is that a mixed pattern is often found. For example, interstitial fibrosis may eventually be superimposed on a disease that was primarily alveolar, such as alveolar proteinosis, diffuse alveolar hemorrhage, eosinophilic pneumonia, or organizing pneumonia. Sarcoidosis, a disease characterized by interstitial granuloma, can be alveolar in appearance due to either coalescence of granulomas with compression of adjacent lung or a lymphocyte-macrophage alveolitis. Dense fibrosis from any fibrotic lung disease can compress adjacent lung, producing homogeneous shadows. The appearance of alveolar opacities during the course of an ILD may represent renewed activity of the primary disease, superimposed infection, or development of adenocarcinoma.
Other Radiographic Features.
Several distinctive patterns may accompany the interstitial changes and may point to a diagnosis ( Table 63-5 ). There are a group of interstitial diseases, often granulomatous, in which the radiographic changes are more prominent in the upper lung zones ( Fig. 63-8 ). Some interstitial diseases are bronchiolocentric, resulting in either maintenance or expansion of the lung volume. If an ILD is superimposed on emphysema, the lung volumes are often preserved. The majority of ILD s result in a gradual reduction of the lung volumes. In fact, lower lobe volume loss and traction bronchiectasis are typical for the advanced stages of these diseases. Short horizontal lines at the lung periphery (Kerley B lines), representing thickened interlobular septa, are seen after obstruction of the pulmonary lymphatics ( Fig. 63-9 ). Pneumothorax is often the presenting manifestation of PLCH or lymphangioleiomyomatosis. Diaphragmatic pleural calcification in a patient with interstitial opacities is indicative of asbestos exposure. Pleural thickening and pleural effusion may complicate the course of the connective tissue–associated ILDs. Chronic hypoxemia results in radiographic evidence of pulmonary hypertension.
Feature | Diseases |
---|---|
Upper zone—predominant disease | Radiation pneumonitis; neurofibromatosis; chronic sarcoidosis; pulmonary Langerhans cell histiocytosis; silicosis; coal workers’ pneumoconiosis; chronic hypersensitivity pneumonitis; chronic eosinophilic pneumonia; ankylosing spondylitis; nodular rheumatoid arthritis; berylliosis; drug-induced (amiodarone, gold, bischloroethyl carmustine nitrosourea [carmustine]); radiation |
Increased lung volumes | Lymphangioleiomyomatosis (with or without tuberous sclerosis); chronic sarcoidosis; pulmonary Langerhans cell histiocytosis; neurofibromatosis |
Radiologic honeycomb lung | Idiopathic pulmonary fibrosis; connective tissue disease; asbestosis; drug-induced; lymphocytic interstitial pneumonia; chronic aspiration pneumonia; hemosiderosis; Hermansky-Pudlak syndrome |
Pneumothorax | Pulmonary Langerhans cell histiocytosis; lymphangioleiomyomatosis (with or without tuberous sclerosis); neurofibromatosis |
Kerley B lines | Lymphangitic carcinomatosis; lymphangioleiomyomatosis; left atrial hypertension (mitral valve disease, veno-occlusive disease); lymphoma; amyloidosis |
Lymphadenopathy | Sarcoidosis; lymphoma; lymphangitic carcinomatosis; lymphoid interstitial pneumonia; berylliosis; amyloidosis; Gaucher disease |
Pleural disease | Lymphangitic carcinomatosis; connective tissue disease; asbestosis (pleural calcification); lymphangioleiomyomatosis (chylous effusion); drug-induced (nitrofurantoin, radiation); sarcoidosis |
Eggshell calcification of lymph nodes | Silicosis; sarcoidosis; radiation |


Computed Tomography
Conventional chest radiographic assessment for ILD may miss up to 10% of cases. Conventional CT, obtained using 8- to 10-mm slices, offers little more for the detection of radiographic-negative ILD. HRCT, obtained with a section thickness of less than 2 mm, enables better visualization of fine parenchymal detail and therefore the detection of early air space filling or interstitial change ( Fig. 63-10 ). In cases of suspected ILD with negative conventional radiography, it is important to perform HRCT in the prone and supine positions. Dependent lung density can mask interstitial change, and vascular engorgement of the dependent portion of lung mimics septal thickening on supine imaging. Abnormalities that persist on prone imaging are indicative of disease.

In patients with abnormal chest radiographs, the diagnostic accuracy increases with HRCT evaluation. In cases of IPF, peripheral reticular opacities, lower zone subpleural honeycombing, and traction bronchiectasis are seen ( Fig. 63-11 ). In the connective tissue diseases, asbestosis, and some drug-induced ILD, the results on HRCT, as on chest radiography, are indistinguishable from those of IPF or nonspecific interstitial pneumonia (NSIP). In scleroderma, a disease in which the prevalence rate of ILD approaches 100% in autopsy series, HRCT detects disease in 45% to 75% of patients when conventional radiography is negative.

In sarcoidosis, in addition to the hilar and mediastinal adenopathy, nodules deposited along bronchovascular bundles and interlobular septa, air space filling due to the lymphocyte-macrophage alveolitis, and linear densities secondary to fibrotic scarring can be seen ( Fig. 63-12 ). In patients with hypersensitivity pneumonitis, air space–filling centrilobular nodules and linear opacities without adenopathy are present. The HRCT can be normal in symptomatic patients with biopsy-proved hypersensitivity pneumonitis. In patients with PLCH, the combination of centrilobular nodules and cysts, most prominent in the upper lobes and occasionally accompanied by a pneumothorax, is characteristic ( Fig. 63-13 ). In earlier PLCH, coalescence of the nodules produces an air space–filling pattern.


In lymphangioleiomyomatosis, the HRCT is typical, revealing rounded, thin-walled cysts throughout ( Fig. 63-14 ). A pneumothorax or pleural effusion (chylous) may accompany this change. Identical findings are present in lymphangioleiomyomatosis associated with tuberous sclerosis. Lymphangitic carcinomatosis produces a beaded-chain appearance of the interlobular septa that correlates with Kerley B lines seen with conventional radiography. In asbestos-related disease, noncalcified pleural plaques and early ILD are often difficult to detect, and the HRCT is more sensitive than conventional chest radiography.

Functional Assessment
In ILD, there are characteristic alterations of the lungs’ mechanical properties and impairment of gas exchange at the alveolar-capillary interface. Assessment of ventilatory function and the mechanical properties of the lungs, as well as gas exchange, particularly during exercise, are vital components of the initial evaluation of patients with suspected ILD. In addition, serial measurements of function enable the physician to determine progression of the disease and the effects of therapeutic intervention.
Ventilatory Function
Ventilatory function tests provide an indirect index of alterations to the impedance to respiration offered by the elastic resistance to distention of the lungs and the frictional resistance to airflow in the tracheobronchial tree. Clinically, alterations of the mechanical properties of the respiratory system are also reflected in the pattern of breathing that is adopted by the patient. These patients tend to breathe rapidly and shallowly because a larger tidal volume would require an inordinate increase in work of breathing to overcome the greatly increased elastic resistance.
Elastic Resistance
ILD is associated with an increase in elastic resistance (or decreased compliance), and this can be seen in a plot of the static transpulmonary pressure (i.e., at points of no flow) at differing decrements of lung volume from total lung capacity (TLC) to residual volume. In most patients with ILD, the plot of the volume-pressure relationship of the lungs is characteristically shifted downward and to the right, with a reduced slope (i.e., compliance is low) and a markedly increased coefficient of elastic recoil (maximum elastic recoil pressure, TLC). Conversely, as is demonstrated in Figure 63-15 , there is significant variation in the position of the volume-pressure curve in patients with IPF and sarcoidosis, and the correlation between alterations of the elastic properties (and lung volume compartments) and the degree of fibrosis present is poor. This is, at least in part, a consequence of the impact of smoking, which appears to differ in the two disorders. In patients with IPF who smoke, the volume-pressure curve is shifted upward and to the left, whereas in patients with sarcoidosis who smoke, it is shifted downward and to the right.

Measurements of lung volume reflect changes in position of the volume-pressure curve of the lungs. This is because alterations of the elastic recoil of the lung or chest wall disturb the balance between the elastic forces of the lungs, which act in an expiratory direction, and those of the chest wall, which act in an inspiratory direction. In patients with ILD, the TLC, functional residual capacity, and residual volume are generally reduced ( Fig. 63-16 ). A lower than expected TLC (and vital capacity) in association with a normal functional residual capacity and a greater than expected residual volume generally reflects a mixed restrictive and obstructive disorder. However, because maximum effort is necessary to determine the inspiratory capacity and the expiratory reserve volume, a less than maximal inspiration or expiration, either because of weak respiratory muscles or because of poor effort, leads to the same findings. Similar considerations apply to the vital capacity, which is often used as an index of alterations of elastic resistance. In addition, as can be seen in Figure 63-16, a low vital capacity is not specific for restrictive disorders because it is also lower than expected in patients with airflow limitation (because of an increased residual volume).

Flow Resistance
Measurement of lung volume is important when evaluating airflow resistance. This can be assessed directly from the relationship between the rate of airflow and the resistive component of the transpulmonary pressure.
In clinical practice, various indirect measures of flow resistance can be used: the forced expiratory volume in 1 second (FEV 1 ); the mean expiratory flow rate between 25% and 75% of the forced vital capacity (FEF 25%-75%) ; the maximal expiratory flow rate ( ) at a particular proportion (such as 75%, 50%, or 25%) of the forced vital capacity (FVC) (from a flow-volume curve); the FEV 1 /FVC ratio; or the FEV 1 -to–vital capacity ratio. Low flow rates or a low FEV 1 /FVC ratio (i.e., <70% of predicted) is believed to indicate expiratory airflow limitation. However, like airway resistance,
values depend on the diameter of the airways, which is influenced by the lung volume. The FEV 1 /FVC ratio will be overestimated, and the degree of flow limitation underestimated, in the patient who does not exhale fully because of severe dyspnea or muscular weakness, pain, or poor effort.
Airflow resistance is not generally thought to be increased in ILD. As is seen in Figure 63-17 , is low in ILD, not because of an increase in flow resistance but rather because of the low lung volumes at which the flow is being measured. In fact, in uncomplicated ILD,
values are greater than expected at any particular lung volume because the lung elastic recoil pressure, which is the driving pressure for flow in the peripheral airways, is increased. As a corollary, a
lower than expected at a particular lung volume in a patient who is suffering from a restrictive disorder indicates an increase of flow resistance in the more peripheral airways. An increase in peripheral airway resistance has been reported in patients with IPF, hypersensitivity pneumonitis, and asbestos exposure.


Gas Exchange
Alterations of gas exchange are readily assessed by analysis of the arterial oxygen pressure (P o 2 ) and carbon dioxide pressure (P co 2 ) and calculation of the alveolar-arterial P o 2 difference ((A−a)P o 2 ), both at rest and during exercise.
In patients with ILD, arterial blood analysis while at rest usually reveals hypoxemia and an increased (A−a)P o 2 , along with hypocapnia. In addition, the diffusing capacity for carbon monoxide (D l CO ) is reduced, primarily because of a reduction in the alveolar-capillary surface available for gas exchange. The disturbance of gas exchange generally accompanies abnormalities in ventilatory function, but gas exchange may be normal at rest in a significant number of patients. Similarly, the resting D l CO may be only slightly reduced in patients who demonstrate a mild restrictive disorder or normal gas exchange while at rest, or both.
The D l CO is generally greater than expected in those cases associated with recent pulmonary hemorrhage because the red blood cells in the pulmonary alveoli take up carbon monoxide readily. Sequential measurements of the D l CO within minutes may aid in the establishment of the diagnosis of alveolar hemorrhage. When there is fresh blood in the alveolar spaces, each successive D l CO value will be lower as the hemoglobin in the alveoli becomes saturated with carbon monoxide.
Exercise
In most patients, gas exchange is disturbed during exertion, even in those with normal gas exchange at rest. Assessment of gas exchange during exercise provides the best correlation with the severity of disease and is probably the most important physiologic determination in patients with ILD (see Chapter 26 ).
In general, patients with the most severe restrictive disease have the poorest exercise tolerance. As is shown in Figure 63-18 , ventilation generally rises excessively during exercise and may approach the ventilatory ceiling. Characteristically, the respiratory frequency rises inordinately with increasing exercise loads because of the increased work that would be required to overcome the elastic resistance of the lungs if the tidal volume were to increase.


The excessive ventilation is frequently preferentially distributed to areas of lung that have normal compliance but diminished perfusion (i.e., high ventilation-perfusion ratio); as a result, unlike the normal response, the calculated dead space (V d ) and the ratio of dead space to tidal volume (V d /V t ) rise in association with rapid, shallow breathing. The increase in cardiac output with exercise and the rapid transit of blood through the pulmonary capillaries along with its redistribution in the lungs lead to a greater maldistribution of ventilation-perfusion ratios. This results in a rise in (A−a)P o 2 and a fall in arterial P o 2 ( Fig. 63-19 ). Except for heavy exercise, when the transit through the circulation is exceptionally rapid, it is unlikely that a reduced ability of oxygen to diffuse across a thickened alveolar-capillary membrane plays a significant role in the hypoxemia.


Laboratory Findings
Table 63-6 summarizes the results of laboratory tests that suggest or support the diagnosis of a specific ILD.
Finding | Diseases |
---|---|
Leukopenia | Sarcoidosis; connective tissue disease; lymphoma; drug-induced |
Leukocytosis | Systemic vasculitis; hypersensitivity pneumonitis; lymphoma |
Eosinophilia | Eosinophilic pneumonias; sarcoidosis; systemic vasculitis; drug-induced (sulfa, nitrofurantoin, methotrexate) |
Thrombocytopenia | Sarcoidosis; connective tissue disease; drug-induced; Gaucher disease; idiopathic pulmonary fibrosis |
Hemolytic anemia | Connective tissue disease; sarcoidosis; lymphoma; drug-induced; idiopathic pulmonary fibrosis |
Normocytic anemia | Diffuse alveolar hemorrhage syndromes; connective tissue disease; lymphangitic carcinomatosis |
Urinary sediment abnormalities | Connective tissue disease; systemic vasculitis; drug-induced |
Hypogammaglobulinemia | Lymphocytic interstitial pneumonia; granulomatous lymphocytic interstitial lung disease |
Hypergammaglobulinemia | Connective tissue disease; sarcoidosis; systemic vasculitis; idiopathic pulmonary fibrosis; asbestosis; silicosis; lymphocytic interstitial pneumonia; lymphoma |
Serum autoantibodies | Connective tissue disease; systemic vasculitis; sarcoidosis; idiopathic pulmonary fibrosis; silicosis; asbestosis; lymphocytic interstitial pneumonia |
Serum immune complexes | Idiopathic pulmonary fibrosis; lymphocytic interstitial pneumonia; systemic vasculitis; connective tissue disease; pulmonary Langerhans cell histiocytosis |
Serum angiotensin-converting enzyme | Sarcoidosis; hypersensitivity pneumonitis; silicosis; acute respiratory distress syndrome; Gaucher disease |
Antibasement membrane antibody | Goodpasture syndrome |
Antineutrophil cytoplasmic antibody | Systemic vasculitis |
Bronchoalveolar Lavage
BAL is a technique employed to sample the distal airways via the instillation of sterile saline through a wedged fiberoptic bronchoscope. After aspiration, the contents, which are thought to represent the cellular, immunologic, and biochemical milieu of the alveolar structures, can be analyzed. The results of BAL for the evaluation of ILD have been difficult to interpret because of the lack of standardized techniques for both the performance of the procedure and the subsequent analysis of the data. Furthermore, in some of the earlier studies, correlative data between BAL and lung biopsies were not obtained. Often, the treatment or smoking status of the patient was not considered. A multicenter publication has set forth the methods of performing and analyzing the results of BAL. This study involved a large cohort of normal subjects of varying age and race who were compared with patients with documented ILD. Smoking affects the BAL results of both normal subjects and patients by amplifying the macrophage and eosinophil populations.
BAL is a useful investigative tool in many ILDs. Cytologic analysis of BAL specimens can be diagnostic for lymphangitic carcinomatosis, adenocarcinoma, and pulmonary lymphoma. If the eosinophil level exceeds 40% (normal, <2%), eosinophilic pneumonia is usually the cause of the diffuse pulmonary infiltration. BAL eosinophilia of a lesser degree may be found with some connective tissue diseases, IPF, or organizing pneumonia.
The finding of periodic acid–Schiff–positive lipoproteinaceous bodies in the BAL was originally thought to be diagnostic for pulmonary alveolar proteinosis but has proved to be nonspecific. In cases of diffuse alveolar hemorrhage, red blood cells and hemosiderin-laden macrophages dominate the lavage specimen. Sequential BAL samples show an increase in red cells and in its bloody appearance (see Fig. 67-3 ).
BAL lymphocytosis (>35% lymphocytes) predominates in some diseases. Sarcoidosis and hypersensitivity pneumonitis are most common. Others include lymphocytic interstitial pneumonia (LIP), pulmonary lymphoma, berylliosis, and some drug-induced ILDs. Smaller but increased percentages of lymphocytes (normal range, 10%–15%) can be seen with these entities in addition to a number of other ILDs, including IPF, organizing pneumonia, connective tissue diseases, and some pneumoconioses. In patients with suspected asbestos-related disease, one or more asbestos bodies per high-powered field in the BAL specimen indicate significant exposure, although this does not establish the diagnosis of asbestosis. Because of the vertical gradient of asbestos bodies recovered in the lungs of patients with asbestosis, if lavage is used to document previous asbestos exposure, samples should be obtained from a basal segment of one of the lower lobes. A diagnosis of berylliosis is confirmed when lavaged lymphocytes undergo proliferation after exposure to beryllium in vitro.
In the BAL recovered from patients with PLCH, all inflammatory cells are increased even though their percentages remain unchanged. Electron microscopy demonstrates increased numbers of Langerhans cells. This monocyte, which is thought to be central in the pathogenesis, has a typical pentilaminar body (Birbeck granule) in the cytoplasm, as revealed by electron microscopy. Langerin and CD1a are specific diagnostic markers for Langerhans cells. Langerhans cells have been described in other fibrotic lung diseases, but not in numbers equivalent to those of PLCH.
Other applications of BAL include assessment of disease status and the prediction of therapeutic responsiveness. For example, in both the connective tissue diseases and IPF, BAL lymphocytosis is associated with a cellular histology (as opposed to fibrosis) and an improved response to treatment. Furthermore, the overall survival rate in these patients is increased. Conversely, the combination of lavage neutrophilia and eosinophilia without lymphocytosis often portends progressive unresponsive disease. In sarcoidosis, a disease characterized by increases in the number of T-helper (CD4 + ) lymphocytes in the lung, it has been suggested that clinical deterioration may be expected if the BAL lymphocyte level exceeds 28%; however, more recent data indicate that the level of BAL lymphocytes has no value in predicting the clinical outcome in sarcoidosis.
Another role for BAL is in the assessment of potential ILD in populations at risk. For example, in patients with scleroderma and rheumatoid arthritis in whom clinical, radiologic, and physiologic evidence of ILD is lacking, BAL studies have revealed increases in inflammatory cell populations.
Histologic Diagnosis
The final step in the evaluation of a patient with ILD is to decide whether tissue is necessary for diagnosis. As previously noted, the diagnosis of connective tissue, occupational, or drug-related ILD is often obvious after a careful history has been taken. In cases of idiopathic and primary ILD (see Table 63-1 ), the diagnosis may not be as obvious, although clinical, laboratory, and radiologic findings are often suggestive. Furthermore, the diagnosis of IPF, a commonly encountered ILD, in many cases can be established only by lung biopsy.
Transbronchial Biopsy
As a matter of practicality, transbronchial biopsy can be performed during the bronchoscopy for BAL. Transbronchial biopsy is relatively safe and is often diagnostic of sarcoidosis, diffuse malignancy, alveolar proteinosis, or eosinophilic pneumonia. Other entities are less frequently confirmed by this procedure. A transbronchial biopsy interpretation, which describes only inflammation, fibrosis, or both, is not evidence for IPF or any other entity listed in Table 63-1 . Furthermore, even in clinically confirmed cases of connective tissue or drug-induced ILD, several different histologic patterns may evolve (see Table 63-2 ), and surgical lung biopsy is often undertaken to predict prognosis and therapeutic responsiveness, particularly if BAL and HRCT are inconclusive.
Surgical Lung Biopsy
If the transbronchial biopsy, clinical, and BAL data are inconclusive and the patient is not at high risk, a video thoracoscopic or open-lung biopsy should be performed. Moreover, there is a poor correlation between the results of transbronchial and open-lung biopsies unless the transbronchial biopsy yields a specific diagnosis. Therefore, when the transbronchial biopsy is inconclusive and a definitive diagnosis is required, open or thoracoscopic lung biopsy is indicated.
Diagnosis and Management of Idiopathic Interstitial Pneumonias
IIPs represent a heterogeneous group of diffuse parenchymal lung diseases characterized by varying patterns of inflammation and fibrosis. This group of lung diseases represents a subset of ILDs and comprises seven clinical-radiologic-pathologic entities that are sufficiently different from one another to be designated as separate diseases. The classification, diagnosis, understanding of the pathogenic mechanisms, and management of IIP s continue to evolve. As a group, they can be distinguished from other forms of ILD by clinical methods including history, physical examination, chest radiologic and laboratory studies, and pathology. It is crucial for clinicians to be aware that the histologic pattern underlying these IIPs can be encountered in subjects with known causes for their lung disease (e.g., connective tissue diseases, smoking, drugs). Thus, usual interstitial pneumonia (UIP) can be seen not only in patients with IPF but also in patients with rheumatoid arthritis as a form of rheumatoid arthritis-associated ILD. In addition, the interstitial pneumonia patterns are associated with varying degrees of specificity with respect to etiology. For example, respiratory bronchiolitis–associated ILD (RB-ILD) in most patients is caused by smoking, whereas organizing pneumonia can be associated with a broad spectrum of causes including infections, aspiration, drug reactions, connective tissue disorders, eosinophilic lung disease, and many others. Tables 63-7 and 63-8 provide an overview of the key clinical and pathologic features of the IIPs.
Features | UIP | DIP | RB-ILD | AIP | NSIP | COP |
---|---|---|---|---|---|---|
Temporal appearance (age of lesion) | Variegated | Uniform | Uniform | Uniform | Uniform | Uniform |
Interstitial inflammation | Scant | Scant | Scant | Scant | Usually prominent | Usually moderate |
Fibrosis/honeycombing | Patchy | Variable, diffuse, moderate | Variable, focal, mild | Typically no, but may evolve to fibrosis | Variable, diffuse | No |
Fibroblast proliferation | Fibroblast foci prominent | No | No | Diffuse | Occasional, diffuse, or rare fibroblast foci | Within airways and air spaces (Masson bodies) |
Honeycomb change | Yes | No | No | No | Rare | No |
Intra-alveolar macrophage accumulation | Occasional, focal (smokers) | Yes, diffuse | Peribronchiolar | No | Occasional, patchy | Foamy macrophages common |
Hyaline membranes | No | No | No | Occasional, focal | No | No |
Features | UIP | DIP | RB-ILD | AIP | NSIP | COP |
---|---|---|---|---|---|---|
Mean age at onset (yr) | 60s | 40s | 40s | 50s | 50s | 50s |
Onset | Insidious | Insidious | Insidious | Acute | Subacute, insidious | Acute or subacute |
History of cigarette smoking | About two thirds | Most | Most | Not known | Uncommon | About half |
Mortality rate (mean survival) | 68% (5-6 yr) | 27% (12 yr) | 0% | 62% (1-2 mo) | ≈25% in 10 yr | 10% in 5 yr |
Response to steroids | Poor | Good | Good | Poor | Good | Excellent |
Complete recovery possible | No | Yes | Yes | Yes, rarely | Yes | Yes (≤70% of patients) |
Idiopathic Pulmonary Fibrosis
IPF is the most common form of IIP and accounts for 25% to 30% of ILDs. IPF is a well-defined clinical entity with characteristic clinical, radiographic, physiologic, and pathologic manifestations but is also a diagnosis of exclusion (i.e., UIP of unknown cause). The exact prevalence of this condition is unknown. In a recent study using narrow case-finding criteria, the prevalence ranged from 0.8 to 65/100,000 individuals; comparable figures for incidence were 0.4 to 27/100,000 persons. Extrapolating these rates to the overall U.S. population, prevalence was estimated to be 14/100,000 (incidence, 7/100,000). Both the prevalence and the incidence of IPF increase markedly with age.
Clinical Features
IPF is a disease of middle age, usually seen in patients between 50 and 70 years of age. Patients with familial pulmonary fibrosis tend to present at a younger age; otherwise, it is quite uncommon for a patient to present before the age of 40. The typical patient presents with the insidious onset of exertional breathlessness and a nonproductive cough. Constitutional symptoms are uncommon, but weight loss, fever, fatigue, myalgias, or arthralgia is occasionally present. Although patients may present with only a nonproductive cough, all patients experience dyspnea with exertion as the disease progresses. Most patients have these symptoms for months to years before definitive evaluation, usually around 12 to 18 months.
The physical examination is rarely normal. Most patients have bibasilar late inspiratory fine crackles (Velcro crackles) on chest examination. Clubbing of the fingers is seen in 40% to 75% of patients and is a late finding in the disease course. Cardiac examination is usually normal except in the middle or late stages of the disease, when findings of pulmonary hypertension (e.g., augmented P2, right-sided lift, tricuspid regurgitation, and S3 gallop) and cor pulmonale may become evident. Similarly, cyanosis is a late manifestation indicating advanced disease. Spontaneous pneumothorax or pneumomediastinum is rare.
Blood and Serologic Studies
An elevated erythrocyte sedimentation rate, low-level antinuclear antibodies titer positivity (≥40 and <1 : 320), and elevated rheumatoid factor (>60 IU/mL) have been identified in some of these patients. Hemoglobin level along with leukocyte and differential counts are usually normal.
Chest Imaging Studies
Chest Radiography.
The typical finding in patients with IPF is peripheral reticular opacities with a netlike appearance of linear or curvilinear densities, with predominance at the lung bases ( Fig. 63-20 ). A coarse reticular pattern or multiple cystic or honeycombed areas (i.e., coarse reticular pattern with translucencies measuring 0.3 to 1 cm in diameter) are radiographic findings that correlate with advanced disease and poor prognosis (see Figs. 63-6 and 63-7 ). Chest radiographic evidence of reduced lung volumes is usually present unless there is associated obstructive airway disease, as can be seen in smokers.

Pleural abnormalities are uncommon in IPF; their presence should suggest another diagnosis, such as collagen vascular disease (especially rheumatoid arthritis or systemic lupus erythematosus), mitral valve disease, congestive heart failure, asbestosis, infection, drug-induced lung disease, or lymphangitic carcinomatosis (see Table 63-5 ).
Computed Tomography Scan.
CT plays a major role in the evaluation of pulmonary parenchymal diseases, especially IPF. HRCT is useful in the differentiation of IPF from other ILDs, the determination of the extent and severity of disease activity, and, most importantly, the detection of disease, especially in patients with normal or minimal change on chest radiography. HRCT findings in IPF include a marked peripheral (subpleural) distribution of the interstitial opacities. The involvement is patchy, with areas of reticulation intermingled with areas of normal tissue, often associated with cystic spaces 2 to 4 mm in diameter (see Fig. 63-11 ). Early disease appears as patchy, predominantly peripheral, subpleural reticular opacities ( eFig. 63-1 ) and minor degrees of honeycomb change. In more advanced disease, a more diffuse reticular pattern in the lower lung zones with thickened interlobular septa and intralobular lines progresses to traction bronchiectasis and subpleural fibrosis ( eFig. 63-2 ). The predominantly basal nature of these abnormalities is often readily appreciated with coronal imaging ( eFig. 63-3 ).
One of the key findings indicating the diagnosis of IPF is the presence of honeycomb cysts in a basilar subpleural distribution ( eFig. 63-4 ). Honeycomb changes appear on the HRCT scan as variably sized cystic spaces that share walls and frequently stack on one another in several layers. Ground-glass opacities are common but of mild severity and characteristically much less extensive than the reticular pattern. Architectural distortion, which reflects lung fibrosis, is often prominent.
Combined lower lobe pulmonary fibrosis and upper lobe emphysema has been recently described as a new CT-defined syndrome ( eFig. 63-5 , ) ( Fig. 63-21 ). Smoking appears to be the predominant risk factor for this disorder. Patients with this entity are primarily males with a mean age of 65 years who have relatively preserved lung volumes but marked reductions in D l CO on pulmonary function testing. Limited data suggest that these patients with IPF and coexisting emphysema are more likely to require long-term oxygen therapy, to develop pulmonary hypertension, and to have a worse outcome than those without emphysema.

HRCT Correlation with Physiologic Data in ILD.
Identification of a relationship between pulmonary function testing and HRCT findings remains incomplete. FVC, D l CO , arterial P o 2 measured at peak exercise, and oxygen desaturation during exercise are the physiologic parameters that best correlate with the global extent of disease on HRCT. In one study, D l CO was the physiologic characteristic most highly correlated with HRCT findings. Serial HRCT scanning showed that the changes over time in the total extent of the disease were similar to those changes observed in D l CO and FVC.
Ability of HRCT Scanning to Diagnose ILD.
HRCT plays an important role but has not replaced lung biopsy in the diagnosis and assessment of most ILDs. In the case of IPF, connective tissue diseases (particularly scleroderma) and asbestosis may cause a similar HRCT appearance (except for the presence of parenchymal bands of fibrosis and pleural plaques seen in patients with asbestosis). Patients with chronic hypersensitivity pneumonitis can have similar reticular opacity or honeycombing, but often accompanied by a mosaic pattern, centrilobular nodules, and lack the bibasilar predominance seen in IPF ( eFig. 63-6 ).
Studies evaluating the ability of HRCT scanning to diagnose IPF accurately have found that HRCT significantly increases the level of diagnostic confidence. In general, the sensitivity for a confident diagnosis is low (≈48%) but the specificity is high (≈95%). The accuracy of a confident diagnosis of IPF made on HRCT by a trained observer appears to be about 90%. Less experienced observers are substantially less accurate than experienced observers. An HRCT pattern highly suggestive of the diagnosis of IPF is associated with a poor prognosis. Of those with a histopathologic diagnosis of UIP, those with basilar honeycombing have a worse survival than those without honeycombing. In a multivariate analysis of a large multicenter trial, it was found that extent of reticulation and honeycombing on HRCT is an important independent predictor of mortality in patients with IPF.
Other Imaging Techniques
Gallium Scanning.
Lung scanning with radioactive gallium ( 67 Ga) is a noninvasive test for staging the “alveolitis” found in ILD s , particularly sarcoidosis. However, 67 Ga lung scanning is not recommended in the routine evaluation of IPF because inflammation is not a prominent feature, the scan is difficult to interpret, the findings are not specific, and a negative scan does not exclude disease.
Ventilation-Perfusion Lung Scanning.
Ventilation-perfusion lung scanning is not recommended as a routine part of the evaluation. In most parenchymal diseases, ventilation-perfusion scanning reveals an inhomogeneous reduction of blood flow, ventilation, or both. There are two types of perfusion abnormalities in IPF: nonsegmental inhomogeneities, probably due to a localized loss of the capillary bed, most often in the lower lobes; and increased perfusion of the upper lung zones, resulting from pulmonary hypertension, which induces an upward shift in the gradient of capillary perfusion. Ventilation scans often reveal patchy, nonsegmental areas of decreased ventilation, reflecting regions of airway obstruction or alveolar destruction. Patchy areas of high and low ventilation-perfusion matching are usually seen, with a few areas of well-maintained ventilation-perfusion matching. These findings of mismatching of ventilation and blood flow help explain the hypoxemia and high V d /V t ratio found in many of these patients at rest.
Magnetic Resonance Imaging for Differentiating Inflammation and Fibrosis.
Magnetic resonance imaging (MRI) has several advantages over CT, including lack of ionizing radiation and ability to identify tissue characteristics at a nuclear level that may allow for novel assessments of lung function and microstructure. Ten patients with IPF and 16 with NSIP were examined by comparing 3-T MRI of the lung to the morphologic findings on surgical biopsy. Compared with fibrotic areas, inflammation-predominant areas showed an early enhancement pattern on dynamic studies and a high-signal intensity on T2-weighted triple-inversion black-blood turbo spin-echo images compared with fibrotic areas. These results indicate that qualitative analysis of MRIs may be helpful for differentiating inflammation- and fibrosis-predominant lesions.
Imaging of Fibrogenesis by Positron Emission Tomography.
The putative role of physiologic imaging using 18 F-2-fluoro-2-deoxy-D-glucose (FDG) positron emission tomography (PET) scans in IPF has been explored in few patients without clear results. In general, the lungs of patients with IPF appear to show increased uptake of FDG on PET ( eFig. 63-7 ). Some studies have suggested that cis-4- 18 F-fluoro-L:-proline [ 18 F-proline] may be a reliable marker for fibrosis formation. This radioligand has been tested in patients with IPF. PET acquisition was performed 1, 2, and 3 hours after injection of 18 F-proline. Surprisingly, low uptake of 18 F-proline was found in the lungs of all patients with IPF. The highest uptake was seen at 2 hours postinjection, with a decline at 3 hours past injection. The authors speculate that this low uptake may be due to the slow nature of fibrogenesis or to the relatively low dose of proline that can be used. Further research is necessary to know if imaging through PET has a role in the diagnosis or prognosis of ILD s .
Pulmonary Function Tests
The lung volumes (TLC, functional residual capacity, and residual volume) are reduced in IPF. Early on, the lung volumes may be normal, especially in patients with superimposed chronic obstructive pulmonary disease (COPD). Lung volumes are higher in smokers with IPF compared with those who have never smoked. As mentioned previously, a group of smokers develop a combined IPF and emphysema and show almost normal lung volumes, even in advanced disease. Expiratory flow rates (FEV 1 and FVC) may be decreased because of the reduction in lung volume, but the FEV 1 /FVC ratio is maintained. Because of the increased static elastic recoil found in these patients, flow rates (at any given lung volume) are often increased (see Figs. 63-15A and 63-17 ).
Patients with IPF are tachypneic, with rapid shallow breaths, probably because of increased work of breathing. This rapid respiratory rate presumably results from altered mechanical reflexes, caused by the increased elastic load, vagal mechanisms, or both, because no defined chemical basis for the hyperventilation has been identified (see Fig. 63-18 ).
The D l CO is reduced, often before the loss of lung volume. The decrease in the D l CO results from both a contraction of the pulmonary capillary volume and the presence of ventilation-perfusion abnormalities. Resting arterial blood gases are usually abnormal, revealing hypoxemia and respiratory alkalosis. The major cause of resting hypoxemia is ventilation-perfusion mismatching; it is not due to either impaired oxygen diffusion, as was originally suspected, or to anatomic shunts. With exercise, the (A−a)P o 2 widens, and the arterial P o 2 and oxygen saturation fall. During maximal exercise, 20% to 30% of the exercise-induced widening of the (A−a)P o 2 may be caused by some impairment of oxygen diffusion. Importantly, the abnormalities identified at rest do not accurately predict the magnitude of the abnormalities seen with exercise (see Fig. 63-19 ). In addition, gas exchange during exercise has been demonstrated to be a sensitive parameter for following the clinical course.
In patients with IPF, a highly significant correlation has been reported between the 6-minute walk distance (6MWD) test and D l CO , as well as ; the 6MWD is a strong predictor of mortality. The 6MWD test is difficult to perform in patients with advanced lung disease, however, and variability is common.
The 15-steps Climbing Oximetry Test has been found useful for estimating ventilatory reserve in patients with COPD and for predicting postoperative complications of lung resection. In a recent study, it was shown that the desaturation measured by the 15-steps Oximetry Test in IPF patients is comparable with the desaturation measured by the cardiopulmonary exercise test and the 6MWD test, suggesting it may be a reliable tool for monitoring IPF progression and for evaluating the need for oxygen supplementation. This test may serve as a surrogate marker of and an alternative to the 6MWD test in this setting.
During exercise, patients with IPF increase their minute ventilation primarily by increasing their respiratory frequency (see Fig. 63-18 ). This method of increase differs from that in normal subjects, in whom ventilation increases during mild exercise by an increase in the tidal volume (V t ) rather than respiratory rate. Thus, patients with IPF have elevated minute ventilation during exercise that is in part related to the increase in V d . In addition, the V d /V t ratio is increased at rest and is maintained or decreases only slightly with exercise. Occasionally, the V d /V t ratio increases in ILDs that have a prominent pulmonary vascular component, such as scleroderma or PLCH.
Pulmonary Hemodynamics
Recent evidence suggests pulmonary hypertension due to IPF (PH-IPF) is relatively common and may contribute substantially to functional status, quality of life, morbidity, and mortality. (See Chapter 59 .) The prevalence of PH complicating the course of patients with IPF has been reported in between 32% and 85% of patients, being more frequent in advanced disease. The most appropriate method to detect PH noninvasively is transthoracic (Doppler) echocardiography. Unfortunately, echocardiography may be inaccurate in estimating pulmonary arterial systolic pressure in patients with ILD. Right heart catheterization is the “gold standard” for the hemodynamic evaluation of the pulmonary circulation and diagnosis of PH but is an invasive technique. Right heart catheterization may be necessary, however, to document the severity of PH and potential right ventricular dysfunction. Abnormalities in gas exchange and exercise capacity seem to have a significant association with PH-IPF. Thus, exaggerated exercise oxygen desaturation, out-of-proportion reduction of D l CO , and supplemental oxygen requirement should raise suspicion of (and may be a useful surrogate for) the presence of underlying PH. Brain natriuretic peptide concentrations predicted moderate to severe PH with 100% sensitivity and high specificity (89%) in a small cohort of patients with pulmonary fibrosis. This study requires further validation.
Pathologic vascular findings in IPF consist of changes in the arteries, arterioles, and venules, as well as destruction of the capillary bed. Advential thickening around the pulmonary vessels reflects an increase of connective tissue. Smooth muscle cells hypertrophy and proliferate, and collagen and elastin accumulate in the media of the small muscular pulmonary arteries. Distal pulmonary arterioles become muscularized (see Fig. 59-4E,F ). In addition, there may be extensive intimal hyperplasia, fibrosis, and reduplication of the inner elastic lamina in the small muscular pulmonary arteries in IPF. Increased hemosiderin deposition and alveolar septal capillary density have been associated with higher right ventricular systolic pressure (as assessed by transthoracic echocardiography) and may represent histologic correlates of pulmonary hypertension in IPF.
Supplemental oxygen is the most obvious choice for prevention or treatment of PH; however, there are no data supporting favorable effects of oxygen on survival in PH-IPF. Vasodilators agents are currently being studied, but one must recognize that decreased physiologic vasoconstriction in low ventilation-perfusion lung units may worsen shunt and hypoxemia in IPF.
Endothelin-1 (ET-1) receptor antagonists have been useful in patients with other types of pulmonary hypertension, mostly in primary PAH or PAH associated with connective tissue disease. Clinical trials in PH-IPF are ongoing ( Table 63-9 ). Prostacyclin ( prostaglandin I 2 [PGI 2 ]) analogues used via inhalation could maintain (or even improve) ventilation-perfusion matching and could have a beneficial effect targeting PH. In one study, inhaled iloprost decreased mean pulmonary artery pressure without changes in shunt flow, suggesting the utility of selective pulmonary vasodilation in patients with PH-IPF. Sildenafil, a phosphodiesterase-5 inhibitor, promotes vasodilation and decreases smooth muscle proliferation and vascular remodeling. In an open-label study of treatment with sildenafil for 3 months in a small cohort of patients with PH-IPF, a modest but significant improvement in 6MWD was noticed. In a subsequent randomized clinical trial, sildenafil therapy did not show a benefit for the primary outcome (increase in 6MWD of 20% or more), but a small yet significant improvement was noted in arterial oxygenation, diffusing capacity, dyspnea, and quality of life. Several other randomized clinical trials are currently ongoing (see Table 63-9 ) to learn whether any therapies will be useful in PH-IPF (see Chapter 59 ).
Drug | Aim/Mechanism of Action | Sponsor | ClinicalTrials. Gov Identifier |
---|---|---|---|
QAX576 | It may down-regulate IL-13 | Novartis | NCT00532233 |
FG-3019 | It blocks CTGF | FibroGen | NCT00074698 |
Macitentan | Inhibitor of ET-1 | Actelion | NCT00903331 |
Bosentan | Indicated for PAH | UCLA; Actelion | NCT00625469 |
Pirfenidone | Antifibrotic effects in vitro and in experimental models | InterMune | NCT01366209 |
Zileuton | Inhibitor of leukotrienes | University of Michigan | NCT00262405 |
Gleevec (imatinib mesylate) | Tyrosine kinase inhibitor | Daniels, Craig E., MD, Novartis | NCT00131274 |
Octreotide (somatostatin analogue) | Anti-inflammatory and antifibrotic properties in vitro and in vivo | Institut National de la Santé et de la Recherche Médicale, France | NCT00463983 |
GC1008 | Antibody that neutralizes TGF-β | Genzyme | NCT00125385 |
Inhaled IFN-γ | Antifibrotic effects | New York University School of Medicine; National Center for Research Resources, Stony Brook University; Respironics | NCT00563212 |
Inhaled IFN-γ | Same as previous | New York University School of Medicine | NCT00212563 |
Tetrathiomolybdate | Copper chelating agent | University of Michigan; Coalition for Pulmonary Fibrosis | NCT00189176 |
Nintedanib | Inhibits profibrotic growth factors | Boehringer Ingelheim Pharmaceuticals | NCT01335177 |
NAC alone | Effect of the antioxidant NAC | NHLBI | NCT00650091 |
Thalidomide | Indicated for cough. Suppresses functional up-regulation of sensory fibers within the respiratory tract | Johns Hopkins University | NCT00600028 |
Sildenafil | Indicated for PAH | UCLA and Pfizer | NCT00625079 |
Sildenafil | Indicated for PAH | NHLBI; Pfizer | NCT00517933 |
Sildenafil | Indicated for PAH | Department of Veterans Affairs | NCT00359736 |
Inhaled iloprost | Indicated for PAH; secondary purpose: to evaluate antifibrotic properties | Actelion | NCT00109681 |
CNTO 888 | Phase II study | Centocor | NCT00786201 |
Losartan | An antagonist of angiotensin type 1 receptor | H. Lee Moffitt Cancer Center and Research Institute; NCI | NCT00879879 |
IFN-α lozenges | Reduce the frequency and severity of coughing | Amarillo Biosciences; Texas Tech University Health Sciences Center | NCT00690885 |
Inhaled carbon monoxide | Decrease serum level of MMP7 | Brigham and Women’s Hospital | NCTO1214187 |
Tralokinumab | Binds IL-13 | MedImmune | NCT01629667 |
Lysophosphatidic acid receptor antagonist | Antifibrotic effects in vitro and in experimental models | Bristol-Myers-Squibb | NCT01766817 |
Simtuzumab | Lysyl-oxidase-like 2 (LOXL2) inhibitor | Gilead Sciences | NCT01769196 |
SAR156597 | IL-4 and IL-13 inhibitor | Sanofi | NCT01529853 |
STX-100 | Integrin αvβ6 inhibitor | Stromedix | NCT01371305 |
Sirolimus | Reduces the number of circulating fibrocytes | University of Virginia | NCT01462006 |
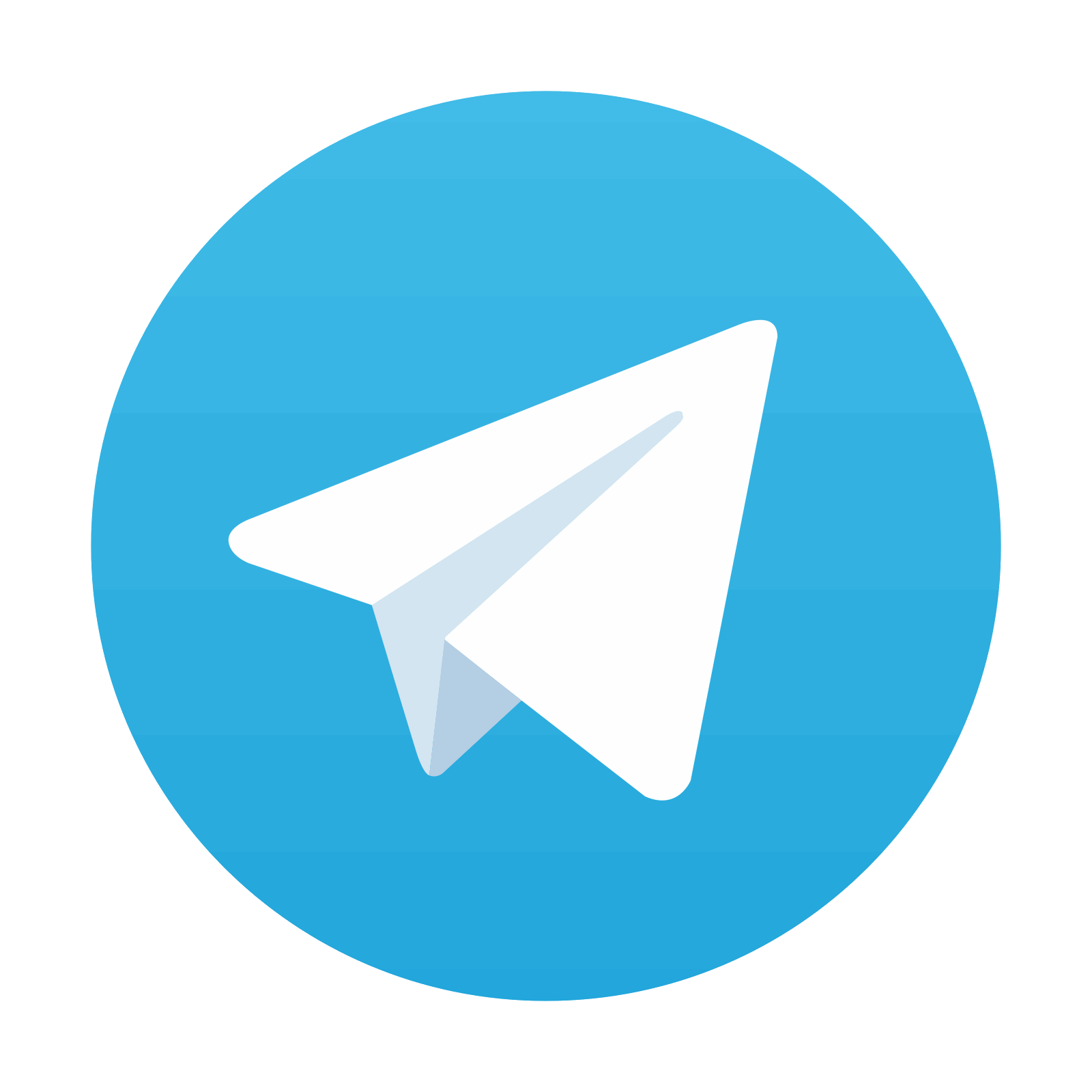
Stay updated, free articles. Join our Telegram channel

Full access? Get Clinical Tree
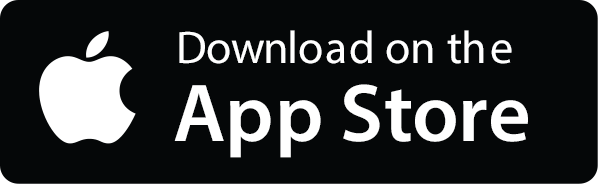
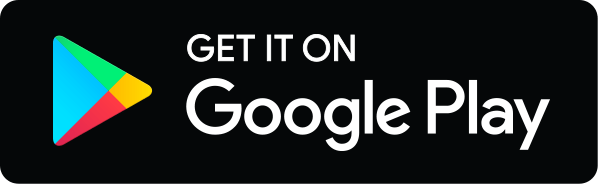
