CHAPTER 128 Hypoplastic Left Heart Syndrome
Hypoplastic left heart syndrome (HLHS) is characterized by a generalized underdevelopment of the left ventricle and the structures related to it: the mitral valve, the aortic valve, and the preductal and ductal aorta. Because HLHS constitutes a spectrum of diseases, the value of a consistent, defined nomenclature that reflects its anatomic severities has been recognized. Such a nomenclature has been proposed by the Congenital Heart Surgery Nomenclature and Database Project,1 which proposed an operative definition for hypoplastic left heart syndrome as “a spectrum of cardiac malformations, characterized by a severe underdevelopment of the left heart–aorta complex, consisting of aortic and/or mitral atresia, stenosis, or hypoplasia with marked hypoplasia or absence of the left ventricle, and hypoplasia of the ascending aorta and the aortic arch.”
EPIDEMIOLOGY
Two large epidemiologic reports have estimated the prevalence of HLHS to be 0.16 to 0.18 per 1000 live births.2,3 Males account for 57% to 67% of new cases, and the risk of sibling recurrence has been reported to be 0.5%.4,5 No environmental risk factors associated with the diagnosis of HLHS have been identified.6
CLINICAL PRESENTATION
On physical examination, these children may appear entirely normal at birth. Within hours to days, however, tachypnea and pallor may become apparent, with nonspecific chest radiography and electrocardiography findings.7,8 Depending on the adequacy of the ductal and atrial communications, there may be rapid progress to acidosis, cyanosis, and cardiopulmonary collapse. An exception to this usual sequence occurs in neonates presenting with a restrictive atrial septum; these children present immediately after birth in severe respiratory distress, respiratory acidosis, and cyanosis that is unresponsive to medical management.
Other congenital lesions should be sought. Natowicz and colleagues9 reported that 28% of patients with HLHS suffer from a genetic disorder or a major noncardiac abnormality. Looking specifically at the central nervous system among infants with HLHS, Glauser and colleagues10 documented anomalies in 29% and microcephaly in 27%. Blood flow pattern in the fetus with HLHS may have important implications for brain development. Shillingford and coworkers11 reported a correlation between microcephaly and the size of the ascending aorta. Dent and colleagues12 used magnetic resonance imaging postnatally and identified ischemic lesions in 23% of patients. Preoperative evaluation of these neonates should also include genetic and neurologic evaluations.
DIAGNOSIS
Echocardiography has become the diagnostic procedure of choice in patients with HLHS. Details such as aortic valve size and status (i.e., atretic versus patent), aortic dimensions, origin of the coronary arteries, brachiocephalic branching, status of the atrial septum, function of the tricuspid and pulmonary valves, and presence of systemic or pulmonary venous anomalies should be sought. Coronary anomalies, although rare, are more likely to be encountered in the setting of mitral stenosis and aortic atresia, and they may take the form of coronary–ventricular fistulas, hypoplasia, tortuosity, or single coronary arteries (e.g., anomalous left coronary from the pulmonary artery).12–18 Coronary abnormalities have been recognized to be more frequent in mitral stenosis–aortic atresia (MS/AA) variants of HLHS, and these patients undergo additional scrutiny, as will be discussed.
Fetal Diagnosis
Improvements in fetal echocardiography have led to an increasing frequency of antenatal diagnoses of HLHS. Tworetzky and colleagues19 reported that prenatal diagnosis was associated with improved preoperative clinical condition as well as improved survival after the Norwood operation. Unfortunately, improved survival after Norwood palliation in children with a prenatal diagnosis has not been consistently demonstrated.20 Mahle and others21 reported that although antenatal diagnosis improves preoperative clinical condition and may reduce the incidence of neurologic injury, an impact on surgical survival has not been shown.
PATHOPHYSIOLOGY
In patients with HLHS, the right ventricle must support both the systemic and the pulmonary circulation. Pulmonary venous return must have access to the right atrium, either via an atrial septal defect, a patent foramen ovale, or, rarely, via anomalous pulmonary venous connections to the systemic veins. Systemic output, delivered via the ductus arteriosus, is entirely dependent on ductal patency (Fig. 128-1). Ductal involution may be a rapid process, restricting right ventricle–dependent systemic blood flow and leading to progressive metabolic acidosis, tachypnea, and irritability before cardiopulmonary collapse.
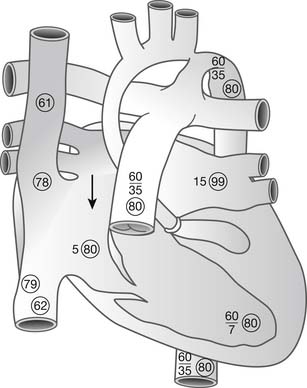
Figure 128–1 Hemodynamics and oxygen saturations (in circles) of hypoplastic left heart syndrome in the unoperated state. Pulmonary venous return must cross the atrial septum (arrow), where there is mixing with systemic venous return in the right atrium. Systemic cardiac output is dependent on a patent ductus arteriosus www.lww.com.
(Reprinted with permission from Jacobs ML. In: Kaiser LR, Kron IL, Spray TL, editors. Mastery of cardiac surgery. Philadelphia: Lippincott-Raven; 1998, p. 859.)
Finally, a few children present with severe restriction of the atrial communication. These neonates suffer from a prompt and profound hypoxemia at birth, leading rapidly to metabolic acidosis. This variant, the functional equivalent of obstructed total anomalous pulmonary venous return (TAPVR), represents a true hemodynamic emergency requiring immediate relief. As with obstructed TAPVR, medical management of this variant is uniformly unsuccessful. Interventions designed to enlarge and maintain the atrial communication have been successfully applied, such as balloon atrial septostomy and stenting.22
ETIOLOGY
HLHS can be experimentally created in the chick embryo by left atrial ligation.23 These experimental findings were anticipated clinically by Remmell-Dow and colleagues,24 who suggested that abnormal development of the atrial septum, including underdevelopment of the eustachian valve and limbus, reduced right-to-left shunting at the atrial level, resulting in hypoplasia of the left heart structures. Premature closure of the foramen ovale has likewise been implicated, as have primary abnormalities of the aortic valve.25–27
Although identifiable genetic sequences have been reported to be associated with HLHS, their relevance remains uncertain.28 An understanding of the developmental factors leading to HLHS assumes new relevance as intervention during the process of cardiac morphogenesis (i.e., fetal surgery) is pursued.
SURGICAL TRIAGE
Patients satisfying all facets of the definition of HLHS are clearly destined for the Norwood operation and single-ventricle palliation. However, for some patients, the decision between the pursuit of single-ventricle palliation and biventricular repair is not so clear. Once one course of action is pursued, changing to the competing treatment is difficult, and efforts to stratify patients with equivocal anatomy have been made. Rhodes and colleagues29 combined four factors (body surface area, indexed aortic root dimension, left ventricle length, and indexed mitral valve area) to predict death after biventricular repair for critical aortic stenosis in the neonate. However, application of these criteria to hypoplastic, but nonstenotic, left heart structures using Rhodes’ criteria has proved unsatisfying, as these criteria appear to be too stringent in this setting.30 Recognizing this, the Congenital Heart Surgeons Society (CHSS) sponsored a multi-institutional study to delineate the outcomes and risk factors of critical aortic stenosis in the neonate.31
Presented by Lofland and colleagues,31 this study identified multiple morphologic and functional factors that can be used to predict which surgical approach, single-ventricle palliation or biventricular repair, is more likely to result in the survival of any particular patient. This study determined that solution of a multivariable equation using these factors can predict patient survival. This equation, which can be found on the CHSS website (www.chssdc.org) is as follows:
The prediction model was recently tested and refined by Hickey and the CHSS.32 They reported that the consequences of inappropriate pursuit of a repair strategy (biventricular versus single ventricle), as predicted by the model, was minimal for single-ventricle palliation but was very significant among patients undergoing biventricular repair. This report underscores the importance of the initial decisions made when assessing and triaging neonates with borderline left heart structures to a treatment strategy.
MANAGEMENT
The natural history of HLHS is dismal: 95% of untreated patients die within 1 month.33 Medical treatment modifies the natural history, primarily by delaying the demise.34
The surgical management of HLHS represents the paradigm from which a generalized approach to the shunted single ventricle has evolved. Simply stated, the cardiovascular system in HLHS consists of a single pumping chamber supporting two parallel circulations. These parallel circulations can be considered to be in competition with one another for blood flow. For any given cardiac output, the flow apportioned to each circulation is inversely proportional to the resistance of that circulation (i.e., high pulmonary vascular resistance, low pulmonary blood flow). Thus, efforts to control the circulation have focused on controlling the competing vascular resistances. These efforts have generally involved manipulation of the pulmonary vascular resistance using inspired gases (oxygen, carbon dioxide, and nitrous oxide) and pressures.35–38
Pulmonary Vascular Resistance
Carbon Dioxide
Inspired CO2 has been suggested to improve the hemodynamic status after the Norwood operation.35 Tabbutt and colleagues39 compared the clinical use of hypoxia (fraction of inspired oxygen [FiO2], 17%) to CO2 in 10 neonates with HLHS prior to the Norwood operation under the conditions of fixed minute ventilation, anesthesia, and paralysis. Although both strategies reduced the ratio of pulmonary to systemic blood flow (Qp/Qs), CO2 increased both superior vena cava co-oximetry and cerebral oximetry, whereas hypoxia reduced these indices of oxygen delivery. Bradley and associates40 reported similar results among postoperative patients. These benefits, however, were realized only when the minute ventilation remained constant.
Although thought by some to reflect a direct effect, there is evidence that the increase in pulmonary vascular resistance is reversed by alkalinization, suggesting that the effect is mediated by [H+] and pH.41
Oxygen
Because the neonatal pulmonary vasculature is also sensitive to the concentration of inspired O2, hypoxic mixtures incorporating nitrogen have also been used to control the circulation in patients with HLHS. Animal models have shown that nitrogen-induced hypoxic mixtures increase the pulmonary vascular resistance and increase systemic blood flow. However, the prolonged use of hypoxic mixtures has been shown to quickly induce anatomic changes in the pulmonary vasculature. In animals, changes in arterial wall thickness and muscularity can be seen within 24 hours, and fewer intra-acinar arteries are recruited into the circulation.42–44
Day and colleagues45 reported their clinical experience using hypoxia to manipulate the pulmonary vascular resistance in 20 neonates with single-ventricle, duct-dependent circulation awaiting heart transplant. Eight patients survived; of the 10 patients who underwent lung biopsy or autopsy, nine showed medial hypertrophy in distal arterioles, and seven of these nine patients died. Although the outcome cannot be completely ascribed to hypoxic management, this strategy does suggest that prolonged supportive care of the neonatal single ventricle may expose these patients to significant risks.
Systemic Vascular Resistance
Manipulation of the systemic vascular resistance is a demonstrated means of controlling the shunted circulation. In fact, our ability to pharmacologically control the systemic vascular resistance probably exceeds our current ability to selectively manage the pulmonary vascular resistance. Clinically, this approach usually employs an irreversible α-adrenergic antagonist, phenoxybenzamine, to achieve systemic vasodilation. The desired systemic vascular resistance, defined as that which optimizes the Qp/Qs and systemic oxygen delivery, is then obtained by titrating an α-adrenergic agonist, usually norepinephrine. Specifics of this approach have been reported in the literature.43–45
A recent review of this strategy identified the use of phenoxybenzamine, continuous systemic venous oxygen monitoring, and the reduction of time in deep hypothermic circulatory arrest (DHCA) as factors favoring survival to the bidirectional Glenn operation.46 However, no studies directly comparing outcomes for these two fundamentally different management schemes have been performed.
STAGE I
Standard Norwood Operation for Hypoplastic Left Heart Syndrome
The standard Norwood operation is performed through a median sternotomy. The branch pulmonary arteries are dissected and controlled. We routinely anastomose a 3.5-mm stretch Gore-Tex graft to the underside of the innominate artery. This graft, later to serve as the right modified Blalock-Taussig shunt, is left long and is used as the primary arterial cannulation site. Venous cannulation is through the right atrial appendage, through a pursestring suture large enough to allow subsequent atrial septectomy. With the patient cooled and on bypass, the ductus is divided, and the brachiocephalic vessels are mobilized and loosely snared. The main pulmonary artery is divided and the branch bifurcation is closed, primarily or with a patch. After at least 20 minutes of cooling and at a rectal temperature of 18° C, the brachiocephalic vessels are snared and the descending aorta is clamped. For truly diminutive aortas (<2 mm), cardioplegia is administered via a side arm into the arterial cannula, and direct needle insufflation is used in the larger aorta. We perform the Norwood operation during continuous regional low-flow perfusion, as described elsewhere.47–49
The atrial septectomy is performed through the right atrial pursestring suture during a period of low-flow sucker bypass. The ductal remnant is amputated from the aorta, and the aorta is filleted open distally beyond the site of coarctation, and proximally to a point adjacent to the lip of the transected pulmonary valve. The ductal insertion site is circumferentially resected, and the back wall of the aorta is sewn together, either directly or with an interdigitating technique. Proximally, a side-to-side anastomosis between the aorta and pulmonary valve is performed, while the arch is augmented using a piece of pulmonary homograft or fixed autologous pericardium (Fig. 128-2). Central bypass is resumed after cannulation of the reconstructed aorta, and the pulmonary anastomosis of the shunt is completed.
Results of Norwood Operation
With experience and with advances in surgical, anesthetic, and critical care management, survival after the Norwood operation now approaches 90% at specialized centers.50,51 In one of the largest single-center series reported, Mahle and colleagues52 reported their 15-year (1984 to 1999) experience with the Norwood operation for 840 patients with HLHS. The sex ratio (65% male and 35% female) was similar to that reported elsewhere. The 1-, 2-, 5-, 10-, and 15-year survival rates for the group were 51%, 43%, 40%, 39%, and 39%, respectively. Risk factors identified for death were earlier era of operation, age greater than 14 days, and weight less than 2.5 kg. Neither anatomic subtype nor heterotaxy was associated with mortality.
Because the surgical and medical management techniques for HLHS continue to evolve, contemporary experiences are important. Tweddell and colleagues51 recently reported their experience with 115 consecutive patients with HLHS undergoing the Norwood operation between 1992 and 2001. They reported that, with the introduction of new management techniques in 1996, they were able to obtain 93% hospital survival for neonates undergoing the Norwood operation for HLHS. These strategies included the use of phenoxybenzamine, continuous systemic venous oxygen monitoring, aprotinin, modified ultrafiltration, and, most recently, continuous cerebral perfusion. This report is also of interest because it attempts to quantify the influence of anatomic and operative variables on survival to stage II palliation, rather than on operative survival. Only duration of DHCA was associated with survival to stage II palliation; no other anatomic or physiologic variable, including weight, was predictive.
A more recent review of the surgical experience of 237 patients undergoing stage I palliation at Children’s Hospital Boston between 2001 and 2006 reported a hospital survival of 88.6%.50 An arteriopulmonary shunt was used in 157 patients, and a right-ventricle-to-pulmonary-artery (RV-PA) conduit in 80. Although the source of pulmonary blood flow did not affect hospital survival, the RV-PA conduit imparted a significant survival advantage during the interstage period (Fig. 128-3).
Anatomic Subtypes
Although previous studies16,17 reported a trend toward higher mortality among patients with aortic valve atresia, the Children’s Hospital Boston review50 clearly identified patients with aortic atresia and mitral stenosis to be at higher risk than those with other anatomic subtypes (Fig. 128-4).Thirty-eight of 165 patients (23%) with HLHS had MS/AA. Hospital mortality and need for transplant for patients with MS/AA was significantly higher than for other anatomic subgroups (29% versus 7.8%, P = .006; Fig. 128-5). Recently, we identified the presence of significant left-ventricle-to-epicardial coronary artery fistula in these patients, and, in one case, autopsy-proven aortocoronary atresia. A retrospective review of these patients identified echocardiographic evidence of coronary artery fistulas in 20 patients (53%) with MS/AA. The presence of suggestive echocardiographic findings was associated with a significantly higher hospital mortality (50% versus 6%, P = .04; Fig. 128-6). It is in this cohort of patients that the increased risk seems to be isolated, as patients with MS/AA without evidence of fistulas demonstrated survival rates similar (94%) to those with other anatomic subtypes (aortic atresia–mitral atresia [AA/MA], 91%, and aortic stenosis–mitral stenosis [AS/MS], 93%; P = .9). Although these findings were originally interpreted as small muscular ventricular septal defects, cardiac catheterization has clearly identified left-ventricle-to-coronary-artery fistulas in these patients (Fig. 128-7). No advantage to the arteriopulmonary shunt or the Sano modification has been identified for these patients.
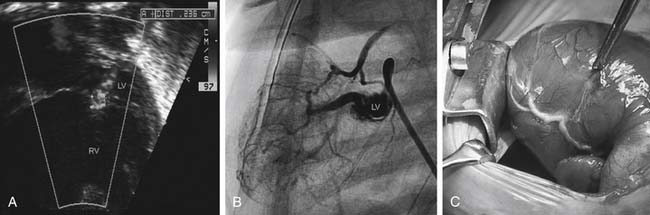
Figure 128–7 Diagnosis of left-ventricle-to-coronary-artery fistulas. A, Echocardiographic examinations often reveal flow “across” the interventricular septum and have been considered to represent ventricular septal defects. B, Angiographic demonstration of left-ventricle-to-coronary-artery fistulas with retrograde filling of the ascending aorta in addition to the right coronary artery system. C, Intraoperative examination has consistently revealed a small “duvet” or “pucker” in the myocardium, presumably at the site of the fistulas, with a thickened and corkscrew appearance of the epicardial coronary artery www.lww.com.
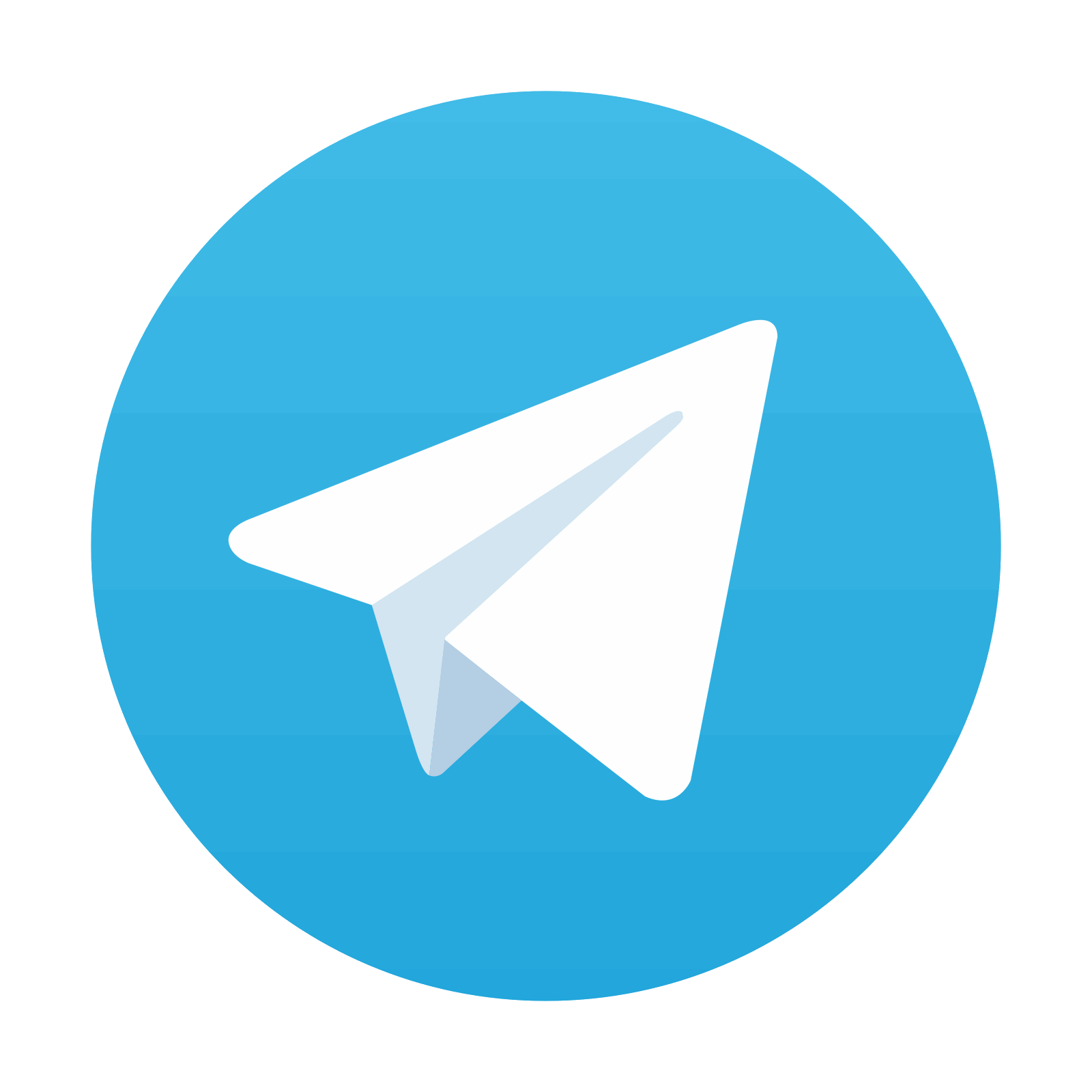
Stay updated, free articles. Join our Telegram channel

Full access? Get Clinical Tree
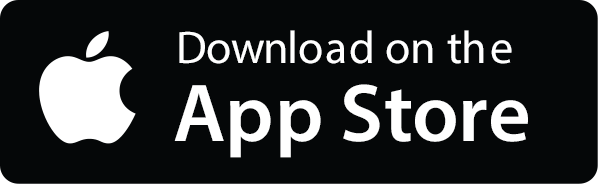
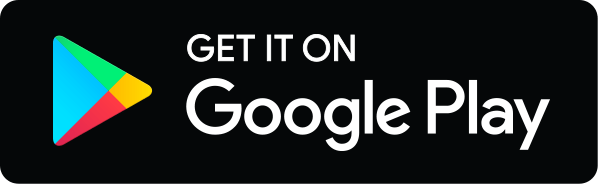