How to Obtain a Good Echocardiography Examination: Ultrasound Physics, Technique, and Medical Knowledge
The burgeoning technologic revolution of the past two decades has produced a continuous evolution in the definition of a complete and comprehensive echocardiographic evaluation (Fig. 1-1). Echocardiography is now a fully grown tree. It has numerous clinical applications, with various forms of ultrasound technology being used throughout the entire field of cardiovascular medicine. This mature ultrasound tree has grown from a seed planted more than 50 years ago. Since then, the tree has been trimmed and nourished carefully by many pioneers to serve the needs of patients and clinicians.
In 1954, Edler and Hertz (1) of Sweden were the first to record movements of cardiac structures, in particular, the mitral valve, with ultrasound. In the early 1960s in the United States, Joyner and Reid (2) at the University of Pennsylvania were the first to use ultrasound to examine the heart. Shortly afterward, in 1965, Feigenbaum and colleagues (3) at Indiana University reported the first detection of pericardial effusion with ultrasound and were responsible for introducing echocardiography into the clinical practice of cardiology. However, M-mode echocardiography produced only an “ice pick” view of the heart; two-dimensional (2D) sector scanning, developed in the mid-1970s, allowed real-time tomographic images of cardiac morphology and function (4). The first phased array 2D sector scan at Mayo Clinic was made on March 17, 1977. Although the development of Doppler echocardiography paralleled that of M-mode and 2D echocardiography from the early 1950s, it was not used clinically until the late 1970s. Pressure gradients across a fixed orifice could be obtained reliably with blood-flow velocities recorded by Doppler echocardiography. Two groups, Holen and colleagues (5) and Hatle and colleagues (6, should be credited for introducing Doppler echocardiography into clinical practice.
Numerous validation studies subsequently confirmed the accuracy of Doppler echocardiography in the assessment of cardiac pressures. Therefore, the Doppler technique made echocardiography not only an imaging but also a hemodynamic technique. On the basis of the Doppler concept, color flow imaging was developed in the early 1980s so that blood flow could also be visualized noninvasively (7).
Another ingenious modification of Doppler echocardiography was tissue Doppler imaging (TDI), which allows echocardiographers to record myocardial tissue velocity and to measure the extent of myocardial deformation as strain (8, 9). These measurements provide a sensitive assessment of systolic and diastolic function and are becoming a standard component of a comprehensive echocardiography examination. Widespread clinical use of transesophageal echocardiography (TEE) began in 1987 (10, and the subsequent development of intravascular and intracardiac high-frequency transducers has permitted extraordinarily detailed imaging and hemodynamic assessment of the cardiovascular system. Most recently, three-dimensional (3D) echocardiography has become a reality. It provides a more realistic depiction of cardiovascular structures and more accurate volumetric quantitation (11, 12).
Another ingenious modification of Doppler echocardiography was tissue Doppler imaging (TDI), which allows echocardiographers to record myocardial tissue velocity and to measure the extent of myocardial deformation as strain (8, 9). These measurements provide a sensitive assessment of systolic and diastolic function and are becoming a standard component of a comprehensive echocardiography examination. Widespread clinical use of transesophageal echocardiography (TEE) began in 1987 (10, and the subsequent development of intravascular and intracardiac high-frequency transducers has permitted extraordinarily detailed imaging and hemodynamic assessment of the cardiovascular system. Most recently, three-dimensional (3D) echocardiography has become a reality. It provides a more realistic depiction of cardiovascular structures and more accurate volumetric quantitation (11, 12).
With these technologic advances, the application of echocardiography has been spreading into numerous clinical areas, including the evaluation of diastolic function, stress echocardiography, intraoperative echocardiography, fetal echocardiography, contrast echocardiography, intracardiac imaging, and vascular imaging. The size of the ultrasound unit is becoming smaller, and some units can be hand-carried to the patient’s bedside (13, 14). We are fortunate to have this versatile diagnostic modality to provide reliable structural, functional, and hemodynamic information about the cardiovascular system of our patients.
Ultrasound and Transducer
Echocardiography uses ultrasound to create real-time images of the cardiovascular system in action. Ultrasound represents sound waves with a frequency of 20,000 Hz or more. All sound waves (Fig. 1-2) are characterized by the following seven variables (15: frequency (f), wavelength (λ), period (p), speed (sp), amplitude (A), power, and intensity.
f = the number of cycles per second; 1 cps is 1 Hz.
λ = the length of one complete cycle of the sound; its usual unit of measure is millimeters (mm).
sp = the speed or velocity of sound waves through a medium is equal to the product of f and λ (sp = f • λ) and is determined by the characteristics of the medium. Speed is not affected by the frequency of sound. The average speed of sound in soft tissue is 1,540 m/s.
p = the time duration of 1 cycle; hence, 1 second/f = p or f • p = 1.
A = the magnitude of a sound wave, the maximum change from the baseline.
Power is the rate at which energy is transferred from a sound beam, in watts (W), and is proportional to the amplitude squared (15).
Intensity is the concentration of energy in a sound beam and equals power divided by its cross-sectional area.
Sound waves can be combined to create one wave. Thus, two in-phase (or superimposed) waves create a wave with a larger amplitude, and two out-of-phase (or mirror-image) waves create a wave with a smaller amplitude or the two waves cancel each other if they have the same amplitude. This phenomenon is called interference (15). It is used in pulse-inversion and pulse-modulation techniques for harmonic imaging and contrast echocardiography.
At the start of an echocardiography examination, the appropriate transducer is selected according to the type of examination and patient’s body habitus. A higher frequency transducer provides better resolution, but it has a shallower depth of penetration. For the pediatric population, the transducer frequency is usually 5 to 7.5 MHz (1 MHz = 1 million cps), but for adults the transducer frequency at the start of an examination is usually 2 to 2.5 MHz and occasionally 5 MHz for patients with a thin chest wall.
The transducer consists of piezoelectric elements that convert electrical energy to ultrasound and vice versa. Electrical energy is applied to the transducer in pulses with a defined pulse repetition frequency (PRF in kilohertz [kHz]), producing ultrasound waves at defined, regular intervals of pulsed repetition period. The wavelength of the ultrasound generated is related to the thickness of the piezoelectric elements. The thinner the elements, the shorter the wavelength. Because the product of wavelength
(λ) and sound frequency (f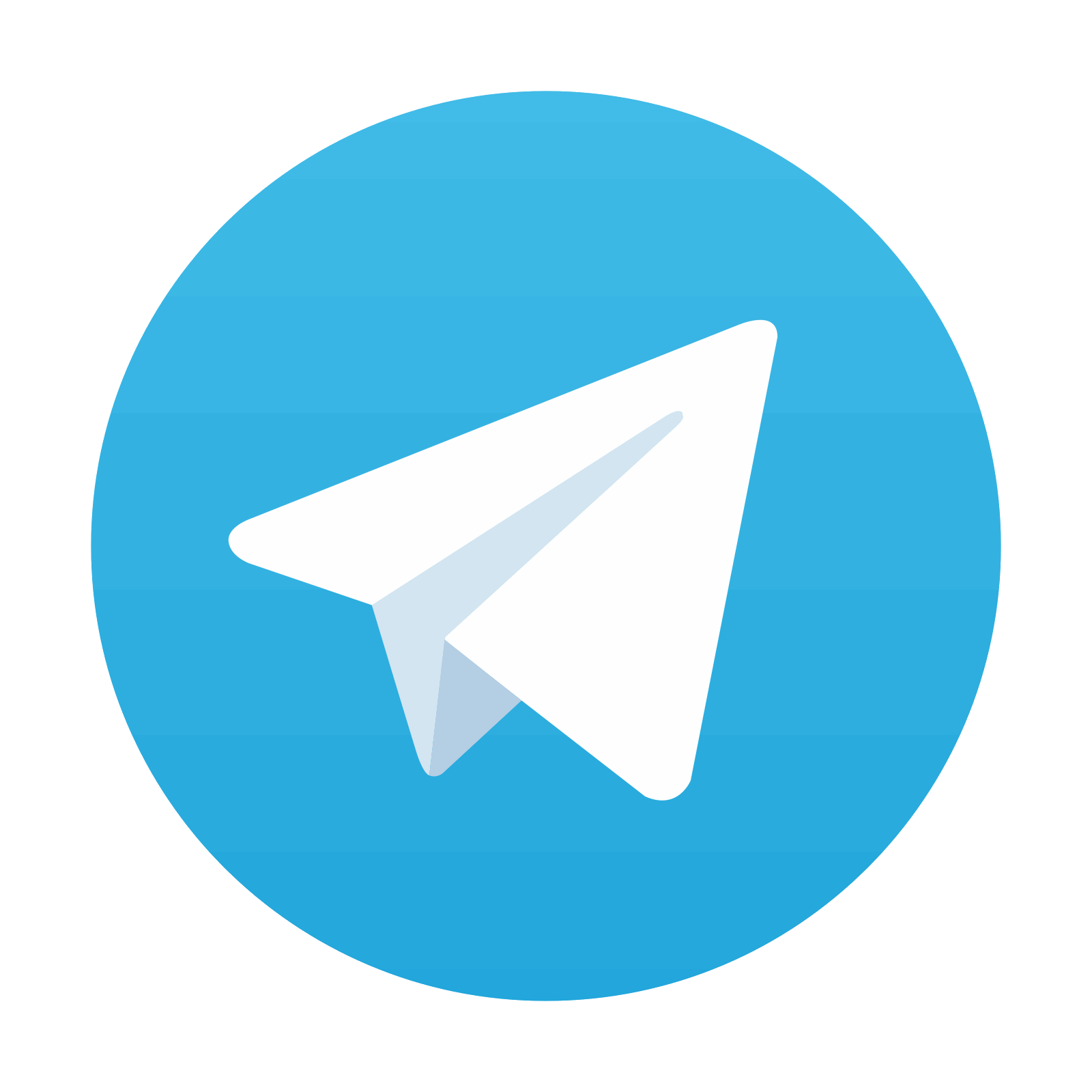
(λ) and sound frequency (f
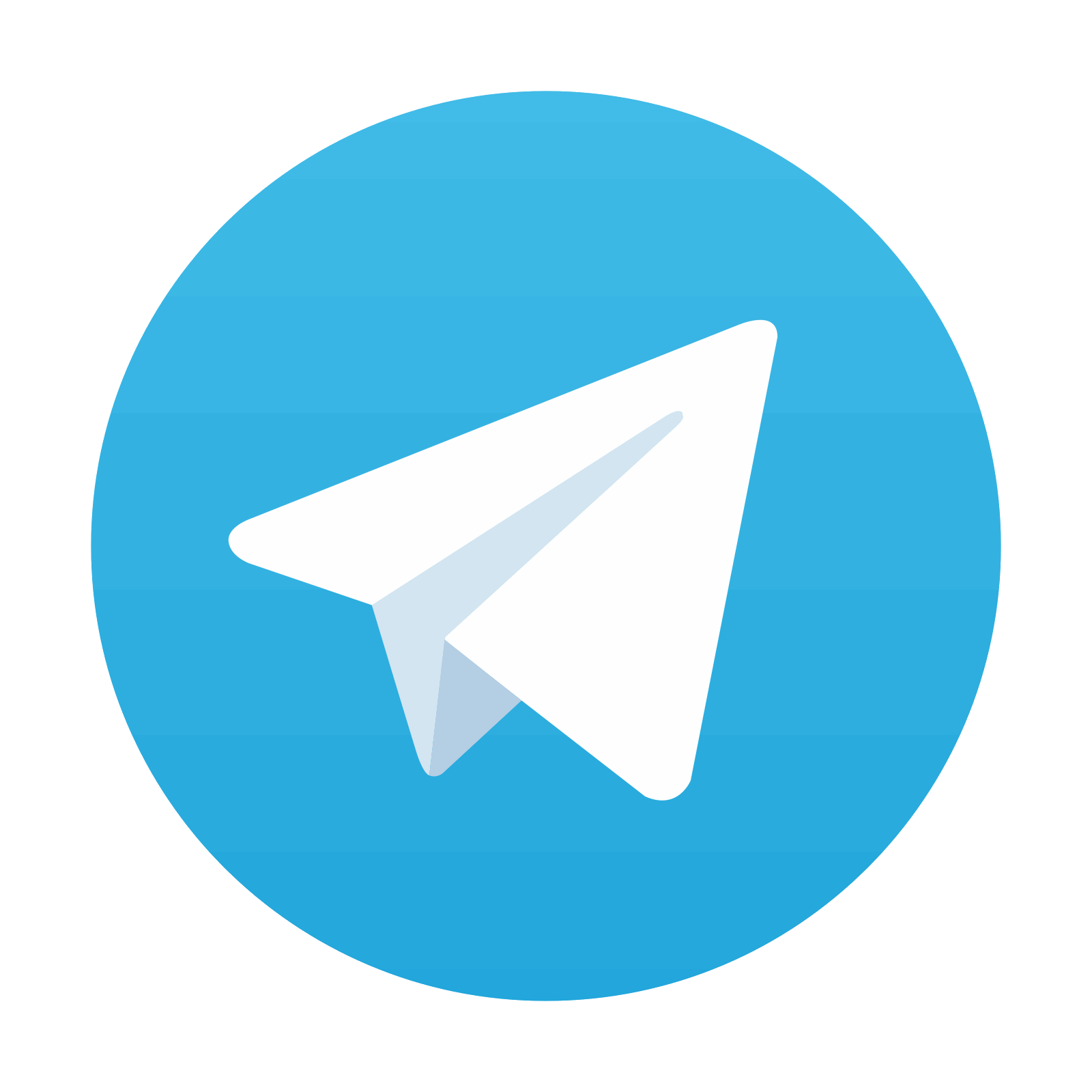
Stay updated, free articles. Join our Telegram channel

Full access? Get Clinical Tree
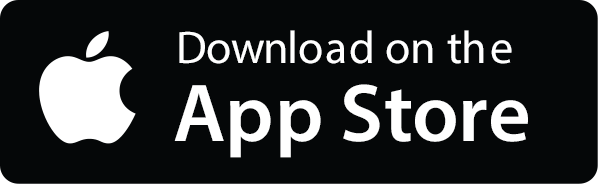
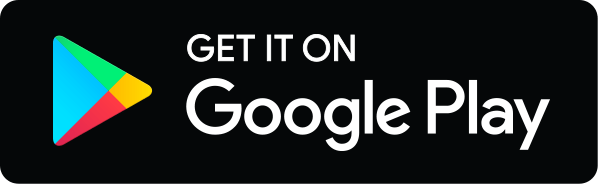