Fig. 34.1
Apical view of the four heart valves—aortic, mitral, pulmonic, and tricuspid
34.2 A New Frontier: Valve Replacement
Before 1950, the ability to safely and effectively operate on the human heart was considered an insurmountable goal. Attempts to operate to correct valvular diseases without stopping the heart resulted in severe, often fatal complications including uncontrollable bleeding and the introduction of air emboli [1]. The ability to maintain forward flow of blood while stopping the heart to allow the surgeon access to the valve would have to wait for the development of cross-circulation, and later for the perfection of the cardiopulmonary bypass procedure by Dr. C. Walton Lillehei, Richard L. Varco, and Dr. F. John Lewis at the University of Minnesota [2] (see also Chap. 25). With this new technology, a new frontier in surgical options for the treatment of heart valve disease began to emerge. During the past several years, major advances have occurred in diagnostic techniques (i.e., imaging) and therapeutic interventions for valvular diseases, as well as improved understanding of the natural history of both treated and untreated valvular disease (for more detail, see Chaps. 35 and 36).
34.2.1 Mechanical Prosthetic Valves
By 1961, Dr. Albert Starr and Lowell Edwards had successfully implanted the world’s first mechanical valve into a human to replace a mitral valve that had been deformed by rheumatic fever [3]. Initially, this steel ball and cage design was successful in approximately 50 % of implantations. Major complications were soon recognized, including: (1) clot formation resulting in embolic strokes; (2) significant noise; (3) red blood cell destruction; and/or (4) tissue in-growth causing subsequent valve obstruction. A complete history of the development of currently used mechanical prostheses is beyond the scope of this text. However, it is important to mention two key aspects of any successful new valve design: (1) improved valve hemodynamics; and (2) reduced thrombogenic (or clot forming) potential. Efforts to optimize valve hemodynamic function date back to the development of the Lillehei/Kaster tilting disk valve which allowed blood to flow centrally through the valve. At that time, this new type of valve emphasized the requirement to design a valve that would reduce turbulent blood flow, reduce cell destruction, and minimize the transvalvular gradients [4]. A transvalvular gradient is defined as the pressure difference across the valve. Despite the advantages of a new steel tilting disk design, careful strict anticoagulation therapy was still required to reduce the risk of clot formation [5]. The next improvements of these valves came with the development of the pyrolytic carbon valve leaflets. The nonthrombogenic weight and strength properties were determined by Drs. Jack Bokros and Vincent Gott. Subsequently, pyrolytic carbon was used in the creation of a bileaflet valve inspired by Dr. Kalke. This valve, originally manufactured by St. Jude Medical (St. Paul, MN, USA), provided exceptional performance, and today this design remains the gold standard for mechanical valves [6]. To date, all patients with mechanical valves require anticoagulation, e.g., with oral warfarin therapy which reduces the risk of thromboembolism to 1–2 %/year (Table 34.1) [7]. It should be noted that numerous studies have demonstrated that the risk of thromboembolism is directly related to the valve implant position, i.e., in the descending order of risk, the tricuspid, mitral, and aortic valves. In addition, this risk of emboli appears to be greatest in the early post-implant period, and then becomes reduced as the valve sewing cuff becomes fully endothelialized.
Warfarin INR 2.5 | Warfarin INR 3.0 | Aspirin 75–100 mg | ||
---|---|---|---|---|
Mechanical prosthesis | ||||
First 3 months post implantation | + | + | ||
After initial 3 months | Aortic valve | + | ||
Aortic valve + Risk factor | + | + | ||
Mitral valve | + | + | ||
Mitral valve + Risk factor | + | + | ||
Biological prosthesis | ||||
First 3 months post implantation | + | + | ||
After initial 3 months | Aortic valve | + | ||
Aortic valve + Risk factor | + | + | ||
Mitral valve | + | |||
Mitral valve + Risk factor | + | + |
In general, management of anticoagulation must be individualized to the patient to minimize risk of thromboembolism and, at the same time, prevent bleeding complications. In situations where a patient with a valve prosthesis requires noncardiac surgery, warfarin therapy should be stopped only for procedures where risk of bleeding is substantial. A complete discussion of anticoagulation therapy is beyond the scope of this chapter, however several excellent reviews are available on this subject [7, 8].
34.2.2 Biological Prosthetic Valves
Because of the problems related to anticoagulation, a majority of subsequent valve research focused on developing tissue alternatives that avoid the need for anticoagulation. From a historical perspective, Drs. Lower and Shumway performed the first pulmonary valve autotransplant in an animal model [9]. Later in 1967, Dr. Donald Ross completed the first successful replacement in a human. The Ross Procedure is a well-established method still used today to replace a diseased aortic valve with the patient’s own pulmonary valve (Fig. 34.2); a donor tissue valve or homograft (Table 34.2) is then used as a prosthetic pulmonary valve. In general, tissue valves are significantly more biocompatible than their mechanical counterparts. These valves are naturally less thrombogenic, and thus the patient does not require aggressive anticoagulation. Specifically, a risk of <0.7 %/year of clinical thromboembolism has been reported in valve replacement patients eliciting sinus rhythm without warfarin therapy [7]. Therefore, this treatment option is advantageous in clinical situations where the use of anticoagulation would significantly increase morbidity and mortality. Yet, to date, a potential major disadvantage of tissue valve implantation is early valvular degeneration as a result of leaflet calcification. Thus, methods for tissue preservation to prevent such calcifications are currently a major focus of research in this field.
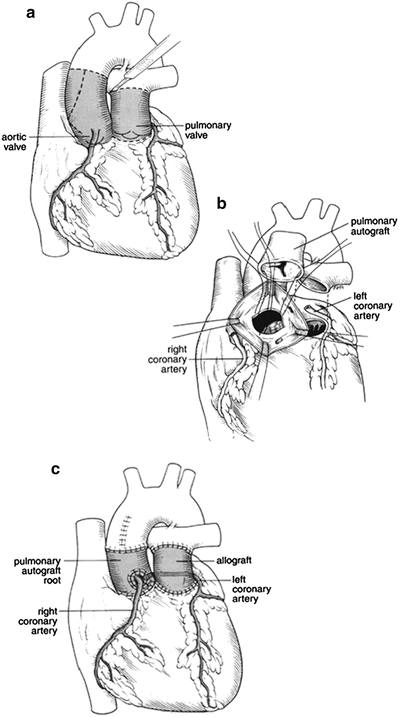
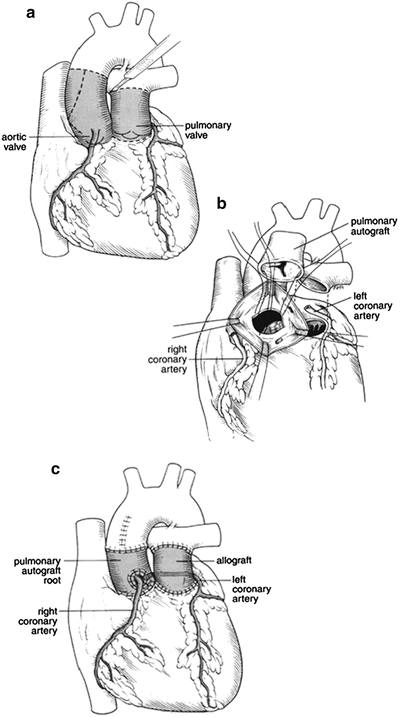
Fig. 34.2
Schematic drawing of the Ross Procedure. (a) Resection of the diseased aortic valve. (b) Harvesting of native pulmonary valve. (c) Implantation of the pulmonic valve in the aortic position and reimplantation of coronary arteries
Table 34.2
Tissue valve graft options: classification of bioprosthetic valves
Bioprosthetic valve | Description |
---|---|
Stented porcine valve (Xenograft) | A three leaflet valve supported by three artificial struts or stents to maintain leaflet structure and geometry. |
Stentless porcine valve (Xenograft) | A length of porcine aorta including tissue below (proximal) and above (distal) to the valve, called the “root.” |
Bovine pericardial valve (Xenograft) | A three leaflet valve created from bovine pericardium attached to a stented frame. |
Homograft | A human aortic valve and root. |
Autograft | A pulmonary valve and root excised from the patient and reimplanted in the same patient. |
34.2.3 Biological Versus Mechanical Valves
The choice of a mechanical or biologic valve for implant will typically depend on various factors: (1) the patient’s current disease status; (2) the specific native valve involved; and/or (3) the surgeon’s preference and experience. If these factors are not limiting, the choice of valve type should be based on the maximization of benefits over risks for the individual patient. Unfortunately, the ideal prosthetic valve that combines excellent hemodynamic performance and long-term durability without increased thromboembolic risk or the need for lifelong anticoagulation remains elusive. In general, mechanical valves offer greater durability at the cost of requiring lifelong anticoagulation, as well as the risk of thromboembolism. In contrast, bioprosthetic valves have a much lower thromboembolic risk without the need for anticoagulation, but elicit a higher risk for structural degeneration and thus potential need for reoperation. As such, mechanical valves are perhaps most well suited for the younger patient who does not desire future reoperations. Currently, mechanical valve replacement in the USA is quite standardized and commonplace, i.e., yielding satisfactory valve function that is reproducible from patient to patient. Furthermore, the flow gradients with newer bileaflet mechanical valves have dramatically improved from the early ball valve type; currently, a trileaflet valve is in the preclinical stages of development and may eventually not require anticoagulation therapy. Nevertheless in the interim, bioprosthetic or tissue valves offer a safe alternative for patients in whom the risk of anticoagulation is prohibitively high (e.g., elderly patients >70 years of age, women of child bearing years desiring pregnancy). Yet, the length of their durability remains a serious concern for tissue valves, and thus a patient whose life expectancy is greater than that of the prosthesis will likely encounter the risk of another surgery for a second valve replacement. Note that a transcatheter-delivered valve in valve procedure is a more recent option (Chap. 36).
It is important to note that two historic randomized clinical trials have compared outcomes between early generation tissue and mechanical valves—the Edinburgh Heart Valve trial and the Veteran Affairs Cooperative Study on Valvular Heart Disease [9–11]. Both trials showed increased bleeding associated with mechanical valves and increased reoperations with tissue valves. While the strength of these trials is a prospective randomized design, the disadvantages are that the valves used in these trials are currently obsolete. More recently, a large meta-analysis comparing mechanical versus bioprosthetic aortic valves found no difference in risk-corrected mortality regardless of patient age [12]. Based on this and other studies, the choice of valve should not be based on age alone. Clearly, there is a trend towards increasing use of bioprosthetic valves in younger patients; this is based on the fact that advances in tissue fixation and improved anti-calcification treatments have resulted in superior durability of the newer generation bioprosthetic valves. Specifically, third generation bioprosthetic valves have been shown to have a greater than 90 % freedom from structural generation at 12-year follow-up [13]. Furthermore, improvements in cardiac surgery including better techniques for myocardial preservation, less invasive procedures (i.e., robotic surgery), as well as strategies for cardiac reoperation have significantly reduced the risk for cardiac reoperation. This has further allowed an increasing application of bioprosthetic valves in patients younger than 55–60 years old. In conclusion, in the absence of current randomized trials, physicians must make a choice based on existing data and individualize that choice based on patient-related factors such as age, lifestyle, tolerance for anticoagulation, and/or position of the replacement valve [14].
34.2.4 Prosthetic Heart Valve Endocarditis and Performance Tracking
All patients with prosthetic valves also need appropriate antibiotics for prophylaxis against infective endocarditis. Details of these therapies are beyond the scope of this chapter, but the reader is referred to guidelines published by a joint committee from the American Heart Association (AHA) and American College of Cardiology (ACC) for the applicable protocols. In addition, a registry has been established to track the long-term performance of all clinically approved implanted valve prostheses. Established standards were revised in 1996 and are briefly summarized in Table 34.3. As alluded to in Chap. 27, investigators seeking approval for all new valves must also report any complications that occur in the preclinical animal testing phase to the appropriate regulatory authority.
Complication | Description |
---|---|
Structural valvular deterioration | Any change in function of an operated valve resulting from an intrinsic abnormality, causing stenosis or regurgitation. |
Nonstructural dysfunction | Any stenosis or regurgitation of the operated valve that is not intrinsic to the valve itself, including inappropriate sizing, but excluding thrombosis and infection. |
Valve thrombosis | Any thrombus, in the absence of infection, attached to or near an operated valve that occludes part of the blood flow path or interferes with function of the valve. |
Embolism | Any embolic event that occurs in the absence of infection after the immediate perioperative period (new temporary or permanent, focal or global neurological deficit, and peripheral embolic event). |
Bleeding event (anticoagulant hemorrhage) | Any episode of major internal or external bleeding that causes death, hospitalization, permanent injury, or requires transfusion. |
Operated valvular endocarditis | Any infection involving an operated valve, resulting in valve thrombosis, thrombotic embolus, bleeding event, or paravalvular leak. |
34.3 Specific Valvular Diseases: Etiologies and Treatments
The remainder of this chapter is devoted to a generalized summary of the most common valvular diseases affecting patients in the Western world. Of the four heart valves, significant clinical disease can primarily affect all but the pulmonary valve. Yet, compromised function of this valve is noted to occur in the adult congenital heart patient who previously underwent reparative surgeries. Indications for diagnostic, therapeutic, and follow-up intervention will be discussed for each disease. Note that a complete evidence-based summary of recommendations for intervention and level of physical activity for individuals with valvular disease is available from several excellent reviews [8, 15, 16].
34.3.1 Aortic Valve Disease
Anatomically, the normal aortic valve is composed of the annulus and the left, right, and noncoronary leaflets (sometimes referred to as cusps) (Fig. 34.3). Diseases affecting these structures can be subdivided into aortic stenosis or regurgitation, or some combination thereof. Overall, aortic stenosis is considered a surgical disease with aortic valve replacement considered to be the standard of care. Treatment of aortic regurgitation is also typically surgical, though the exact method chosen will vary widely based on the etiology of the disease.
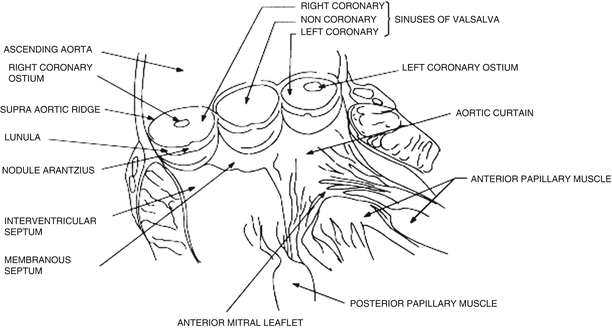
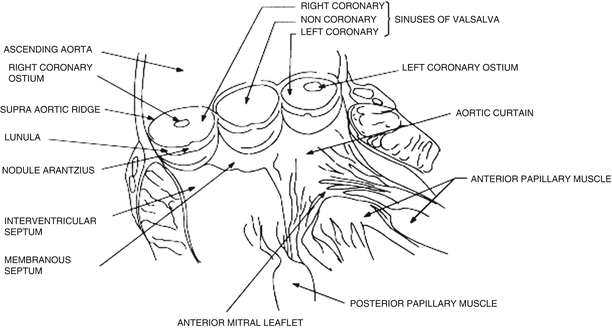
Fig. 34.3
Anatomy of the aortic valve. Adapted from Duran CMG (1994) Conservative valve surgery. In: Zaibag MA, Duran CMG (eds) Valvular heart disease. Marcel Dekker, New York, p 584
34.3.1.1 Aortic Stenosis
Aortic stenosis causes varying degrees of left ventricular outflow tract obstruction [17, 18]. The various etiologies of aortic stenosis are subdivided into acquired versus congenital. Regardless of the etiology, the most common two causes of aortic stenosis in adults are calcification of a normal trileaflet or a congenital bicuspid aortic valve. Interestingly, among individuals under the age of 70, bicuspid aortic valve disease is the most common cause of aortic stenosis. These congenitally abnormal valves typically develop progressive fibrosis and calcification of the leaflets over several decades, and can present for surgery at any time during an individual’s life, i.e., depending on the degree of deformity and rate of progression of the narrowing. Patients over the age of 70 more typically elicit the so-called senile aortic stenosis; these valves start out as normal valves, but develop thickening, calcification, and stenosis with aging. In a patient with any degree of aortic stenosis, careful clinical follow-up is mandatory to follow the progression of stenosis, and typically surgery is indicated at the onset of any symptoms (see below). Congenital malformation (typically presenting in bicuspid aortic valves) results in progressive fibrosis and calcification of the leaflets over several decades. The average rates of reduction in valve orifice area have been estimated to be ~0.12 cm2/year, and valve orifice areas are typically used to grade the relative severity of valve stenosis (Table 34.4) [19]. Nevertheless, progression of aortic stenosis varies significantly and the appearance of symptoms may not correlate well with the given measured valve area. Therefore, careful clinical follow-up is mandatory, as it is difficult to predict an actual individual rate of stenotic progression. In general, aortic stenosis is graded into various categories of severity based on degrees of mean pressure gradient, aortic jet velocity, and/or valve area.
Valve orifice area (cm2) | Peak aortic velocity (m/s) | |
---|---|---|
Mild | >1.5 | <3.0 |
Moderate | >1.0 to 1.5 | 3.0–4.0 |
Severe | <1.0 | >4.0 |
Valve stenosis may also be associated with progressive outflow tract obstruction, which can then cause additional increases in left ventricular pressure. As a result, concentric left ventricular hypertrophy is an early response, which assists initially in maintaining normal left ventricular systolic wall tension and ejection fraction [20]. However, once this response becomes functionally inadequate, afterload tends to increase which, in turn, results in a gradual reduction in overall ejection fraction (Fig. 34.4). In some patients, an initial ventricular hypertrophy itself may also be detrimental, producing subendocardial ischemia even in the absence of coronary artery disease [21, 22]. As such, this results in further systolic and diastolic left ventricular dysfunction and may predispose such patients to a potentially larger degree of myocardial ischemia and higher mortality [7, 8, 17, 18, 23].
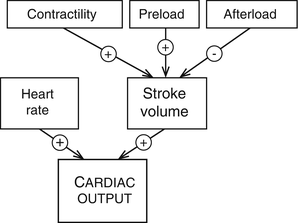
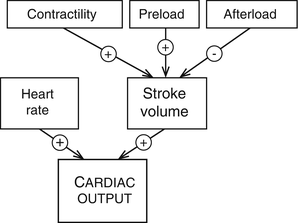
Fig. 34.4
Determinants of cardiac output include contributions from preload and afterload pressures, contractility, and heart rate. Adapted from Lilly LS (ed) (1993) Pathophysiology of heart disease. Lea & Febiger, Philadelphia, p 149
Although aortic stenosis may not produce symptoms early in its clinical course, in time symptoms of angina, syncope, heart failure, and/or even sudden death will develop. Although the latter are the classic symptoms of aortic stenosis, more subtle symptoms such as reduced effort tolerance, fatigue, and exertional dyspnea can also occur. Once symptoms are present, average survival without intervention is less than 2–3 years [17, 18, 24–29]. Furthermore, the mortality of patients with aortic stenosis, in the absence of surgical treatment, present with: (1) angina, 50 % within 5 years; (2) syncope, 50 % mortality within 3 years; and (3) heart failure, 50 % mortality within 2 years. Therefore, a high degree of skepticism is necessary to make the diagnosis prior to the onset of symptoms to maximize a given patient’s outcome. In general, aortic stenosis can be detected early based on: (1) the presence of a systolic outflow murmur; (2) the occurrence of delayed/diminished carotid upstrokes; (3) a sustained left ventricular impulse; (4) a reduced intensity of the aortic component of the second heart sound; and/or (5) evidence of left ventricular hypertrophy on exam, chest X-ray, and/or EKG. Typically, results of echocardiography can be further used to confirm the diagnosis of aortic stenosis and also provide for the detailed assessment of: (1) mean transvalvular pressure gradient; (2) derived valve area; (3) relative left ventricle size (degree of hypertrophy) and function; and/or (4) presence of other associated valvular disease. For more details on the clinical use of echocardiography, the reader is referred to Chap. 22. It should also be noted that advances in magnetic resonance imaging may be applied in diagnosing such patients (Chap. 24).
Physicians who follow patients with known aortic stenosis commonly perform an annual history and physical examination, and urge these patients to promptly self-report the development of any new symptoms. Although changes in valve area alone are not totally predictive, annual echocardiography is also useful to assess progression of ventricular hypertrophy and alterations in function. In any case, the development of any new symptoms (e.g., exertional chest discomfort, shortness of breath, or fainting spells) warrants additional clinical assessment, given that aortic stenosis progresses rapidly once such symptoms are present.
In patients being considered for aortic valve replacement secondary to aortic stenosis, cardiac catheterization is generally indicated in individuals >40 years of age to assess for any degree of significant coronary artery disease. Additional indications include the assessment of: (1) hemodynamic severity of the aortic stenosis in situations where there is a discrepancy between clinical and echocardiographic findings; and (2) situations where there is evidence of pulmonary hypertension or other valvular or congenital disease. Complete diagnostic evaluation should include: (1) measurement of transvalvular flows (liters/min); (2) determination of the transvalvular pressure gradients (mmHg); and (3) calculation of the effective valve areas (cm2) [30].
Stress testing is recommended in patients with equivocal symptoms, and should only be carried out under close monitoring by a physician. Positive findings suggestive of hemodynamically significant aortic stenosis include the development of symptoms, limited exercise tolerance, and a blunted blood pressure response to exercise. In all such cases, surgical replacement of the aortic valve is indicated.
Medical therapy for aortic stenosis is primarily relegated to the prevention of endocarditis and the control of arterial hypertension. As most asymptomatic patients lead a normal life, no interventions are typically considered. Yet, there are some studies that have shown slowing of disease progression with statins. While there are theoretical benefits for the use of ACE inhibitors, studies so far have not shown significant benefits on this disease progression [31, 32]. Nevertheless, once symptoms develop, prompt intervention should be offered to prevent morbidity and mortality. In some patients, interventional radiological therapy using balloon aortic valvotomy can effectively reduce the transvalvular pressure gradients. This procedure uses percutaneously inserted catheters advanced into the aortic valve, then a balloon is inflated to fracture calcific deposits and separate fused commissures [33, 34]. Though successful at providing clinical improvements, the post-procedure valve area rarely exceeds 1.0 cm2, and aortic regurgitation is often created, thus increasing the burden on the left ventricle. To date, the rate of significant complications (10 %) and symptomatic restenosis (6–12 months) unfortunately makes balloon valvotomy an undesirable substitute for aortic valve replacement in adults with aortic stenosis [7]. Yet, percutaneous aortic balloon dilations may be considered as a bridge to surgical aortic valve replacement or transcatheter aortic valve replacement in patients with severe symptomatic aortic stenosis [8].
Aortic valve replacement is technically possible at any age, and is the treatment of choice for aortic stenosis in most adults [35]. Yet, the degree of stenosis mandating surgery in asymptomatic patients remains an issue of debate. Nevertheless, the degree of improvement following aortic valve replacement is directly related to preoperative left ventricular function; patients with depressed ejection fractions caused by excessive afterloads demonstrate significant improvement in left ventricular function after aortic valve replacement. Conversely, if depressed left ventricular function is caused by myocardial insufficiency, improvement in left ventricular function and resolution of symptoms may not be reversed after valve replacement. In general, survival is improved for patients undergoing aortic valve replacement, with the possible exception of a subset of patients with severe left ventricular dysfunction caused by coronary artery disease [36, 37]. In summary, in contrast to the dismal survival rates for patients with untreated severe aortic stenosis, the long-term survival of patients who have undergone aortic valve replacement approaches the rate in the normal population. Therefore, it is recommended that patients with severe aortic stenosis, with or without symptoms, who are undergoing coronary artery bypass surgery should also undergo aortic valve replacement at the time of the revascularization procedure. Similarly, patients with moderate-to-severe aortic stenosis undergoing surgery for the replacement of other heart valves or an aortic root repair should also undergo an aortic valve replacement as part of their overall surgical procedure. Hence, in the absence of contraindications, aortic valve replacement is indicated in virtually all symptomatic patients with severe aortic stenosis (Table 34.5).
• Symptomatic patients with severe aortic stenosis alone or: – Undergoing coronary artery bypass surgery. – Undergoing surgery on the aorta or other heart valves. |
• Patients with moderate aortic stenosis and: – Undergoing coronary artery bypass surgery. – Undergoing surgery on the aorta. – Undergoing surgery on other heart valves. |
• Asymptomatic patients with severe aortic stenosis and left ventricular systolic dysfunction typified by: – Abnormal response to exercise (e.g., hypotension). – Ventricular tachycardia. – Marked or excessive left ventricular hypertrophy (>15 mm). – Valve area <0.6 cm2. – Prevention of sudden death without the findings listed. |
In recent years, transcatheter aortic valve replacements have been increasing in use. According to ACC/AHA guidelines, transcatheter aortic valve replacement is a reasonable alternative to surgical aortic valve replacement in patients who meet an indication for replacement and/or who have a high surgical risk for surgical aortic valve replacement. However, transcatheter aortic valve replacement should only be performed in patients with an expected post-procedure survival longer than 12 months [8, 38, 39]. For a more detailed discussion of these valves, see Chap. 36.
Currently, there are two areas of major controversy in the management of aortic stenosis including: (1) the asymptomatic patient with a severe aortic stenosis; and (2) the patient with low ejection fraction with a reduced gradient aortic stenosis [17, 18]. There is low (1–2 %) risk of sudden death or rapid progression to symptoms in the asymptomatic patient with a severe aortic stenosis. Adverse clinical outcomes are more likely in the asymptomatic patient with severe aortic stenosis who demonstrates more rapid progression of hemodynamic parameters, such as: (1) an increase in aortic jet velocity greater than 0.3 m/s/year; or (2) a decreasing aortic valve area greater than 0.1 cm2/year. Therefore, other than in a small selected group of patients, the risk of surgery may still exceed any potential benefits in this group of patients, i.e., those with a severe aortic stenosis with normal ventricular function who are truly asymptomatic. On the other hand, patients with low ejection fractions and reduced gradient aortic stenosis may present an even more challenging problem. These complexities partly lie in the difficulty to distinguish this entity from those patients with reduced ejection fractions and only mild-to-moderate aortic stenosis; this latter group will not benefit from aortic valve replacement. It should be noted that patients with severe aortic stenosis who present with reduced ejection fractions and reduced gradients will ultimately face increased operative mortality. The use of dobutamine stress echocardiography to measure the pressure gradients and the effective valve areas, both during baseline and at stress, can help determine the true severity of aortic stenosis [40]. It should be noted that, in general, patients with reduced ejection fractions with low transvalvar gradients who elicit no response to stress such as inotropes have poorer outcomes, even with surgery.
34.3.1.2 Aortic Sclerosis
Aortic sclerosis is a common finding in older patients, and is present in approximately 25 % of patients older than 65 years [16, 17]. The classic findings of aortic sclerosis include focal areas of valve thickening with otherwise relatively normal leaflet mobility. It is important to note that, by definition, valvular hemodynamics in these patients are within normal limits. In other words, other than the presence of a systolic murmur, these individuals elicit no clinical signs or associated symptoms. Histologic findings in aortic sclerosis include focal subendocardial plaque-like lesions with accumulations of lipoproteins. The similarity of these findings to atherosclerosis suggests that both are, in some way, an age-related process.
Despite the lack of valve-related symptoms with aortic sclerosis, it is generally associated with an increased risk of cardiovascular mortality. This may be related to the development of coronary artery disease and/or occasionally to a progression to severe aortic stenosis. Thus, while symptoms in the patient identified with aortic sclerosis may be initially benign, these individuals warrant close cardiovascular follow-ups.
34.3.1.3 Aortic Regurgitation
Aortic regurgitation results from a structural defect in the aortic valve that allows for blood flow to reverse direction across the valve during diastole (i.e., re-enter the ventricle). The etiologies of aortic regurgitation are best discussed if one subdivides this disease into acute or chronic regurgitation (Table 34.6). The majority of such lesions result in chronic aortic regurgitation, with insidious dilatation of the left ventricle. In contrast, lesions responsible for acute aortic regurgitation may result in sudden catastrophic elevation of left ventricular filling pressures, reduction in cardiac outputs, and/or sudden death.
Table 34.6
Etiologies of aortic regurgitation (subdivided by presentation time)
Acute | Chronic |
---|---|
Infective endocarditis Aortic dissection Trauma | Idiopathic aortic root dilatation Congenital bicuspid valves Calcific degeneration Rheumatic disease Infective endocarditis Systemic hypertension Myxomatous proliferation Ascending aortic dissection Marfan syndrome Syphilitic aortitis Rheumatoid arthritis Osteogenesis imperfecta Giant cell aortitis Ehlers-Danlos syndrome Reiter’s syndrome Discrete subaortic stenosis Ventricular septal defects with aortic cusp prolapse |
Chronic Aortic Regurgitation
Valve damage that results in progressively larger retrograde flows across the aortic valve produces the condition of chronic aortic regurgitation. The patient’s left ventricle responds to the volume load of aortic regurgitation with several compensatory mechanisms such as an increase in end-diastolic volumes and a combination of eccentric and concentric hypertrophy [41]. The increased diastolic volume allows the ventricle to eject a larger total stroke volume, thereby initially maintaining stroke volume within a relative normal range. As a result, the majority of such patients remain asymptomatic for prolonged periods of compensation, during which time they maintain forward stroke volume within the normal ranges. Yet, after a while, the compensatory mechanisms become inadequate, and further increases in afterload result in reduced ejection fractions. Once the left ventricle can no longer compensate, patients typically present with symptoms of: (1) dyspnea and exertional angina, reflecting declining systolic function; (2) elevated filling pressures; and/or (3) diminished coronary flow reserves of the hypertrophied myocardium [42]. Several natural history studies have identified age and left ventricular end-systolic pressures (or volumes) as predictive factors associated with higher risks of mortality in these clinical populations (Table 34.7) [7].
Table 34.7
Natural history of aortic regurgitation
Asymptomatic patients with normal left ventricular systolic function | • Progression to symptoms and/or left ventricular dysfunction • Progression to asymptomatic left ventricular dysfunction • Sudden death | <6 %/year <3.5 %/year < div class='tao-gold-member'>
Only gold members can continue reading. Log In or Register a > to continue
![]() Stay updated, free articles. Join our Telegram channel![]() Full access? Get Clinical Tree![]() ![]() ![]() |