Abstract
The goal of predictive monitoring is to predict or detect adverse events far enough in advance of conventional detection methods to allow for earlier treatment and improved outcomes. The first example of a commercially available predictive monitoring system for neonatal intensive care unit patients is the heart rate characteristics (HRC) index monitor, which analyzes heart rate patterns for signs of incipient illness. The three HRC identified in preterm infants with sepsis and incorporated into the HRC index algorithm are decreased variability, increased sample asymmetry toward more decelerations and fewer accelerations, and decreased sample entropy, a measure of signal complexity. In real time, the monitor runs the algorithm on the prior 4096 heartbeats, and then averages 12 hours of data to calculate and display a score that was validated to be the fold-increased risk of clinical deterioration from sepsis in the next 24 hours. In a large randomized clinical trial of 3003 very low birth weight infants, those with the score displayed to clinicians (with no mandate about when to start antibiotics) had significantly lower all-cause and sepsis-associated mortality compared with control infants. A limitation of the HRC index is that an elevated score is not specific for sepsis and may occur in other conditions associated with nonspecific inflammation such as respiratory failure and brain injury. Also, since it was developed as fold-increased risk and since the risk of sepsis diagnosis on any particular day is low, the positive predictive value of a high score for imminent diagnosis of sepsis is low. Nonetheless, this continuous physiomarker can be a useful tool for identifying some infants in the early stages of sepsis or necrotizing enterocolitis. Whether adding respiratory analysis or laboratory tests improves the diagnostic utility of HRC monitoring is a subject of current and future research.
Keywords
apnea, heart rate characteristics, heart rate variability, necrotizing enterocolitis, NICU, periodic breathing, preterm infant, sepsis, very low birth weight infant
- •
Sepsis and necrotizing enterocolitis (NEC) are major causes of mortality and morbidity for preterm infants in the neonatal intensive care unit (NICU), and earlier detection and treatment are likely to improve outcomes.
- •
Abnormal heart rate characteristics (HRC) of decreased variability and transient decelerations occur in some infants with sepsis and NEC. These abnormal patterns often begin hours or even days before more obvious clinical signs become apparent, and they cannot be discerned through conventional heart rate monitoring.
- •
An HRC monitor (HeRO monitor) was developed as an early warning system for sepsis in NICU patients. The HRC index incorporates three measures (heart rate variability, sample asymmetry, and sample entropy) and is the fold-increased risk an infant will experience a clinical deterioration consistent with sepsis in the next 24 hours.
- •
Display of the HRC index to clinicians was shown to reduce all-cause mortality by 22% and sepsis-associated mortality by 40% in a randomized trial of 3003 very low birthweight preterm infants.
- •
Adding analysis of respiratory characteristics to HRC may improve predictive monitoring algorithms. For example, cross-correlation of heart rate and oxygen saturation can detect deceleration-desaturation episodes that may accompany sepsis-associated apnea.
- •
Predictive monitoring is a screening tool that supplements but does not replace clinical judgment.
- •
Adding targeted biomarker screening to continuous physiomarker monitoring (HRC or combined cardiorespiratory metrics) may assist in decisions about testing and therapy.
Predictable Systemic Inflammatory Crises in the Neonatal Intensive Care Unit
Preterm infants are susceptible to late-onset sepsis and necrotizing enterocolitis (NEC), which, despite preventative efforts, continue to be major causes of mortality and morbidity in this population. Recent epidemiologic surveys indicate that the incidence of septicemia has decreased amongst very low birth weight infants (VLBW <1500 g) from about 25% to about 15%, due in part to improved hand and central line hygiene and increased breast-milk feeding. Unfortunately the incidence of NEC has not fallen in the same time period, remaining at about 5% to 10%. Mortality related to septicemia and NEC is reported in the range of 15% to 20%, and survivors have a higher risk of long-term neurodevelopmental impairment compared with age-matched preterm infants without these complications. Adverse outcomes may be attributed in part to brain damage from circulating inflammatory mediators and also to cardiovascular compromise, leading to ischemic or hypoxic tissue damage. Early prediction or detection of sepsis and NEC, prior to overt clinical deterioration, should lead to improved short- and long-term outcomes.
In this chapter we will briefly discuss the value of prenatal or early postnatal risk-based scores that incorporate cardiovascular data for prediction of adverse events or conditions diagnosed days or weeks later. We will then focus on early warning scores that reflect a systemic inflammatory response and predict imminent clinical deterioration from sepsis or NEC. We will primarily describe heart rate characteristics (HRC) monitoring, since this is the area that has the largest body of research and development. We will also discuss changes in breathing patterns and in cardiorespiratory interactions that may occur in the early phases of these potentially catastrophic illnesses. We will address important questions and controversies and present challenges and future directions for research in this field.
Of note, the terminology throughout the chapter will include heart rate variability (HRV) and HRC. HRC include HRV together with other measurements that reflect potentially pathologic heart rate (HR) patterns in preterm infants, such as repetitive brief decelerations.
Risk Prediction Incorporating Prenatal Heart Rate Data
Predicting the risk of future adverse events can be useful for clinical and research purposes and can include both fetal and early postnatal assessment of cardiovascular compromise. Abnormal fetal HRC of decreased variability and superimposed decelerations are commonly used as an indication of fetal distress and are associated with adverse perinatal outcomes. These abnormal fetal HRC can be an indicator of acidemia, uteroplacental insufficiency, or a systemic inflammatory response and may predict the risk for postnatal ischemic or inflammatory conditions. A study in growth-restricted human fetuses drew a link between low HRV and increased middle cerebral artery perfusion, a measure of impaired tissue oxygen delivery that could predict ischemic complications postnatally. In fetal sheep, the intrauterine administration of an inflammatory stimulus led to depressed fetal HRV and was associated postnatally with intestinal inflammation and altered mucosal integrity, which could increase the risk of NEC and intestinal perforation if this finding translates to human fetuses.
The problem with current methods of assessing fetal HR is that they are primarily qualitative and prone to interobserver variation, which limits the predictive value for future adverse events. Moorman and colleagues, who developed the neonatal HRC index monitor described later in this chapter, also developed quantitative methods for analyzing abnormal fetal HRC (low variability and superimposed decelerations) and showed good correlation between this quantitative assessment and obstetricians’ impressions of “nonreassuring fetal HR.” More work is needed to determine whether quantitative measures of fetal HRC provide information that could impact perinatal and postnatal management in a way that improves neonatal outcomes.
Early Postnatal Predictors of Late Events or Outcomes
Assessment of HR, oxygenation, and perfusion in the first minutes, hours, and days after birth can provide important information about the well-being of newborn infants that might be useful for prediction of adverse events or outcomes diagnosed much later. Well-known examples of scores used to assess newborns in the first minutes to hours after birth are the Apgar, Score for Neonatal Acute Physiology (SNAP), Score for Neonatal Acute Physiology (SNAP) Perinatal Extension-II (SNAPPE-II), and Clinical Risk Indicator for Babies (CRIB-II) scores. The Apgar score includes assessment of HR, breathing, oxygenation, and other variables in the first minutes after birth and has some association with mortality and adverse neurologic outcomes. The SNAPPE-II and CRIB-II were developed to measure the severity of illness and mortality risk in NICU patients using clinical and laboratory indices in the first 12 hours after birth. Metabolic acidosis (reflecting cardiovascular compromise) is incorporated into both of these scores, and lowest blood pressure is included in SNAPPE-II. The advantage of these scores is that they do not require specialized monitoring equipment, but the disadvantage is that they require some effort to retrieve the data and perform the calculations. While these scores were designed to predict mortality, they have been found to predict other adverse outcomes, but serial assessment beyond the day of birth does not appear to accurately predict mortality or morbidities such as septicemia or NEC.
A number of groups have sought to identify new physiologic metrics in the first hours or days after birth to predict later adverse events in preterm infants. One study found low HRV in the high-frequency spectrum in the first week after birth in preterm infants who developed NEC. Another study reported that a “PhysiScore” that included low HRV in the first 3 hours after birth predicted morbidities including sepsis in a small cohort of preterm infants. Early assessment of the HRC index was also studied for its ability to predict later events or outcomes. A high average HRC index in the first week after birth (reflecting low HRV and decelerations) was associated with late-onset septicemia but did not predict NEC after adjusting for gestational age (GA).
Further research is needed to determine whether the analysis of fetal or early postnatal HR patterns can identify infants at high risk for various pathologies. Validated risk scores identifying the highest risk infants among the inherently high-risk preterm population could lead to targeted strategies to prevent or mitigate the damage from sepsis and NEC. Illness severity or risk scores may also be used as an objective measure of patient acuity for stratification in clinical trials, for comparing outcomes between units, or for resource allocation.
Risk Prediction for Imminent Clinical Deterioration
Early warning systems, in contrast to the risk scores described previously, are designed to detect subtle pathologic signs predicting imminent deterioration before clinicians would recognize that a patient is ill. Often there are changes in vital sign patterns such as HRV and respiratory rate variability that would not be detected by even the most vigilant and experienced clinicians through inspection of conventional intensive care unit (ICU) monitors. In preterm infants this is especially true, since signs of sepsis or NEC such as apnea with associated HR deceleration and oxygen desaturation are nonspecific and occur in healthy preterm infants. A continuously displayed risk score might detect a worsening in the cardiorespiratory pattern over an infant’s prior baseline.
Fig. 20.1 depicts the potential value of early warning systems for detection of sepsis before there are obvious clinical signs. A key point with any such system is that clinician judgment is not replaced but supplemented, as reflected in the figure by the clinician carefully evaluating an infant with a rising risk score and considering the appropriate course of action. The decision about whether to obtain cultures and start antibiotics would incorporate an assessment of the infant’s risk factors, clinical signs, vital sign trends, and laboratory data. Another key point is that for early warning systems to improve patient outcomes, there needs to be a significant time interval between the risk score rising and the time when clinicians using conventional systems would have recognized illness and initiated interventions. How much lead time is required to improve outcomes is debatable, but likely to be more than a few hours. With the HRC index monitor described later in this chapter, some infants have “spikes” in their score several days prior to clinical deterioration, which might reflect a sepsis prodrome and the opportunity for earlier intervention.

Heart Rate Regulation by the Autonomic Nervous System
In order to optimally utilize the data presented by the HRC monitor, it is important to have a basic understanding of regulation of HR in health and disease. Beat-to-beat HRV is regulated by the autonomic nervous system (ANS). Sympathetic activation leads to the release of norepinephrine, which acts on beta adrenergic receptors in the sinoatrial node to trigger acceleration in the HR. Parasympathetic activation leads to the release of acetylcholine, which binds to muscarinic cholinergic receptors leading to transient slowing of the HR. In the healthy state, the two branches of the ANS are balanced, with sympathetic activity associated with low-frequency HRV (0.04 to 0.15 Hz) and parasympathetic activity associated with high-frequency HRV (0.15 to 0.4 Hz in adults). In conditions of stress, adrenal glucocorticoid release leads to sympathetic nervous system activation (the so-called fight-or-flight response), which increases the HR. The parasympathetic nervous system, in contrast, generally regulates organ functions that occur when the body is not acutely stressed (so-called rest-and-digest responses) but is also important in regulating the systemic inflammatory response.
Blood pressure and breathing impact HR and HRV through feedback systems. In the arterial baroreflex, an acute increase in blood pressure leads to decreased sympathetic and increased parasympathetic tone, with an associated decline in HR. Baroreflex sensitivity may be depressed in pathologic conditions such as congestive heart failure, leading to low HRV. Respiratory sinus arrhythmia (RSA) is a normal reflex whereby HR increases during inhalation and decreases during exhalation. The basis of RSA is thought to be increased intrathoracic pressure as the lungs fill, causing decreased venous return to the heart. The decline in pressure in the right atrium leads to increased sympathetic and decreased parasympathetic output and a rise in HR, keeping cardiac output stable. On exhalation the process is reversed, leading to HR deceleration. Sepsis can impact respiratory rate and blood pressure, thereby indirectly altering HRV, but pathogens and inflammatory mediators also exert direct effects on adrenergic and cholinergic responses.
The ANS not only regulates HR but also plays a critical role in host defense. In the cholinergic anti-inflammatory response, vagus nerve activation leads to suppression of inflammatory cytokine release following binding of acetylcholine to nicotinic cholinergic receptors on leukocytes. The transient decelerations in HR seen in the course of sepsis and NEC may thus, at least in part, represent a protective parasympathetic nervous system response to pathogens or tissue damage.
Measurement of Heart Rate Variability Using Linear (Time and Frequency Domain) and Nonlinear Mathematical Methods
Research in complex physiologic systems has grown exponentially over the past several decades, leading to a more complete understanding of methods to analyze HRV. Basic time series analysis involves measuring the standard deviation of interheartbeat (RR) intervals. After removing or replacing an artifact (e.g., noisy signal due to motion or loose leads), detection of R wave peaks in the QRS complex is performed. Ectopic or blocked beats are then discounted so that only normal RR intervals are analyzed. A frequently used measurement is therefore called SDNN, or standard deviation of normal-to-normal beats. Two other commonly used time domain measures are the root mean square of successive differences (RMSSD) and the proportion of intervals, which differ by more than 50 milliseconds from the prior interval (pNN50) and can be an indicator of parasympathetic activity. Since neonates have a faster HR and shorter RR intervals than adults, pNN20 may be more appropriate than pNN50. HRV can also be assessed in terms of mathematical moments of probability, including mean (first moment), variance (second moment), skewness (third moment), and kurtosis (fourth moment). Skewness and kurtosis both describe the shape of a probability distribution, where skewness measures the asymmetry and direction of the tails and kurtosis measures its sharpness or flatness. When considering a histogram of all HRs for an infant for a specific period of time, skewness and kurtosis can be used to assess the relative number and depth of decelerations compared with accelerations.
Frequency domain measurement of HRV may also be performed to quantify low- and high-frequency fluctuations, with the former related to sympathetic nervous system activity and the latter thought to primarily reflect parasympathetic (vagal) activity. Power spectrum analysis identifies dominant periodic variation in HR over time, thereby separating frequency components. Sympathetic HR activation occurs more slowly, in the range of about 0.04 to 0.15 Hz, or one cycle of increasing and decreasing HR every 7 to 25 seconds. Parasympathetic responses occur much faster, resulting in high-frequency HRV in the range of 0.15 to 0.4 Hz in adults, or one cycle of rise and fall in HR every 2.5 to 7 seconds. This corresponds to the frequency of respirations in adults and represents RSA, as described previously. While the presence of RSA reflected as high-frequency HRV is commonly used in adults to assess parasympathetic nervous system functioning, the evidence linking RSA to HRV in neonates is limited. The rapid and irregular respiratory rate of neonates makes RSA analysis more complicated, with some studies using a frequency of 0.4 to 1 Hz (respiratory rate 24 to 60) for power spectrum analysis. If the respiratory rate is more than half the HR (e.g., RR 70 and HR 120), a phenomenon termed cardiac aliasing may occur, which leads to the inaccurate estimation of the magnitude of RSA contribution to HRV.
In addition to the linear mathematical methods for time and frequency HRV analysis, nonlinear equations may also be used, which account for the nonstationarity of interbeat intervals. One commonly used measure is detrended fluctuation analysis (DFA), which quantifies the magnitude of self-similarity or fractal-like correlation of interbeat intervals within a time series. This analysis calculates short- and long-term α values from RR-interval time series that represent a fractal scaling exponent. DFA of HR or blood pressure time series data have been used to predict intraventricular hemorrhage (IVH) in preterm infants. Various entropy measurements can also be used to assess HRV. Sample entropy is a measurement related to approximate entropy that removes bias in short time series and was incorporated into the HRC index algorithm to account for both decreased variability and transient decelerations prior to neonatal sepsis.
Developmental and Pathologic Factors Impacting Heart Rate Variability and Heart Rate Characteristics
Over the course of postnatal development, HR declines from about 150 beats per minute in preterm infants in the first week after birth to 135 beats per minute by 30 to 40 weeks of postmenstrual age (PMA), and with this decline in baseline HR there may be increased beat-to-beat variability. In addition, an increase in HRV with postnatal development occurs due to a lag in maturation of the parasympathetic nervous system, with relatively higher sympathetic tone in the initial weeks after birth. All components of the HRC index described later in this chapter (HRV, sample entropy, and sample asymmetry) have been found to change with increasing gestational and PMA, likely reflecting both physiologic maturation and stabilization of the cardiorespiratory and other systems.
Decreased HRV occurs in a number of acute or chronic disease processes across the age spectrum. In adults, low HRV has been associated with heart disease, renal failure, diabetes, smoking, brain injury, and sepsis. HRV depression can have prognostic significance and has been linked to mortality and other adverse outcomes after myocardial infarction in patients with chronic heart failure and in patients with renal failure on hemodialysis. In fetuses, good beat-to-beat variability is seen as reassuring, whereas low HRV is a sign of distress and may be associated with poor perfusion, acidosis, or a fetal systemic inflammatory response triggered by intrauterine infection. In preterm infants in the NICU, infection is an important cause of decreased HRV and abnormal HRC.
Tachycardia may occur in some neonates with sepsis due to sympathetic activation or hypovolemia but is not an ideal component of early warning systems, since it is usually a late sign associated with advanced illness. In the HRC monitor randomized controlled trial (RCT), HR was not higher within 24 hours of diagnosis of 488 cases of septicemia in 1489 VLBW infants, as compared with HR at other times during the NICU stay (159 vs. 160 beats per minute). Another study of 1065 VLBW infants with 186 cases of septicemia or NEC also did not find higher HR in the 24-hour period before diagnosis compared with time periods without those illnesses (161 vs. 158 beats per minute). More subtle changes in HR patterns must therefore be used in early warning algorithms.
Two elements of abnormal HRC in sepsis in preterm infants are decreased HRV and repetitive, transient decelerations. The decline in HRV is caused in part by elevated circulating levels of inflammatory cytokines. This was shown in a mouse model in which the administration of Escherichia coli lipopolysaccharide or a single cytokine, tumor necrosis factor alpha, led to reduced HRV. Interleukin-6 elevation has also been linked to low HRV in both animal and human sepsis-like illnesses. In both animal and human studies, administration of glucocorticoids has been shown to reduce cytokine levels and increase HRV.
Transient HR decelerations in sepsis may be associated with apnea, or they may occur during regular breathing or on mechanical ventilation. Vagus nerve firing appears to be a major mechanism, and thus these transient decelerations may reflect activation of the cholinergic anti-inflammatory response. In a mouse model, intraperitoneal injection of various bacterial or fungal pathogens very quickly led to repetitive, brief drops in HR during regular breathing. These decelerations were terminated by atropine and associated with activation of vagal nuclei in the brainstem, demonstrating both afferent and efferent signaling in response to pathogens. In this mouse model, the repetitive decelerations did not recur on repeat injections of the same pathogen a few hours or even days after the initial injection, indicating that there was desensitization to the vagal stimulus.
Development of the Heart Rate Characteristics Index Monitor
Based on the observation of abnormal HRC in neonates with sepsis, a team of clinicians, mathematicians, and engineers at the University of Virginia, in partnership with a company, Medical Predictive Science Corporation, developed an HRC monitor (HeRO or He art R ate O bservation monitor) as an early warning system for neonatal sepsis. The idea was to detect subtle changes in HRC that occur in the hours or even days before an infant develops more obvious clinical signs of illness, prompting initiation of therapy. Earlier therapy could then, at least theoretically, avert worsening of the systemic inflammatory response and improve outcomes.
The challenge in developing the HRC index was to derive an algorithm that reflects more than decreased variability, since the transient decelerations observed in preterm infants with sepsis would inflate the HRV measurement toward a “healthy” level. Extensive work went into analysis of electrocardiogram data to develop a statistical model for prediction of sepsis incorporating measurements of HRV and transient decelerations. Electrocardiography (ECG) data from day 7 after birth until NICU discharge were analyzed in 316 preterm infants, with 155 episodes of culture-positive sepsis or sepsis-like illness (culture-negative but treated with a full course of antibiotics due to a high clinical suspicion of sepsis). The ECG signal was analyzed in blocks of 4096 heartbeats (about 25 to 30 minutes of data) and then divided into 6-hour epochs for further analysis with summary statistics and clinical correlation. The 6-hour epoch including the sepsis event was not analyzed, since this period was considered likely to contain obvious clinical signs of illness. The 14-day period after diagnosis was also “blacked out,” since this was considered a recovery period. The 6-hour epoch preceding the one with the sepsis or sepsis-like event was hypothesized to contain abnormal HRC, a preclinical signature of sepsis, and all other times during the NICU stay were considered “well.” Multiple models were tested for the ability to distinguish sick from well ECG patterns, and the best predictive algorithm included weighted measurements of three HRC components:
- 1.
Standard deviation of RR intervals
- 2.
Sample asymmetry, which quantifies decelerations relative to accelerations
- 3.
Sample entropy, which describes the regularity of fluctuations
The HRC index is the output of a logistic regression model using these measures and represents the fold-increased risk of sepsis being diagnosed in the subsequent 24 hours compared with the risk of sepsis at all times. The algorithm was externally validated on a similar number of infants at a second center and in both groups was highly associated with imminent diagnosis of sepsis.
The HRC index monitor is connected to the standard bedside monitor and does not require extra connections to the infant. It captures the ECG signal and runs the HRC algorithm, and then averages the data from the previous 12 hours and displays an updated score every hour. The averaging step smoothes the score to minimize false-positive spikes related to transient, short-lived events that impact HR patterns. Fig. 20.2 shows three screenshots of the HRC index monitor showing normal HRC and two abnormal patterns with acute and chronic low variability and decelerations, resulting in a high HRC index.

It is important to note that although the HRC index (HeRO score) was designed for early detection of sepsis, it identifies HR patterns that predict imminent clinical deterioration. If, for example, the baseline risk for being diagnosed with sepsis for a preterm infant on any given day in the NICU is 2%, then a score of 3 indicates there is a 6% chance the infant will be diagnosed with suspected or proven sepsis at some point in the next 24 hours. Essentially, a rise in the score indicates that there is a physiologic change in the infant, and clinicians should consider whether that change might indicate an acute systemic inflammatory response due to infection, other illness, or nonspecific stimulus.
An important question in evaluating any new risk predictor such as the HRC index is whether it adds information beyond standard tools. In the case of neonatal sepsis, standard diagnosis involves evaluating clinical signs and laboratory tests. The HRC index was analyzed in comparison and in addition to abnormal laboratory tests (hyperglycemia, metabolic acidosis, a high or low leukocyte count, and elevated ratio of immature to total neutrophils) in 678 infants with 149 episodes of blood culture–proven sepsis. From 6 to 24 hours prior to the positive blood culture, an HRC index above the 90th percentile had a higher odds ratio for septicemia than laboratory values, and logistic regression models showed that there was a significant additive value for sepsis prediction when the HRC index was added to laboratory values. Another study sought to determine whether HRC monitoring adds information to the conventional diagnosis of sepsis, using a combination of clinical and laboratory signs. A clinical score was developed, with points given for feeding intolerance, increased apnea, increased respiratory support, lethargy or hypotonia, temperature instability, hyperglycemia, low or high leukocyte count, or immature:total neutrophil ratio of greater than 0.2. Both the clinical score and the HRC index rose prior to sepsis diagnosis, and combining the two gave better predictive value than either value alone.
Does Heart Rate Characteristics Monitoring Improve Outcomes of Infants in the Neonatal Intensive Care Unit?
This question was addressed in a large, multicenter, randomized clinical trial in which 3003 VLBW infants at nine centers had their HRC index monitored. After obtaining parental consent, the infants were randomized either to have their score displayed or not displayed to clinicians. Clinicians were educated about how the score was developed and instructed to evaluate infants whose scores were rising, but there was no mandated intervention for testing or treatment of infection. The primary outcome of days alive and not on mechanical ventilation in the 120 days after randomization was not significantly different in the display versus control infants. However, all-cause mortality, which was a secondary end point, was significantly reduced to 8.1% from 10.2% ( P = .04). This 22% relative reduction in mortality translated to one extra survivor for 48 VLBW infants or 23 extremely low birth weight infants monitored. Slightly more blood cultures were obtained (1.8 per month vs. 1.6, P = .05) but there was no significant difference in total antibiotic days (15.7 days vs. 15.0, P = .31) in HRC display compared with control infants.
In a subanalysis of the 3003 VLBW infants in the RCT, there were 974 episodes of septicemia in 700 infants, and the incidence of septicemia was 23% in both the display and control groups. Among the infants with septicemia, who were of significantly lower GA than those without, the number of total antibiotic days throughout the NICU stay was statistically significantly higher in the display versus control infants (32 vs. 29 days, P = .047). Mortality within 30 days of septicemia was 40% lower when the HRC index was displayed to clinicians (11.8% in the display group and 19.6% in the control group). An important question is whether the mortality reduction was due to earlier treatment, and this cannot be answered with certainty since the exact time that an infant becomes septicemic is unknown. To explore this question, an analysis of HRC index spikes was performed. Focusing on acute, large increases in the HRC index of 3 above the prior 5-day baseline, 34% of control infants had a spike in the week prior to diagnosis of septicemia, whereas only 22% of HRC index display infants had a prediagnosis spike ( P < .01). This led to the hypothesis that some infants have a sepsis prodrome with abnormal HRC prior to overt deterioration, and treating in this phase of illness may lead to improved outcomes.
Can Other Adverse Events Be Predicted by the Heart Rate Characteristics Index?
A high HRC index during the NICU stay was shown to be associated with an increased risk of death within 7 days in a study of VLBW infants. Another study found that the average HRC index in the first day after birth predicted the risk for death prior to NICU discharge and performed similarly for mortality prediction, compared with established illness severity scores SNAPPE-II and CRIB-II. In this study, the average HRC index over the first week was also associated with risk of bronchopulmonary dysplasia (BPD) and late onset septicemia but was not predictive of NEC. The first-day HRC index was also significantly higher in infants with severe IVH, but whether this reflected IVH that had already occurred or identified a pattern associated with later development of IVH could not be determined. Several other studies have found that a high HRC index is associated with adverse neurologic outcomes. In 65 VLBW infants, a high cumulative HRC index throughout the NICU stay was associated with an increased risk of cerebral palsy and lower scores on the Bayley Scale of Infant Development-II at 12 to 18 months. Another study focusing on extremely low birth weight infants found that the average HRC index in the first 28 days after birth was higher in infants with head ultrasound findings of grade III to IV IVH or cystic periventricular leukomalacia than in those with grade I to II IVH or normal head ultrasound. Higher average HRC index also correlated with white matter injury in those infants with brain magnetic resonance imaging (MRI) and with death or Bayley motor or mental developmental index less than 70 in those with neurodevelopmental testing at 1 year of age.
Since depressed HRV reflects ANS dysfunction, the HRC index could be useful for estimating the severity of brain injury following an asphyxial event. In term infants undergoing hypothermia therapy for hypoxic ischemic encephalopathy, low HRV and a high HRC index in the first 24 hours after birth were associated with moderate to severe findings on electroencephalogram (EEG) and MRI. HRV was also about 50% lower in infants who died compared with those who survived.
Another potential application of the HRC index is the prediction of respiratory failure in preterm infants in the NICU. In a study of 96 urgent, unplanned intubations in 51 infants, multiple physiologic parameters were tested and the HRC index was the best-performing individual predictor of intubation within the next 24 hours, with a receiver operating curve (ROC) area of 0.81.
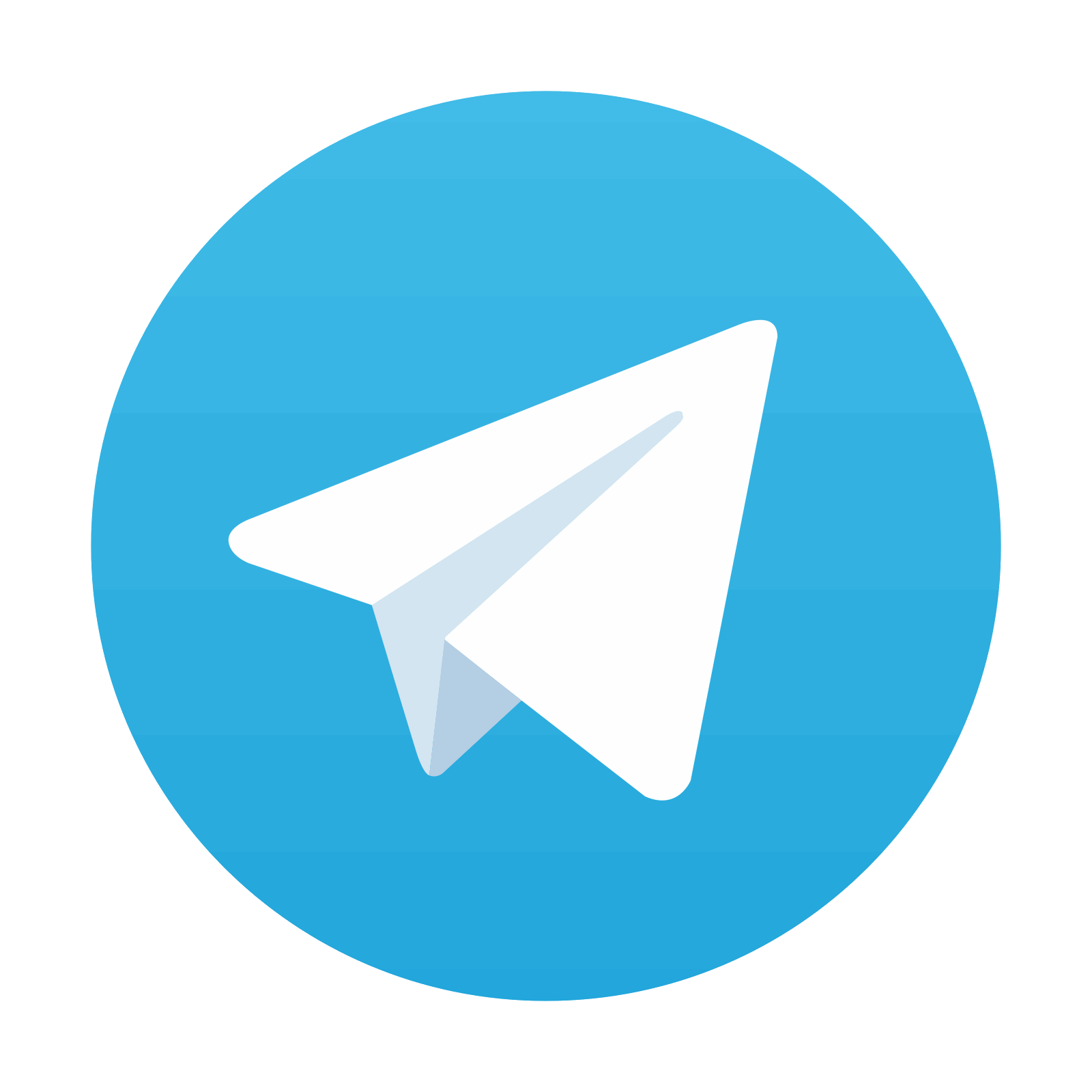
Stay updated, free articles. Join our Telegram channel

Full access? Get Clinical Tree
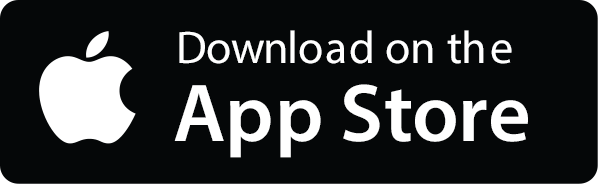
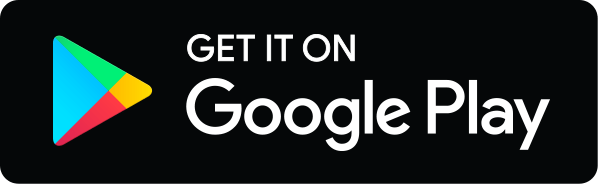