Abstract
Regulation of brain perfusion and oxygen/substrate delivery is, broadly speaking, dependent upon two partially overlapping systems. These are cardiovascular-respiratory regulation by the brainstem autonomic nervous system (ANS) centers and intrinsic cerebral autoregulatory systems (with minor contribution from the ANS). The period of transition from the relatively protected and supported intrauterine environment to the complexities of the external world requires the coordination of the newborn’s cardiovascular and respiratory systems.
The ANS plays a central role in maintaining homeostasis for the infant under fluctuating external conditions. In infants born prematurely, these vital body systems are immature and unprepared to provide reliable support for the infant in the ex utero environment, exposing the infant to the risks of respiratory and hemodynamic instability. Importantly, immature ANS regulation and impaired hemodynamics may lead to brain injury, but may also result in a spectrum of more subtle neurodevelopmental changes whose effects may not become evident until later in childhood.
Significant advances in obstetric and neonatal intensive care have led to a decline in the earlier, often devastating, forms of brain injury seen in the premature newborn. Survival of premature newborns has greatly improved, as has the prevalence of motor impairment among survivors. Unfortunately, psychoaffective disorders and behavioral disorders that may be equally limiting in quality of life remain and thus the spectrum of neurologic morbidity remains prevalent in survivors of premature birth. In this chapter we explore the immature hemodynamic system of the premature newborn and its relation to brain injury, seek to understand the influence of the immature ANS on brainstem responses and how this may affect higher cortical functions, and review how these immature systems may relate to prematurity-related brain injury.
Keywords
autonomic nervous system, cerebral autoregulation, cerebral hemodynamics, intraventricular hemorrhage, preterm brain injury, preterm newborn
- •
The premature infant has an immature cardiovascular and intrinsic cerebral autoregulatory system.
- •
The autonomic nervous system (ANS) is immature in premature newborns, particularly the parasympathetic division which is relatively underdeveloped compared with the sympathetic division at the time of premature birth.
- •
Autonomic dysmaturation may result when the ANS develops in the nonphysiologic conditions of premature ex utero life. Such autonomic dysmaturation of prematurity may have long-term health (e.g., adult hypertension) and psychoaffective consequences.
- •
Knowledge about cerebral hemodynamics in premature newborns is limited by a lack of bedside continuous clinical monitoring technologies.
- •
Cerebral blood flow (CBF) can be measured by the techniques of arterial spin labeling (ASL) magnetic resonance imaging (MRI) and diffuse correlation spectroscopy (DCS). These techniques confirm increasing CBF with advancing gestational age (GA) in premature newborns.
- •
The incidence of severe intraventricular hemorrhage (IVH) and of cystic periventricular leukomalacia has declined in recent decades.
Regulation of brain perfusion and oxygen/substrate delivery is, broadly speaking, dependent upon two partially overlapping systems. These are cardiovascular-respiratory regulation by the brainstem autonomic nervous system (ANS) centers and intrinsic cerebral autoregulatory systems (with a relatively minor contribution from the ANS). The period of transition from the relatively protected and supported intrauterine environment to the complexities of the external world requires the coordination of the newborn’s cardiovascular and respiratory systems.
The ANS plays a central role in maintaining homeostasis for the infant under fluctuating external conditions. In infants born prematurely, these vital body systems are immature and unprepared to provide reliable support for the infant in the ex utero environment, exposing the infant to the risks of respiratory and hemodynamic instability. Importantly, immature ANS regulation and impaired hemodynamics may lead to brain injury, but may also result in a spectrum of more subtle neurodevelopmental changes whose effects may not become evident until later in childhood.
Significant advances in obstetric and neonatal intensive care, ventilation strategies, and management of neonatal hemodynamics have led to a decline in the earlier, often devastating, forms of brain injury seen in the premature newborn, for example, periventricular leukomalacia (PVL) and grade IV intraventricular hemorrhage (IVH). Survival of premature newborns has greatly improved, as has the prevalence of motor impairment and epilepsy among survivors. Unfortunately, this enhanced survival and a reduction in severe motor disabilities have unmasked psychoaffective and behavioral disorders that may be equally limiting in quality of life. Thus, the spectrum of neurologic morbidity remains prevalent in survivors of premature birth. In this chapter we explore the immature hemodynamic system of the premature newborn and its relation to brain injury, seek to understand the influence of the immature ANS on brainstem responses and how this may affect higher cortical functions, and review how these immature systems may relate to prematurity-related brain injury.
Magnitude of the Problem
Prematurity-related brain injury remains a major public health concern. Survivors of prematurity are at risk for long-term motor, cognitive, and psychoaffective disorders ( Fig. 7.1 ). Both the incidence and severity of brain injury in premature infants are inversely related to gestational age (GA). Because advances in survival have been greatest among the sickest, smallest, most immature infants, that is, those at greatest risk for cerebral circulatory instability and brain injury, it is perhaps not surprising that survivors of extreme prematurity have the highest risk for the development of cerebral palsy. Of major concern are the 25% to 50% of ex-preterm children who demonstrate potentially debilitating behavioral or learning problems by school age, with moderate to severe impairment in academic achievement. Children born very preterm are also at increased risk for autism spectrum disorder.

Cerebrovascular insults leading to both hemorrhagic and hypoxic-ischemic injury have long been considered a leading cause of acute and long-term neurologic morbidity in this population (see Fig. 7.1 ). Hypoxia-ischemia/reperfusion injury has been implicated in the causal pathway of prematurity-related brain injury. Systemic hemodynamic impairment is commonly diagnosed and treated in the premature infant and is related inversely to GA at birth. This maturational association in premature infants between disturbed systemic hemodynamics and cerebrovascular injury has led to the notion that the relationship is causative. However, despite plausible extrapolations from human adult and supporting animal studies, establishing a causal link in the premature infant has many challenges, which in turn continues to impede development of rational, safe, and effective interventions.
The principal forms of prematurity-related brain injury are germinal matrix-intraventricular hemorrhage (GM-IVH) and injury to the parenchyma, particularly to the immature white matter. Both anatomic and physiologic features of the premature brain predispose it to cerebrovascular injury (see Fig. 7.1 ). The germinal matrices in the periventricular regions of the developing brain are supported by a profuse but transient vascular system of fragile thin-walled vessels with a deficient basal lamina, no muscularis layer, and a predisposition to hypoxia-ischemia/reperfusion injury increasing their vulnerability to rupture. These germinal matrices are also vulnerable to ischemic insults during hypoperfusion and to rupture during fluctuations in perfusion pressure, the so-called “water-hammer” effect. Over the past two decades, the incidence of GM-IVH in premature newborns has decreased; however, not in infants born below 26 weeks’ gestation where the incidence has remained at about 27%. Both the acute and long-term complications of GM-IVH are more serious in the smallest, sickest infants. The most serious complications of GM-IVH are periventricular hemorrhagic infarction, with an adverse long-term outcome rate exceeding 85%, and posthemorrhagic hydrocephalus, which has an adverse long-term outcome in up to 75% of patients. Fortunately, the incidence of this type of white matter injury has also shown a decline.
Undervascularized end zones in the premature cerebral white matter are susceptible to hypoxic-ischemic injury during periods of decreased perfusion pressure due to incomplete arterial in-growth during prematurity. White matter damage, seen on cranial ultrasound (US) as either hyper- or hypoechoic areas, with IVH in extremely preterm infants, increases the risk of long-term neurologic impairment. Although the severe form of white matter injury, termed as cystic PVL that can be easily appreciated on US now has a very low prevalence, magnetic resonance imaging (MRI) studies describe a high prevalence of diffuse noncystic white matter injury to which cranial US is insensitive. This diffuse form of white matter injury can be detected by MRI in more than 50% of very premature infants.
While parenchymal brain injury in the premature infant has a predilection for the developing white matter, there is increasing recognition of injury and impaired development of gray matter structure and volumes. Neuroimaging and pathology studies have expanded the spectrum of parenchymal lesions in survivors of prematurity by highlighting developmental disruption in both cortical and subcortical gray matter. Brain volume changes can be appreciated in children born prematurely. For example, parietal and temporal lobe brain volumes are smaller in children born in the late preterm period and these findings seem to relate to increased symptoms of anxiety. Differences in early brain structure and volume in premature newborns persist into adulthood with a few areas showing higher volumes (i.e., medial/anterior frontal gyrus gray matter volume), while other areas show reduced volumes (i.e., basal ganglia, thalamus, temporal, frontal, insular, and occipital gray matter). In this same study, similar reductions were seen in the subcortical white matter. These altered brain volumes provide a measure of the structural brain changes that are associated with impaired neurologic outcome, including cognitive and psychoaffective functions in survivors of prematurity.
Hemodynamic Vulnerability in Premature Infants
Immature ANS responses likely contribute to hemodynamic and cardiovascular instability in premature newborns. The prevailing paradigm for hemodynamically mediated brain injury in the premature infant is centered on a confluence of insults emanating from the unstable immature cardiovascular system and immature intrinsic cerebral autoregulation acting on the fragile cerebral vasculature. The brain’s cellular elements are also vulnerable, in particular immature oligodendrocytes, enhancing the risk for brain injury from hemodynamic instability.
The Premature Autonomic Nervous System
The ANS consists of the sympathetic and parasympathetic divisions and has integral control functions on many physiologic aspects of the human body. In addition, the ANS might also provide key input to the development of higher cortical structures involved in emotion, behavior, and thought processing. This important component of our nervous system matures during fetal development and into infancy. Measurement of ANS function is challenging and somewhat limited in the fragile premature newborn. Heart rate variability (HRV), the fluctuation in the length of time between heart beats (R-R intervals), can be analyzed noninvasively to assess sympathetic and parasympathetic tone, providing a window to the ANS in newborns. High-frequency variability reflects parasympathetic function and is influenced by the respiratory rate (respiratory sinus arrhythmia), while low-frequency variability reflects a combination of sympathetic and parasympathetic inputs, and is responsible for baroreflex-induced changes in heart rate (HR). HRV is also influenced by the newborn’s sleep state with active sleep having more sympathetic tone (low-frequency variability) compared with quiet sleep.
Studies have evaluated the relative maturation of the sympathetic and parasympathetic divisions of the ANS using the HRV technique. In these studies, the sympathetic division develops earlier than the parasympathetic division, which shows accelerated maturation at 25 to 30 weeks’ gestation, a time period when many prematurely born newborns may be undergoing transition. Therefore, preterm newborns are born with underdeveloped ANS, especially of the parasympathetic division. The premature engagement of the ANS under the condition of preterm birth may result in “ dysmaturation ,” or a shift in the temporal program of ANS maturation due to aberrant programming.
In addition to prematurity itself, there are a multitude of “unexpected” stimuli in the ex utero environment, which the premature newborn’s ANS may be unprepared to experience and process. The sensory experience in the neonatal intensive care unit (NICU) environment is far harsher than that experienced in the muted intrauterine milieu. Depending on the GA and morbidity of the infant, the extraordinary experiences may include oxygenation disturbances and positive pressure ventilation forces on the premature lungs, hemodynamic instability which is increased by the patent ductus arteriosus, infections, painful procedures, light, and air and temperature changes on the delicate skin, among others. All of these unexpected stimuli can create a challenging environment for maturation of the ANS. Under these conditions, dysmaturation of the ANS may result when the normal programming is disturbed. Importantly, ANS dysmaturation may increase the risk for IVH in premature newborns and for subsequent sudden infant death syndrome and brief resolved unexplained events (BRUE, formerly “apparent life threatening events” [ALTE]). These consequences will be discussed later in the chapter.
Key ANS centers within the brainstem, namely the nucleus tractus solitarius and dorsal motor nucleus of the vagus, receive input from peripheral sensory receptors and exert autonomic control to regulate HR and other critical body functions. In addition, supratentorial ANS centers including the anterior thalamus, anterior cingulate gyrus, and the amygdala integrate the more primitive functions of the ANS with higher cortical processes, a major evolutionary advantage for humans. It is the impaired influence on these higher order cortical structures that likely contributes to the high rate of psychoaffective disorders in children and young adults born prematurely.
The Premature Cardiovascular System
The early period of transition to extrauterine life in the premature newborn is a particularly challenging time for the immature cardiovascular system (see Chapter 1 ), as well as the ANS, as described above. In the premature infant, the normal postnatal closure of fetal circulatory channels, such as the ductus arteriosus and foramen ovale, may be delayed for days or even weeks, compromising cardiovascular efficiency. In addition, the myocardium is also immature, which may compromise systemic blood flow. In certain pathophysiologic conditions, including extreme prematurity, the relationship between systemic blood pressure (BP) and blood flow becomes complex. After normal birth, the term infant experiences a brisk increase in cardiac output, which is determined by HR and myocardial contractility. In the extremely premature infant, this early increase in cardiac output tends to be delayed; in fact, one-third of these infants may experience periods of low cardiac output during the first 24 hours after birth, when the risk for brain injury is particularly high. These low systemic blood flow states are mediated by several mechanisms. First, as detailed previously, the maturation of the fetal sympathetic nervous system precedes that of the parasympathetic system, which remains underdeveloped in the premature infant. This autonomic immaturity leaves the baroreflex and chemoreflex systems underdeveloped prior to term. During periods of low cardiac output, the premature infant becomes particularly dependent on HR; however, with baseline sympathetic activity already close to maximal, the ability to increase cardiac output by increasing HR alone is limited. Second, the immature myocardium has fewer mitochondria and lower energy stores, and its contractility at baseline is close to maximal, with a limited ability to increase stroke volume. The positive pressure ventilation frequently required by extremely premature infants may further reduce cardiac output by decreasing venous return and compressing the cardiac chambers. Third, the premature cardiovascular system is confronted at birth by a sudden and significant increase in afterload during the transition from a low-resistance placental bed to the higher postnatal peripheral resistance. Systemic vascular resistance increases in the postnatal period because of increased release of catecholamines into the circulation. In addition, fluctuations in resistance may result from episodic sympathetic activation during oxyhemoglobin desaturation, suctioning, handling, and hypercarbia, events common in neonatal critical care.
Although cardiac output is the critical measure of overall systemic blood flow, it is not easily measured in the premature infant. Conversely, continuous intra-arterial BP monitoring is widely used to manage hemodynamics in critically ill premature newborns. Hemodynamic management is thus largely pressure based in premature infants. Normal arterial BP may be defined broadly as the range of BP required to maintain perfusion appropriate for the functional and structural integrity of the tissues. In the extremely premature infant, the combination of increased peripheral resistance and myocardial immaturity may translate into decreased tissue blood flow. The above definition of “normal BP” can fail in this situation (see Chapter 3 ).
There have been a number of attempts to define normal BP in premature infants (see Chapter 3 ). Population-based studies have described statistical norms for BP in premature infants without evidence of significant brain injury. In these studies, BP increases with GA at birth as well as with postnatal age during the first week. Other studies have tried to define the limits of normal BP by comparing BP in premature infants with and without brain injury. Despite these studies, the range of “normal BP” remains largely unknown for premature infants, and BP management continues to differ markedly between, and even within, centers. The lack of established normative data for BP notwithstanding, “hypotension” remains commonly diagnosed and treated, especially in smaller and sicker premature infants. Furthermore, there is very little evidence that treatment of hypotension improves neurodevelopmental outcome.
Cerebral Hemodynamic Control in Premature Infants
The hemodynamic physiology of the immature brain increases its vulnerability to injury. Unlike the mature brain where CBF exceeds the ischemic injury threshold by fivefold, the premature brain has significantly lower global and regional CBF, especially in the white matter where there is a much lower ischemic injury threshold. This suggests a reduced margin of safety for cerebral perfusion, but has to be considered in the context of reduced oxygen metabolism in the premature brain (see Chapter 2 ). Monitoring of cerebral tissue oxygenation is an emerging and likely very important monitoring approach during transition of the premature newborn during the first few hours after birth (see Chapter 17 , Chapter 18 ).
Under normal conditions, cerebral perfusion is maintained by a background perfusion pressure provided by the cardiovascular system, which is then “fine-tuned” within the cerebral vasculature by complex intrinsic autoregulatory mechanisms (see Chapter 2 ). However, if cerebral insults are sustained, these responses eventually fail, leading to irreversible brain injury. Cerebral pressure autoregulation maintains CBF relatively constant across a range of cerebral perfusion pressure called the autoregulatory plateau. In addition to these upper and lower pressure bounds, cerebral pressure autoregulation also has a limited impulse-response time, of the order of 5 to 15 seconds. Outside of these pressure and temporal bounds, CBF is pressure passive, with an increased risk of cerebrovascular injury.
Cerebral pressure-flow autoregulation emerges during fetal life but is underdeveloped in the immature brain. With decreasing GA, the autoregulatory plateau is narrower and lower, and normal resting BP is closer to the lower threshold of autoregulation. Although cerebral pressure-flow autoregulation is well characterized in children and adults, this is not the case for the newborn, least of all the sick premature infant. Some studies in stable preterm infants have suggested a lower autoregulatory limit around 25 to 30 mm Hg; however, in the sick premature infant, the existence and limits of cerebral pressure autoregulation remain controversial.
Evidence for an Association Between Systemic Hemodynamic Disturbances and Prematurity-Related Brain Injury
A variety of BP disturbances have been implicated in prematurity-related brain injury, including arterial hypotension, hypertension, cerebral venous hypertension, and/or fluctuating BP. Different mechanisms have been proposed for the role of “hypotension” in GM-IVH. With intact pressure autoregulation, hypotension triggers cerebral vasodilation, with the increased cerebral blood volume potentially leading to the rupture of fragile vessels. Conversely, with disrupted cerebral pressure autoregulation, or with BPs below the autoregulatory plateau, there is greater variability in CBF. During hypotension, hypoxic-ischemic injury to the vessel walls may further disrupt cerebral pressure autoregulation, and vessels may rupture during reperfusion. In addition to GM-IVH, systemic hypotension has also been implicated in the development of white matter injury in premature infants. However, other studies have shown no such relationship. The diastolic closing margin, the difference between the diastolic arterial BP and the critical closing pressure (the arterial BP at which CBF stops), is higher in premature infants that develop GM-IVH. Critical closing pressure, however, increases during the second and third trimesters which may be why some premature newborns show a better ability to handle hypotension without resulting brain injury. Unlike hypotension, sustained hypertension is not commonly diagnosed in premature humans, although it may also result in the development of GM-IVH. Fluctuating BP in premature infants, particularly during positive pressure ventilation, has been associated with GM-IVH in a number of reports, presumably through a “water-hammer” effect of fluctuations in perfusion of the fragile germinal matrix vessels. ∗ However, the role of fluctuating cerebral perfusion in prematurity-related brain injury remains controversial, since several studies found no such association. In summary, the role of systemic BP disturbances and impaired cerebral pressure-flow autoregulation in prematurity-related brain injury is unclear and further studies are needed to more appropriately address this important question.
Doppler US and functional echocardiography studies have identified periods of low cardiac output and low “cerebral” perfusion in premature infants during the early hours after birth. In these studies, the authors observed that these low-flow states are not reliably detected by systemic BP measurements and do not always respond to vasopressor inotropes. In addition, these low cardiac output states are associated with low superior vena cava (SVC) flow, suggesting decreased cerebral perfusion. Up to 80% of premature infants have their lowest SVC flow between 5 and 12 hours after birth. Periods of low SVC flow are associated with disturbed cerebral oxygenation by near-infrared spectroscopy (NIRS). Both the nadir and duration of low SVC flow are associated with severe GM-IVH, and later adverse neurologic outcomes. In summary, the association between systemic BP disturbances, as currently measured and interpreted, and prematurity-related brain injury remains somewhat controversial (see Chapter 6 ).
Resolving the Relationship Between Systemic Hemodynamics and Prematurity-Related Brain Injury: Obstacles to Progress
Several fundamental and interrelated challenges continue to impede our understanding of the relationship between changes in systemic hemodynamics, the immature response of the ANS, and brain injury in the premature infant. Advancing the field will require insight into the interaction between the immature systemic and cerebral hemodynamic systems, the ANS, and how this mediates injury to the immature brain.
Measurement of Relevant Hemodynamic and Metabolic Indices
Currently there are no established techniques for making continuous, quantitative measurements of systemic or CBF in the fragile newborn infant (see Chapter 21 ). Arterial BP, the only continuous systemic hemodynamic signal available in the premature infant, has several important limitations. First, there are no widely accepted noninvasive techniques for acquiring continuous BP in the premature infant. Second, the relationship between BP and systemic blood flow is not constant, particularly during periods of critical physiologic instability. Arterial BP is often used as a surrogate for cerebral perfusion pressure because the effect of venous pressure is disregarded in many clinical situations. However, in premature infants requiring positive pressure ventilatory support, the effect of increased intrathoracic pressure may have a significant impact on cerebral perfusion. At present, techniques for monitoring cerebral perfusion pressure are not available.
Cardiac output is considered the gold standard measure of systemic blood flow (see Chapter 14 ). However, there are currently no well-accepted techniques for the measurement of continuous cardiac output in the premature infant. Similar challenges confront the measurement of continuous CBF volume. Transcranial Doppler US measures cerebral hemodynamics and CBF velocity, but not actual CBF. In the absence of reliable techniques for continuous CBF measurement, a number of intermittent, so-called static approaches have been used to measure quantitative CBF in the premature infant. These techniques are largely based on the Fick principle, using tracers ranging from Xe 133 to oxyhemoglobin measured by NIRS. Another approach has been the use of intermittent measurement of SVC flow by Doppler US as a surrogate for CBF. These studies have also demonstrated the association between abnormally low SVC flow and neurologic morbidity. However, these measurements of SVC flow are not continuous and, in the very preterm infant, less than 50% of the blood in the SVC represents the blood coming from the brain (see Chapter 2 ). Thus, all of the so-called static measurements of CBF suffer from the inability to capture the dynamic nature of cerebral hemodynamics during the period of major physiologic change associated with transition to premature postnatal life.
The ability to measure the relationship between cerebral oxygen demand and supply would provide major insights into the mechanisms of brain injury in premature infants. This is important because it is clear that not only cerebral oxygen deficiency but also excessive cerebral oxygenation may be harmful to the immature brain. In stable premature infants, the premature brain might be “hyper-oxygenated” at baseline compared with the mature brain. NIRS devices measure continuous cerebral tissue hemoglobin saturation as a surrogate measure of the adequacy of cerebral oxygen delivery (see Chapter 17 , Chapter 18 ). The rationale behind this approach is that normal autoregulatory mechanisms maintain appropriate cerebral oxygen delivery and cerebral oxygen extraction; conversely, increasing cerebral oxygen extraction is interpreted as decreased oxygen delivery, presumably due to decreasing CBF. However, this approach assumes that neurovascular coupling is intact in sick premature infants. Furthermore, cerebral oxygen extraction may actually decrease after significant brain insults. For these reasons, measures of cerebral tissue hemoglobin oxygenation may be misleading when considered in isolation.
Understanding CBF in the premature infant has been limited by the lack of bedside techniques capable of measuring regional blood flow within the brain. Not only is global CBF lower in these infants, but blood flow is also particularly low in the most vulnerable white matter regions. Arterial spin labeling (ASL) perfusion MRI at 3 Tesla can measure regional cerebral perfusion noninvasively and without the need for contrast. In a study using pseudo-continuous ASL, frontal brain regions showed a faster increase in CBF with increasing GA compared with the occipital regions. In another study using ASL, neonates born prematurely were found to have higher perfusion at term GA compared with term newborns, indicating a potential effect of ex utero development on brain perfusion. The ASL technique does have some technical challenges in the high-risk preterm neonate, and similar to many other imaging techniques, it is not portable to the bedside and does not allow for a true continuous measurement. Diffuse correlation spectroscopy (DCS) is an emerging technique to monitor CBF in premature newborns. Advantages are that it is noninvasive, portable to the bedside, and provides continuous monitoring of cerebral microvascular blood flow. DCS measures a CBF index, which correlates with CBF as measured by the ASL-MRI technique. In a study using frequency domain-NIRS and DCS, higher indices of CBF, cerebral blood volume, and cerebral hemoglobin oxygenation were found in the temporal and parietal regions compared with the frontal lobe in both premature and term newborns, and this increased with increasing GA. These hemodynamic parameters were also greater in the right hemisphere compared with the left and were higher in male than in female infants. The finding of hemodynamic differences between the hemispheres may be related to different developmental time courses between the right and left forebrain. The etiology of the difference in development between the hemispheres is still being explored and may relate to ductal effects in addition to other factors. DCS and NIRS can be combined to yield quantification of regional cerebral oxygen metabolism (CMRO 2 ). DCS also has limitations with depth of penetration and is sensitive to motion artifacts. Increased use of these newer techniques will hopefully enhance understanding of CBF in premature infants.
Potential for “Dysmaturation” of the Autonomic Nervous System in Premature Newborns
As discussed earlier, at the time of premature birth, the newborn has an immature ANS, with greater sympathetic than parasympathetic influence. The premature engagement of the ANS under ex utero conditions of preterm birth may result in dysmaturation , or a shift in the temporal program of ANS maturation due to aberrant programming. Premature newborns have an increased risk for sudden infant death syndrome and for BRUE/ALTE after discharge from the NICU, which may be due to reduced cardiorespiratory system control from ANS dysmaturation. In a study of premature newborns that developed BRUE/ALTE, there was a paradoxical increase in parasympathetic tone and lower sympathetic tone at 36 weeks’ postmenstrual age, likely restricting the ability of these infants to autoresuscitate by increasing HR and BP during a cardiovascular or respiratory-induced event. Immature ANS function may also increase risk for IVH in premature newborns. Measures of HRV were different in very low birth weight infants who developed IVH compared with infants who did not develop IVH, indicating that impaired ANS function may be an antecedent risk factor for the development of IVH. Brain injury in preterm newborns may alter the trajectory of ANS development which can have long-term consequences on neurologic and psychoaffective development into childhood and adulthood.
Fetal oxygen and nutrient deprivation may have similar dysmaturational influences on ANS development. Two examples of such hypoxemic and potentially nutrient-deprived conditions are complex congenital heart disease and fetal growth restriction with placental insufficiency. The fetal brain, in the third trimester, undergoes rapid growth, maturation, and enhanced complexity of its neuronal connections. Complex forms of congenital heart disease, such as hypoplastic left heart syndrome, are complicated by in utero hypoxemia and hypoperfusion. Fetuses with hypoplastic left heart syndrome have reduced HR and reduced HRV compared with healthy control fetuses at similar GA, suggesting that ANS maturation may be delayed even prior to birth in these fetuses. Similarly, fetal growth restriction is associated with placental insufficiency/failure, nutrient deprivation, and hypoxemia and a recent study found reduced HRV in fetuses affected by fetal growth restriction compared with healthy controls. ANS immaturity under these circumstances may increase the need for early neonatal vasopressor support and mechanical ventilation and may prolong duration of intensive care. Infants who experience suboptimal fetal oxygen and nutrient environments may continue to have reduced HRV, which can be associated with lifelong cardiovascular morbidities.
Characterizing “Significant” Systemic Hemodynamic Insults and Establishing a Temporal Relationship Between Hemodynamic Changes and Brain Insults Is Difficult in Sick Premature Infants
Understanding the role of systemic hemodynamic factors in prematurity-related brain injury has been impeded by a fundamental lack of understanding of the “dose” of hemodynamic insult required to injure the premature brain. Insults capable of causing injury to the premature brain are likely distinctly different from those injuring the mature brain. In the extremely premature infant, the risk period for hypoxic-ischemic brain insults may extend for weeks or months during the prolonged NICU stay. Presumably, injury thresholds exist for brief but severe, mild but prolonged, and repetitive hemodynamic insults, as well as for the cumulative impact of these insults. The prolonged risk period for, and variety of, hemodynamic insults that might theoretically cause brain injury in the premature infant have made it difficult to design appropriate experimental models or to monitor the human premature brain appropriately.
Establishing a temporal association between systemic hemodynamic disturbances and brain insult is particularly challenging in the sick premature infant because the exact onset of brain injury is often unknown and brain injury may be cumulative. Premature infants rarely demonstrate acute signs at the onset of brain dysfunction and therefore may provide little or no clue to the timing of brain insult. Furthermore, neurodevelopmental deficits may only become evident months to years after the initial injury, sometimes as late as school age, or beyond. During this interval, multiple factors such as socioeconomic status and maternal education that are unrelated to the initial insult may significantly influence long-term outcome. In the premature infant, brain injury precedes critical third-trimester events in brain development and neuronal migration is still very active into early infancy. The unfolding of these events after brain injury may have implications for long-term outcomes in infants that are as, or more, important than the primary cerebral injury. On the one hand, normal third trimester developmental events may be derailed by acute injury resulting in acquired brain malformations (disruptions). Conversely, the ameliorating effect of brain plasticity may play an important role in outcome. In addition, other potentially injurious mechanisms may operate before (e.g., fetal inflammation), during (e.g., blood gas disturbances), and after (e.g., apnea and bradycardia) the immediate period of postnatal hemodynamic instability. Of particular importance is the role of infection and inflammation, known to be associated with prematurity-related white matter injury, which may act in concert with hemodynamic insults.
Establishing a causal link between early hemodynamic insults and brain injury is also challenging; specifically identifying acute structural injury during periods of critical illness has been limited by the lack of sensitive portable neuroimaging. Infants are usually too ill to be transported to MRI scanners, especially early in their hospitalization. Cranial US, although sensitive to hemorrhage, is insensitive to and delayed in its detection of diffuse white matter injury. This has been confirmed at autopsy but also in MRI studies that indicate that the majority of white matter injury is undetected by neonatal cranial US. MRI-compatible incubators allow for safer transport of critically ill premature infants to MRI scanners which can advance detection of hyperacute brain injury.
Measures of acute changes in brain function have similarly been difficult in the premature infant, in whom even severe injury may remain clinically silent. The use of the electroencephalogram (EEG) as a monitor of brain function has the advantage of being continuous and noninvasive, especially when using limited-lead amplitude-integrated EEG techniques. Some EEG studies in sick premature infants showed a decrease in EEG amplitude during hypotension. Victor and colleagues found that in infants less than 30 weeks’ gestation, EEG and cerebral oxygen extraction remained normal despite systemic BP levels as low as 23 mm Hg, and suggested that cerebral pressure autoregulation is maintained above this systemic BP level. Indeed, this level of hypotension might represent the “functional threshold” of BP, where cellular function is affected and it is below the documented CBF autoregulatory elbow of 28 to 30 mm Hg (see Chapter 2 , Chapter 3 ). In extremely premature infants, EEG may be less useful as a measure of brain function since the EEG background has a limited range of features at early GAs.
Intrinsic cerebrovascular systems aim to maintain brain oxygen/substrate delivery when systemic delivery fails. These compensatory mechanisms are temporizing at best and, if systemic delivery does not recover, these mechanisms will collapse, elevating the risk for irreversible brain injury. For these reasons, a definition of systemic hypotension, using as a threshold the BP below which cerebral pressure-flow autoregulation begins to fail, appears logical. Because the onset of cerebral pressure autoregulatory failure heralds an elevated risk for cerebrovascular injury at a point prior to irreversible injury, detection of cerebral pressure passivity might provide a sensitive cerebrovascular biomarker to relate to systemic BP changes. However, the presence and characteristics of pressure autoregulation in the sick premature infant remain controversial, in part due to the ongoing lack of established techniques for detecting cerebral pressure passivity. The NIRS hemoglobin difference (HbD) signal, which can be measured continuously, can be time-locked to measurements of systemic BP and applied to the study of cerebral pressure-flow autoregulation in premature infants. This approach can identify periods of pressure passivity using coherence function analysis to identify significant concordance between systemic BP and cerebral HbD changes. The magnitude of pressure passivity during these periods can then be measured using transfer gain analysis. Using this approach, an association between cerebral pressure-passivity and brain injury has been described, as well as between the magnitude of pressure passivity and GM-IVH, and in the term newborn, hypoxic-ischemic encephalopathy. This approach highlighted the prevalence and dynamic nature of cerebral pressure flow autoregulation, with periods of pressure passivity interspersed with apparent autoregulation in most extremely premature infants. Pressure autoregulation is not only affected by BP, but by other influences as well.
There are several limitations, in addition to technical difficulties discussed above, in using the onset of cerebral pressure passivity to define hypotension. Despite constant BP, this threshold may fluctuate, presumably due to other vasoactive factors such as hypoxemia, hypercarbia, and inflammation, among others. Of note is that, among factors affecting CBF, including the BP outside the autoregulatory range, changes in arterial carbon dioxide tension cause the most significant CBF alterations (see Chapter 2 ). Furthermore, preceding insults (e.g., an intrapartum event) may temporarily abolish pressure autoregulation, making it unreliable as a criterion for “normative” definitions of hypotension. Continuous monitoring for cerebral pressure passivity is clearly much needed; however predictable gestational/postnatal age-related BP bounds, between which cerebral perfusion is maintained constant, is a spurious concept.
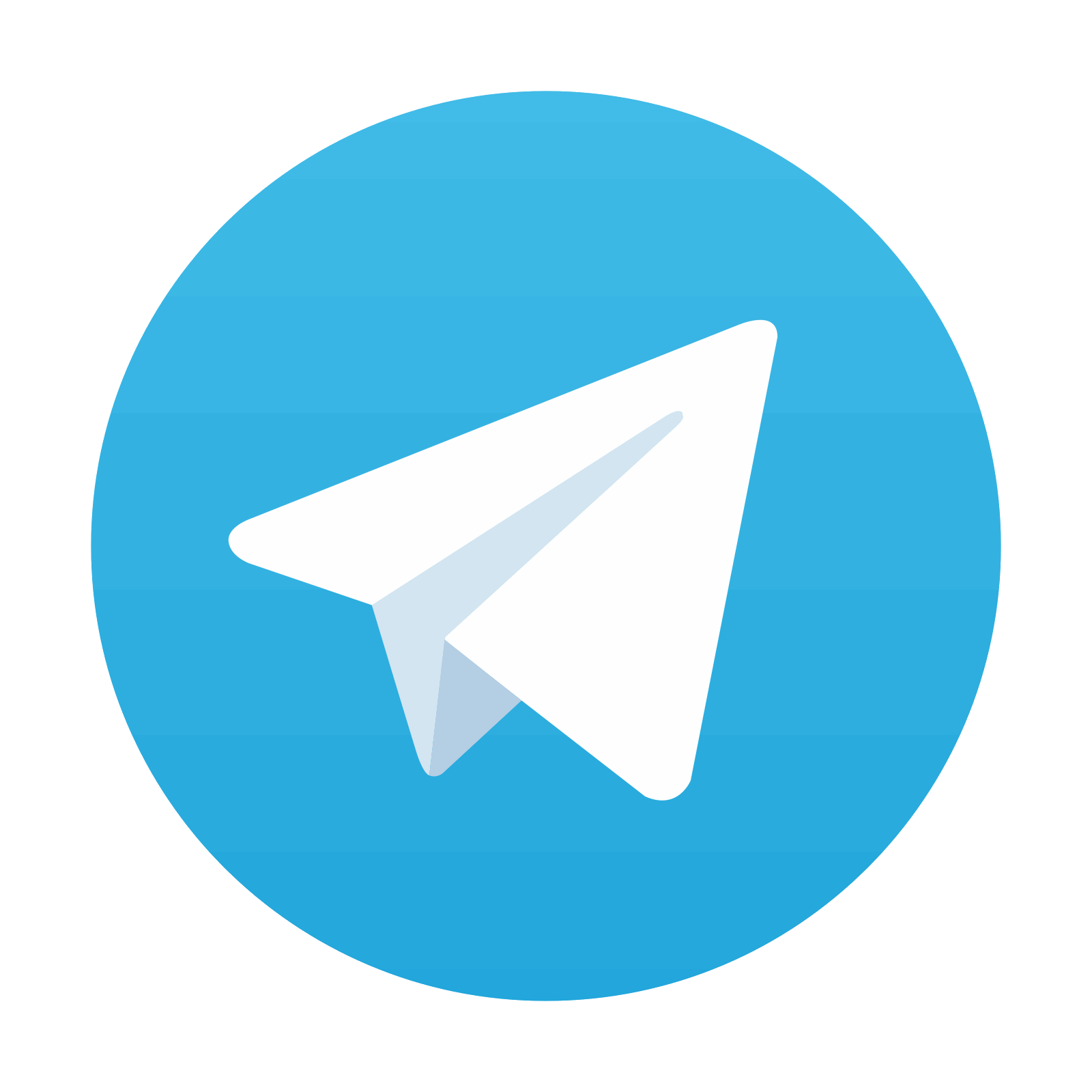
Stay updated, free articles. Join our Telegram channel

Full access? Get Clinical Tree
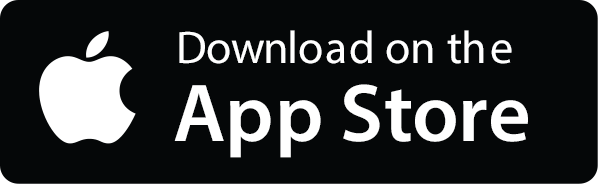
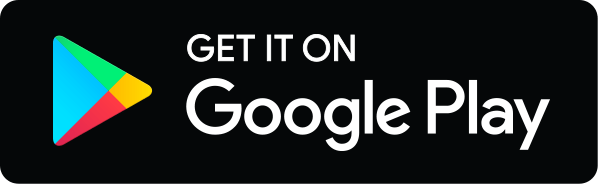