Abstract
Considerable advances in catheter-based therapies have recently allowed interventional cardiac catheterization to play a major role in treating infants with congenital heart disease (CHD). In the neonatal period, a wide variety of such interventions can be performed, either as palliative procedures or as therapeutic interventions by themselves or with surgical procedures (the “hybrid” approach). This chapter summarizes various transcatheter therapies, which are currently performed in neonates with CHD, and discusses the indications, outcomes, and possible complications of these therapies.
Keywords
catheter-based therapy, congenital heart disease, neonates
- •
Transcatheter cardiac interventions currently provide a wide range of different therapeutic or palliative alternatives to surgeries in neonates.
- •
Common catheter-based procedures in neonates include balloon atrial septostomy, balloon valvotomies, perforation of atretic valves, stenting of obstructed vessels, and device closure of abnormal communications.
- •
Interventional cardiac catheterizations are not risk free; common complications can include bleeding, arrhythmia, cardiac perforation, device embolization, vascular access injuries, and even death.
- •
With growing experience in the field coupled with numerous advances in the available low-profile instruments and devices, simple and complex interventional cardiac catheterizations have been performed successfully and more safely in recent years.
Over the past five decades, interventional cardiac catheterization has significantly evolved in the management of pediatric patients with congenital heart disease (CHD), particularly in the neonatal period. Since the first successful catheter-based therapeutic procedure in 1966 by Rashkind and Miller who performed a balloon atrial septostomy (BAS or Rashkind procedure) in an infant with transposition of the great arteries without a thoracotomy, several advances in catheter-based technologies and techniques have been achieved that allowed cardiac catheterization to play a major role in treating both adults and children with congenital and acquired cardiovascular diseases. In the neonatal period, a wide variety of transcatheter interventions are commonly performed, either as palliation or therapy, and either as an alternative to surgical intervention or in coordination with surgery in what has been termed “hybrid” procedures, allowing transcatheter interventions on even the smallest patients.
Among these catheterization procedures are creation or enlargement of atrial communications to improve cardiac mixing, balloon valvuloplasty to treat congenital pulmonary or aortic valvular stenosis, balloon angioplasty or stenting of vessels, such as coarctation of the aorta, ductal stenting in ductal dependent lesions, perforation of the atretic valve, and device closure of the ductus arteriosus. This chapter describes various catheter-based therapies that are currently performed in neonates born with CHD.
Indications for Cardiac Catheterization in the Neonate and Potential Complications
During the past three decades, the indications for pediatric cardiac catheterization have undergone significant changes. With the increasing accuracy of noninvasive diagnostic tools, including echocardiography, computed tomography, and magnetic resonance imaging, cardiac catheterization is no longer considered a mainstay diagnostic tool for most cardiac anomalies, except on rare occasions or when accurate hemodynamic measurements are needed. On the other hand, with the advent of new instruments, it has become more common to perform cardiac catheterization for interventional purposes. This is particularly true in the neonate, where interventional cardiac catheterization can offer successful alternative options to cardiac surgery with potentially lower mortality and morbidity. In addition, for certain conditions, it can be used effectively as an alternative or adjunct to surgical procedures (“hybrid” procedures). Thus, currently, the indications for catheterizations are more likely to be for catheter-based therapies rather than diagnostic purposes in patients with CHD.
The indications for cardiac catheterization usually fall into one of the following categories:
- 1.
Determine the anatomic diagnosis: This could be the case in patients with limited echocardiographic windows, such as patients with lung pathology, as in chronic lung disease (CLD), resulting in poor visualization of cardiac structures. It could also be needed to further delineate structures usually not well seen with echocardiography, such as vascular abnormalities in the systemic or pulmonary circulation, or presurgical and postsurgical intervention, such as with systemic to pulmonary arterial collaterals.
- 2.
Obtain complete hemodynamic evaluation to guide medical and surgical management, such as assessing pulmonary vascular resistance in a patient with pulmonary hypertension or CHD.
- 3.
Perform interventional procedures, such as closure of atrial or ventricular septal defects, occlusion of vascular structures, such as patent ductus arteriosus (PDA), stenting of PDA or narrowed vessels, perforating or balloon dilating atretic or stenotic valves, respectively, and atrial septostomy to facilitate better systemic and pulmonary blood mixing.
Although cardiac catheterization is relatively safe, it can be associated with significant complications, particularly in neonates and small infants. These can be divided into two groups:
- 1.
Major complications. These are significant adverse events that typically lead to compromise needing significant intervention, such as cardiopulmonary resuscitation. Examples include complete heart block, structural injury from intervention related to cardiac perforation or device embolization, vessel perforation, central nervous system (CNS) complications, and death.
- 2.
Minor complications. These tend to be transient and can include blood loss requiring transfusion, hypotension requiring fluid resuscitation or vasopressor-inotrope administration, deep venous thrombosis, pulse loss requiring heparin or a stronger agent such as a tissue plasminogen activator, transient heart block, dysrhythmias requiring treatment, metabolic acidosis due to decreased oxygen delivery to the tissues, and balloon rupture. Vascular complications can be either major or minor and can be particularly common in neonates due to their small vessel sizes. These possible complications deserve special attention and will be discussed more thoroughly in the next section.
Nowadays, with the increased survival of low birth weight infants, advanced technology, smaller sheath sizes, and better intracatheterization monitoring, low birth weight neonates can undergo catheter interventions more safely, though the complication rate is still higher compared with larger infants. Recently, Mobley et al. analyzed the complication rate in neonates in relation to their weight. The overall incidence of complications was higher in neonates weighing less than 2.5 kg compared with those weighing greater than 2.5 kg (34.8% vs. 17.6%, P = .023). This higher overall rate was a result of a greater proportion of minor complications 34.8% in small neonates versus 16.9% in larger ones ( P = .021). However, the percentage of neonates having at least one major complication was the same in both groups (2.2%).
Vascular Access
Femoral arterial and venous access are traditionally the methods of choice for cardiac catheterization in infants using either the right or the left groin. In small newborns, umbilical arterial and venous vessels can also be used usually up to 7 days of age. Other alternative routes for arterial access include carotid, brachial, and axillary arteries. Alternative venous access sites include internal jugular vein, subclavian vein, axillary vein, and transhepatic venous access. Alternative vascular access is occasionally required due to the lack of femoral vascular patency or the need to position the catheter at a particular trajectory not provided through the traditional femoral access. The use of alternative access is safe and effective for performing a wide variety of interventions. In addition, its use may improve the results of selected complex interventional procedures.
Recently, Choudhry and colleagues reported on the successful percutaneous access through the carotid artery using a 21-gauge Doppler needle (Smart Needle) under ultrasound guidance in infants less than 3 months of age. This route can allow the use of larger sheaths (up to 6-French) with no documented arterial occlusions or neurologic sequelae. Using the carotid approach, the catheter can be maneuvered easily in the left heart structures with potentially less technical difficulty.
In more recent years, interventional congenital cardiologists and congenital cardiovascular surgeons have collaborated to obtain access to even larger, more central vessels or even direct access into the cardiac chambers for catheter-based therapies employing “hybrid” procedures.
Complications of vascular access sites account for the majority of adverse events associated with neonatal cardiac catheterization. Injury to the vessel and/or the surrounding area may occur with either venous or arterial access sites, although more significant acute and long-term sequelae are more common with arterial access. In general, vascular injury is more likely in smaller neonates when using large sheaths or catheters, when a patient is on anticoagulation, and after interventional procedures. Complications may include arterial spasm and/or thrombosis causing chronic occlusion, significant tearing or severing of the vessel with extravasation of blood into the surrounding tissue, hematoma, “false” or pseudoaneurysm formation, and creation of an arteriovenous fistula. The frequency of vascular complications has decreased with alterations in access techniques, improvements in availability of size-appropriate equipment for neonates, and meticulous management of the access site following the procedure. With the frequent use of indwelling central venous catheters and/or arterial cannulations and the need for repeated procedures in these small patients (and vessels), the incidence of chronic occlusion of these vessels has increased, necessitating the use of less “standard” access sites and approaches for catheter-based procedures in these patients. These include use of the umbilical vein (in the immediate newborn period), subclavian or jugular veins, or hepatic veins (transhepatic approach) for venous access, or the umbilical artery, the carotid artery (usually via a cut-down technique), the axillary artery for alternative arterial access, or the use of “hybrid” procedures.
Balloon Atrial Septostomy and Septoplasty
Since Rashkind first performed BAS in 1966 in an infant with transposition of the great arteries, the safety and efficacy of this procedure has been well established and has become a lifesaving procedure for patients with CHD who require improved atrial level mixing of blood or atrial decompression. This is typically needed for patients with D-transposition of the great arteries, particularly with intact ventricular septum (IVS) and some forms of double outlet right ventricle (RV). With the use of prostaglandin infusion, the need for BAS has become less urgent as mixing can at times be adequately improved by keeping the ductus patent. Although initial BAS procedures were performed using fluoroscopic guidance, it was Allan and colleagues who first described performing BAS under echocardiographic guidance in 1982. Since then, improvements in echocardiographic imaging have allowed most neonatal BAS procedures to be performed at the bedside under echocardiographic guidance if the atrial septum anatomy is “straightforward” and the septum is not intact. When atrial communication is not present, most centers perform BAS in the catheterization laboratory using either fluoroscopic guidance with biplane imaging alone or, alternatively, fluoroscopy with transthoracic echocardiography (TTE) or transesophageal echocardiography (TEE) to better visualize the septum when septal perforation is required prior to creation of an atrial communication. When possible, TEE imaging is preferred because the ultrasonographer’s hand does not interfere with the fluoroscopic image during the procedure. In small infants in whom a pediatric/infant TEE probe may be too large, successful transesophageal imaging using an intracardiac echo catheter (AcuNav, Acuson/Siemens, Mountain View, California) has been reported. Femoral venous access is preferred to umbilical vein access due to the complexity of the intervention and the more direct course from the femoral vein to the atrial septum. Once access has been obtained in the intubated, sedated neonate, a balloon septostomy catheter is advanced via an introducer sheath to the inferior vena cava and right atrium, across the patent foramen ovale (PFO), and into the left atrium usually under direct visualization using TTE imaging. Once the position of the catheter tip is confirmed to be in the body of the left atrium (and not in a pulmonary vein or across the mitral valve), the balloon at the tip of the catheter is slowly inflated with sterile saline/contrast mixture while simultaneously pulling the balloon back against the left atrial side of the atrial septum. The amount of saline/contrast mixture used to fill the balloon is dependent upon the type of septostomy catheter, the actual size of the left atrium, and the desired size of the resultant septal defect and is usually 2.5 to 3 mL. Next, the balloon inflation lock is secured and the catheter is firmly and rapidly pulled into the right atrium, aiming to produce a tear in the septum primum. Once the balloon has been pulled across the septum, it is quickly deflated so as not to obstruct systemic venous inflow from the inferior vena cava. The procedure may be repeated using serial increases in the inflation volume to fill the balloon until an adequate defect is created or until the maximum volume of the septostomy catheter or the maximum balloon size the left atrium will accommodate has been reached. Once the septostomy procedure has been performed, two-dimensional (2-D) echocardiography and Doppler interrogation are performed to assess the resultant defect size and determine the presence and severity of any residual atrial level restriction to blood flow or mixing. Further echocardiographic assessment of the cardiac function, as well as for evidence of pericardial effusion or damage to any of the cardiac structures, is also recommended following BAS.
Although complications are not common, there have been reports of embolization of balloon fragments or air bubbles in the event of balloon rupture during the procedure (it should be noted here that careful de-airing of the balloon during preparation for BAS should take place to prevent air embolization if balloon rupture should occur). Other complications associated with BAS include heart block or other serious arrhythmias, mitral or tricuspid valve injury, thromboembolic events, such as stroke, and inferior vena cava or pulmonary vein tear or rupture. Death has also occurred. Careful and appropriate echocardiographic imaging prior to and during balloon inflation can decrease the risk of valvular and/or vascular tears or ruptures from occurring. The success rate of BAS in newborns is over 98%, with a procedural mortality of less than 1% and an incidence of major complications reported on the order of 0% to 3%. Hemodynamic improvement is usually noted immediately after a successful BAS with increased oxygen saturations and a decrease in the interatrial gradient. Restenosis after BAS is rarely seen. Some reports have suggested that there is an association between preoperative brain injury in neonates with transposition and performance of BAS. Recently, Mukherjee and colleagues reported that there was a significant, positive association between the Rashkind procedure and the diagnosis of stroke when comparing infants with transposition who either did or did not undergo a BAS procedure. However, the lack of temporal data regarding the timing of BAS and the diagnosis of stroke limited the investigators’ ability to prove causation. In contrast, others have recently published reports that BAS was not associated with an increased risk of clinical stroke, but that there was an increased incidence of preoperative periventricular leukomalacia (PVL) in neonates with transposition of the great arteries that had longer time to surgical repair and/or lower arterial oxygenation prior to intervention. These authors also suggested that BAS was protective against PVL when the procedure resulted in timely improvement in arterial oxygenation. Further studies are needed to better evaluate and define risk factors for preoperative brain injury in this patient population, which may include minimal oxygen saturation after birth and/or length of time from birth to BAS and improvement in systemic oxygenation.
Atrial Septoplasty
Creation or enlargement of an atrial communication in neonates presenting after the immediate newborn period may be difficult due to the presence of a thick or intact atrial septum. Other infants, such as those with hypoplastic left heart syndrome (HLHS), may have malalignment of the atrial septum which is often associated with restriction of pulmonary venous return ( Fig. 32.1A ). In patients such as these, standard BAS techniques may not be sufficient to create a nonrestrictive atrial communication and other transcatheter techniques may be required. When the septum is intact, perforation of the septum to access the left atrium is required. Historically, this was, and often still is, performed using a Brockenbrough needle to puncture the septum. In recent years, radiofrequency (RF)-assisted septal perforation has been reported using a 0.024-inch diameter wire with a 0.016-inch diameter metal tip (Baylis Medical, Montreal, Canada) attached to a dedicated RF generator (Baylis Radiofrequency Generator, Montreal, Canada). The wire is passed within a coaxial catheter that is, in turn, passed within a guide catheter or long introducer sheath/dilator, which has been placed with its tip adjacent to (and in contact with) the right atrial side of the septum. Perforation with this RF wire can be achieved with the application of 5 to 10 W of energy for 2 to 5 seconds. Once mechanical or RF-assisted perforation of the septum and access into the left atrium is achieved, subsequent sequential static balloon dilation (“septoplasty”) is performed, starting with small-diameter balloons, followed by serially larger diameter balloons to a maximum balloon diameter of typically 10 to 12 mm in neonates. When there is a PFO, but the septum is thick or malaligned, septal perforation is often performed in a more “favorable” position along the septum with subsequent septoplasty. The use of coronary and peripheral cutting balloons (Boston Scientific, IVT, San Diego, California) or high-pressure angioplasty balloons has also been reported to be a safe and effective option for septoplasty in neonates (see Fig. 32.1B ). Finally in patients with difficult septal anatomy, an intravascular stent implantation at the atrial septum to maintain septal patency has provided effective palliation until surgical intervention or transplant can be achieved.

Pulmonary Balloon Valvuloplasty for Critical Pulmonary Stenosis
Neonates not diagnosed in utero with the suspicion of critical pulmonary valvular stenosis usually present within the first 24 to 48 hours of life with progressive cyanosis as the ductus arteriosus closes. There is typically an outflow murmur from the stenotic valve. Cyanosis is due to persistent right-to-left shunting at the PFO in the presence of a hypertensive and hypertrophied noncompliant RV. By definition, critical pulmonary valve stenosis is ductal dependent as adequate pulmonary blood flow and oxygenation are dependent on systemic-to-pulmonary artery shunting via the ductus. Management of these infants requires the initiation of a PGE-1 infusion to maintain ductal patency and to augment pulmonary blood flow. Currently, percutaneous balloon valvuloplasty is the initial procedure of choice for neonates with critical or severe pulmonary stenosis. These patients typically have a normal-sized tricuspid valve annulus, a tripartite RV, and a normal pulmonary valve annulus. The RV may be hypoplastic or the cavity may be relatively small due to the often severe right ventricular hypertrophy. The main pulmonary artery usually has evidence of poststenotic dilation from the jet of blood across the stenotic valve and there is almost always predominant right-to-left shunting at the atrial level (PFO) due to the stiff, noncompliant RV. In patients with severe pulmonary stenosis that is not ductal dependent with no significant stenosis or suprasystemic RV pressures or cyanosis, balloon valvotomy is usually delayed beyond the neonatal period.
Careful 2-D echocardiographic and Doppler assessment of these patients prior to any interventions is of the utmost importance. Echo evaluation should include assessment of the RV anatomy, tricuspid valve annulus z-score, and measurements of the pulmonary valve annulus in preparation for catheter-based therapy. Balloon valvuloplasty procedures for critical or severe pulmonary stenosis in the neonate are performed in the cardiac catheterization laboratory usually with the patient intubated and sedated or under general anesthesia. A femoral venous approach is typically utilized, although this procedure has also been performed using umbilical venous access in very small neonates. After hemodynamic assessment, a right ventricular angiogram is performed to assess the size of the right ventricular cavity, the outflow tract, and the pulmonary valve annulus. The valve leaflets and annulus are best demonstrated on a straight lateral projection. This projection is also used for determining the annular diameter. An end-hole catheter is positioned with its tip in the RV outflow tract and a floppy-tipped torque wire is used to cross the stenotic orifice of the valve. When the ductus is patent, the wire is often able to be maneuvered across it into the descending aorta for more stable wire (and balloon) position during the procedure. A balloon diameter to valve annulus diameter (balloon-annulus) ratio of 1:1.2 is used for pulmonary valvuloplasty. The balloon is inflated and deflated rapidly after having been positioned over the wire and across the valve. Inflation times are typically less than 10 seconds. During inflation, a waist is seen in the mid-portion of the balloon at the level of the stenotic valve, and this waist disappears with full inflation of the balloon. The balloon is removed over the wire and follow-up hemodynamic data are obtained to reassess the pulmonary valve gradient. With a PDA, the valve gradient may not accurately quantify the valve gradient that may persist. If the right ventricular pressure is still suprasystemic, valvuloplasty may be repeated using a larger diameter balloon as long as a safe and appropriate balloon-annulus ratio is used. At the end of the procedure, a final right ventricular angiogram is performed to assess for any damage to the RV, tricuspid valve apparatus, and/or pulmonary artery and to assess the location of any residual obstruction.
Following successful balloon valvuloplasty, most patients will have sufficient antegrade pulmonary blood flow to tolerate discontinuation of the PGE-1 infusion and subsequent closure of the ductus. However, many will continue to have predominant right-to-left shunting at the atrial level until the right ventricular compliance improves. In some cases, this may take 3 to 4 weeks with multiple attempts of trialing off and restarting PGE-1 infusion for systemic saturations that drop consistently to or below the 75% to 80% range due to decreased ductal-level pulmonary blood flow in conjunction with a restricting or closing ductus. Most successful balloon valvuloplasty procedures result in some amount of pulmonary insufficiency. This is well tolerated in most infants and some have reported a beneficial effect of pulmonary insufficiency promoting right ventricular growth. With regression of the right ventricular hypertrophy, improvement of the ventricular compliance, and growth of the RV, the amount of right-to-left shunting at the atrial level decreases over time; adequate saturations are achieved and patients are able to be maintained off PGE-1 infusion. Saturations continue to increase as these right ventricular changes continue or with spontaneous closure of the PFO. A small percentage of infants with critical pulmonary stenosis who have undergone a successful balloon valvuloplasty procedure may still be unable to maintain adequate pulmonary blood flow and systemic saturations off PGE-1 infusion greater than 4 weeks following valve dilation. In the past, these patients have undergone surgical placement of a Blalock-Taussig shunt to augment pulmonary blood flow. Some centers have gone on to perform ductal stenting in the catheterization laboratory to maintain ductal patency and augmented pulmonary blood flow off PGE-1 infusion in these patients. This is more commonly the case in patients with membranous pulmonary valve atresia following perforation of the valve with subsequent balloon valvuloplasty (see later).
Holzer and colleagues recently reported using a multicenter registry on 304 balloon pulmonary valvuloplasty procedures performed in eight institutions over a 34-month period. The median age at intervention was 2 months with 39% of procedures performed in the first month of postnatal life. Procedural success was achieved in 82% of all procedures, being defined as either a reduction in peak systolic valvar gradient to less than 25 mm Hg or greater than 50% reduction of the valve gradient or reduction of right ventricular systolic pressure ratio by 50%. There was a trend toward lower procedural success with moderate to severe thickening of the pulmonary valve (72%). Minor adverse events were documented in 10% of procedures, while moderate, major, or catastrophic adverse events were documented in 2% of procedures. However, there were no deaths.
Pulmonary valvotomy can also be used in patients with other congenital lesions to improve pulmonary blood flow. This can be applied as a palliative procedure, for example, in patients with tetralogy of Fallot or other forms of RV outflow tract obstruction with severe cyanosis and hypoplastic pulmonary arteries that preclude early repair. In these cases, stenting the RV outflow tract is usually necessary. A recent report by McGovern et al. demonstrated successful results with alleviation of RV outflow tract obstruction and resolution of hypercyanotic spells, allowing growth of the pulmonary arteries and successful repair at a later stage without the need for a systemic-to-pulmonary shunt.
Perforation of the Pulmonary Valve in Pulmonary Atresia and Intact Ventricular Septum
Pulmonary atresia (PA) with IVS is a complex disorder that is not only characterized by membranous or muscular atresia of the right ventricular outflow tract (RVOT) but also significant heterogeneity of the right ventricular morphology. The coronary artery anatomy also may be affected in this disorder with in utero development of connections between the RV and the subepicardial coronary arteries (RV to coronary artery sinusoids or fistulas), predisposing these patients to abnormal coronary circulations. Prognosis is related to the severity of the coronary circulation abnormalities as well as to tricuspid valve anatomy and function. Prior to transcatheter or surgical intervention, careful assessment of the right heart and coronary anatomy must take place. Newborns with this disorder are obviously dependent on ductal flow and therefore PGE-1 infusion is initiated immediately after birth. TTE is performed for initial assessment. If it is clear that the tricuspid valve and/or RV are severely hypoplastic and that biventricular repair will not be an option, then plans for a palliative surgical shunt are made. In these patients, assessment of the atrial septum to determine adequacy of the atrial communication is made as restriction at the atrial level may lead to poor cardiac output and BAS should be considered. Cardiac catheterization with angiography to assess the coronary artery circulation is usually still performed in these patients, even when there is no plan for right ventricular decompression as patients with severe coronary artery abnormalities may be ultimately managed with cardiac transplantation. In patients where the echocardiographic assessment of right heart adequacy is less clear and/or when relief of the RVOT is considered, cardiac catheterization and angiography are performed to help characterize the size of the right ventricular cavity and outflow tract as well as to define coronary artery anatomy and determine whether coronary circulation is dependent on high right ventricular pressures (right ventricular dependent coronary artery circulation; RVDCC). In patients with RVDCC, relief of the RVOT may result in a catastrophic event due to inadequate perfusion of the myocardium with a sudden drop in the systolic pressure of the RV (i.e., coronary perfusion pressure in patients with RVDCC), resulting in myocardial ischemia, infarct, or death. If RVDCC is not present, then the decision to perform transcatheter relief of the RVOT with or without ductal stenting or surgical relief with placement of a systemic-to-pulmonary shunt is entertained.
It is very important that procedures involving perforation of the membranous valve be performed using a high-quality, biplane x-ray imaging system. Infants should be intubated and sedated and, if necessary, paralyzed so that they do not move during the procedure. Historically, perforation of the atretic pulmonary valve was performed using the stiff end of a guidewire. However, the use of a stiff wire tip in the RVOT of a neonate was often associated with significant and severe adverse events, such as perforating the anterior part of the outflow tract, bleeding, the need for emergent surgical procedures, and death. Laser energy was the first energy source to be used for valvar perforations. Unfortunately, the laser beam would perforate any tissue in its path beyond and/or adjacent to the valve as the depth of laser penetration is difficult to control. RF energy is less powerful than laser energy, but is easier to control and has more recently been used to perforate atretic valve tissue.
The development of a small, flexible coaxial catheter and wire system (Baylis Medical) that could be advanced within small (4-French) catheters positioned with the tip in the RVOT just beneath the atretic pulmonary valve made it possible to deliver RF energy that was confined to the tip of the wire at the membranous valve for perforation. It is very important to clearly define the anatomy of the RVOT as well as the main pulmonary artery by angiography in preparation for this procedure. Operators often use permanent thoracic landmarks and image “roadmaps” in an attempt to avoid inadvertently perforating outside the boundaries of the RVOT. Some advocate placing a retrograde catheter from the aorta across the ductus with its tip in the main pulmonary artery just above the atretic valve for something to aim at. Others have described passing a snare wire (Amplatz Gooseneck; Microvena, Vadnais Heights, Minnesota) within the retrograde catheter and opening it within the main pulmonary artery to act as a “target” as well as to delineate the boundaries of the pulmonary artery lumen ( Fig. 32.2A ). Only 5 to 10 W of energy applied for 2 seconds is needed to perforate the tissue. Once perforation has been performed, the wire and coaxial catheter are advanced into the main pulmonary artery. The RF wire can then be exchanged for a floppy-tipped guidewire that can be maneuvered across the ductus into the descending aorta. The snare may also be used to secure the wire tip in the descending aorta and provide maximum wire stability for tracking balloon catheters over it during subsequent valvuloplasty (see Fig. 32.2B ). As described in patients with critical pulmonary stenosis, serial dilations with incremental balloon diameters may be necessary for successful valvuloplasty. Postprocedure angiography is performed to assess for any injury of the tricuspid valve apparatus, RV outflow tract, main pulmonary artery, or ductus. Due to the nature of the atretic valve, free pulmonary insufficiency is present following a successful procedure. Also, at this point, consideration of whether pulmonary blood flow will require augmentation by either ductal stenting or surgical shunt placement is made, although many centers may observe patients clinically following valvuloplasty and before implanting a stent or placing a shunt. It is possible that, if the RV is only mildly hypoplastic and if the tricuspid valve function is normal, adequate pulmonary blood flow will have been established by the perforation or valvuloplasty procedure and that the infant may tolerate cessation of the PGE-1 infusion and closure of the ductus without the need for further catheter-based or surgical procedures.

Success of this procedure has been reported by many centers. Complications are similar to those associated with pulmonary balloon valvuloplasty for critical pulmonary valve stenosis. Agnoletti and colleagues reported the long-term results in 33 newborns who underwent successful perforation or valvuloplasty procedures for PA or IVS. Of these patients, 50% needed surgery in the neonatal period while an additional three patients had elective surgery beyond the neonatal period for augmentation of pulmonary blood flow.
Schwartz et al. reported on the outcomes and predictors of reintervention in these patients after RF perforation and balloon pulmonary valvuloplasty in 23 patients. All procedures were successful with no major complications and 29% of patients did not require subsequent interventions, 9% required subsequent balloon dilation of the pulmonary valve, and 20% received an additional blood supply for the pulmonary circulation (Blalock-Taussing shunt or PDA stent), while 42% required surgical RVOT augmentation. They found that post-valvuloplasty pulmonary valve gradient was predictive of the subsequent need for RVOT intervention.
More recently, some centers have adopted a hybrid approach for these patients involving perventricular pulmonary valve perforation. Early results showed that this can be a safe and feasible alternative to the surgical approach with the advantages of avoiding cardiopulmonary bypass in the neonatal period, direct access to the RVOT, and lower risk for complications and perforation.
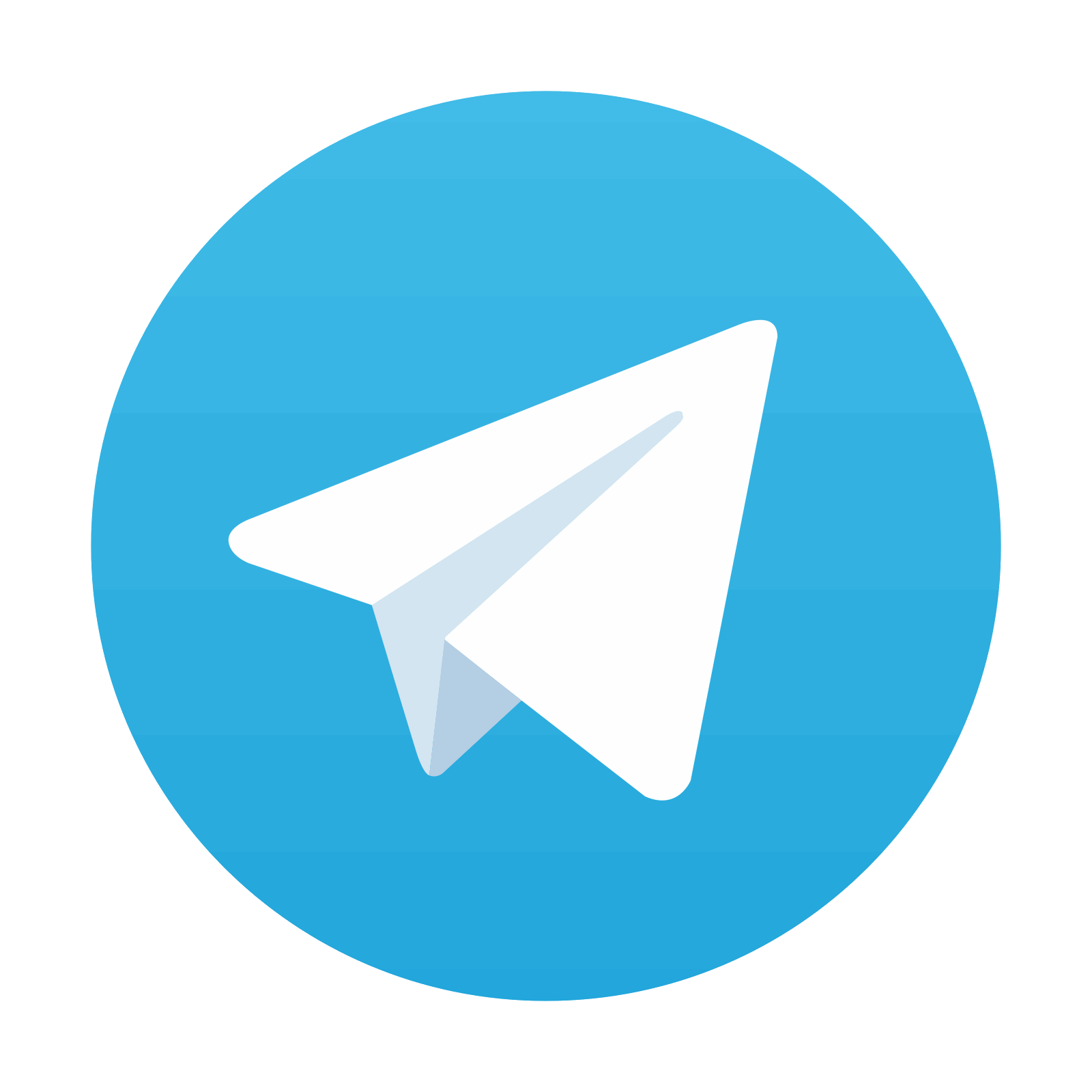
Stay updated, free articles. Join our Telegram channel

Full access? Get Clinical Tree
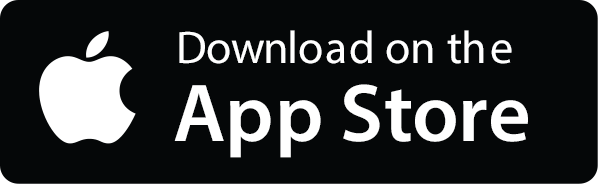
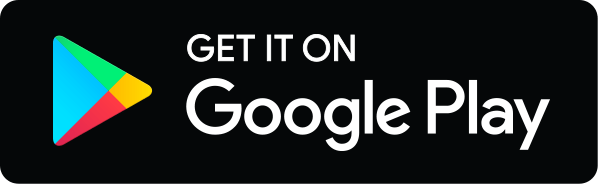