Fig. 38.1
Compression and decompression phases of cardiopulmonary resuscitation
It is critical to understand that blood flow to the vital organs is severely reduced during standard CPR, even under the best of circumstances [3, 4]. During standard CPR, chest compression results in an elevation of intrathoracic pressure and indirect cardiac compression. Both of these mechanisms result in forward blood flow out of the chest to perfuse the brain and other vital organs. However, the effectiveness of standard CPR is largely determined by the amount of blood returned to the chest to refill the heart after each compression phase. This process is highly dependent on the degree of chest wall recoil. When the chest recoils, intrathoracic pressures fall relative to extrathoracic pressures, and venous blood returns to the right heart.
38.3 The Impedance Threshold Device for Cardiac Arrest
Standard CPR by itself has been shown to be inherently inefficient, in large part due to the lack of adequate blood return to the thorax during the chest wall recoil phase [3, 4]. Moreover, the coronary perfusion pressure (CPP) , a critical determinant of CPR efficacy, is only marginally adequate as the pressure gradient between the aorta, the right atrium, and left ventricle is far from optimal. During the decompression (or passive relaxation) phase of standard CPR, a small decrease in intrathoracic pressure (relative to atmospheric pressure) develops, which promotes blood flow back to the heart. Myocardial perfusion predominantly occurs during this decompression phase. Uniquely, the impedance threshold device (ITD) (ResQPOD®, Advanced Circulatory, Roseville, MN, USA) was designed to improve venous return to the heart during the decompression phase of CPR [3–9]. In so doing, the ITD increases the CPP during CPR, enhancing delivery of oxygenated blood to both the heart and brain. The ITD shown in Fig. 38.2 works effectively with both a face mask and an advanced airway (e.g., endotracheal tube, supraglottic airway).
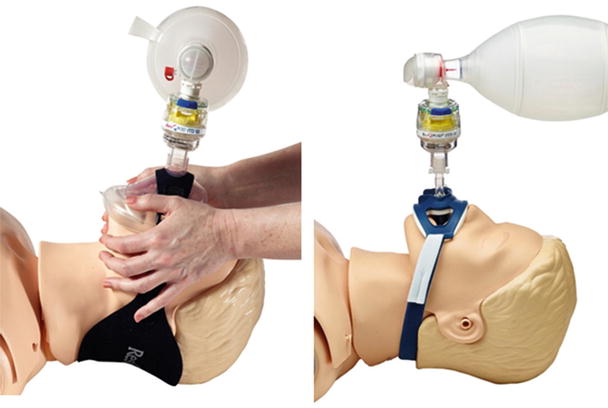
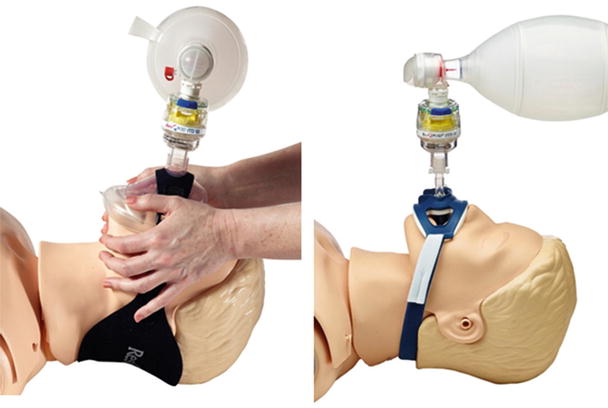
Fig. 38.2
ResQPOD® impedance threshold device on a face mask and endotracheal tube
The concept underlying the ITD was first discovered when measuring intrathoracic pressures in patients undergoing a new type of CPR, active compression-decompression (ACD) CPR [10]. It was found that if the endotracheal tube was transiently occluded during the active decompression phase, intrathoracic pressures became markedly more negative. This led to the idea of transiently blocking the airway or impeding inspiratory gas exchange during the chest wall decompression phase of CPR to create a greater pressure differential between the thorax and the rest of the body, thereby enhancing blood flow back into the thorax. As such, the ITD harnesses the kinetic energy of the chest wall recoil, thereby augmenting the “bellows-like” action of the chest with each compression-decompression cycle [8, 9]. The ITD contains pressure-sensitive valves that selectively impede the influx of inspiratory gas during chest wall decompression, thereby augmenting the amplitude and duration of the vacuum within the thorax. This vacuum draws more venous blood back into the heart, resulting in increased cardiac preload, followed by improved cardiac output and vital organ perfusion. During chest compression , the one-way valve is open and does not cause any resistance to exhalation. When the resuscitation bag is squeezed for active ventilation, the one-way valve remains open and does not cause any resistance to gas exchange. In this manner, the ITD functions to lower intrathoracic pressure only during the decompression phase of CPR without compromising patient ventilation. The ITD consists of a valve body, a one-way pressure-sensitive silicone valve, and a safety check valve [5]. When a spontaneous pulse returns, the ITD is removed from the respiratory circuit. The safety check valve serves as a precautionary measure to prevent negative pressure pulmonary edema and potential barotrauma and to enable the patient to breathe if there is a return of spontaneous ventilation and the ITD has not been removed.
Positive data from animal studies and clinical trials, described in detail below, formed the basis for the American Heart Association’s Level IIb recommendations for the use of ITDs in the 2010 American Heart Association (AHA) guidelines . Today, the ResQPOD® is sold in the United States as a device that can be used to increase circulation in patients with low blood pressure, including patients in cardiac arrest. Further, Aufderheide et al. reported outcomes from the use of the ITD in seven EMS systems in the United States. They showed that the changes in CPR practice, which now emphasize more compressions and fewer ventilations, complete chest wall recoil, uninterrupted chest compressions during advanced airway management, and the use of the ITD during basic life support (BLS) and advanced life support resulted in a doubling of hospital discharge rates for all patients, regardless of presenting heart rhythm, i.e., from 8 to 16 % [11]. In addition, the neurological outcomes were similar between groups, and patients who had an initial rhythm of ventricular fibrillation had a hospital discharge rate of 28.1 % compared with 17.2 % in the historical control group.
One of the first animal studies performed with the ITD demonstrated that the use of the active ITD increased 24-h survival and preserved neurological function after cardiac arrest in swine [8]. There was a statistically significant increase in both of these key outcome parameters, as shown in Fig. 38.3. The improved neurological function was observed in the overall study group, as well as in the subset of animals that were resuscitated with defibrillation shock therapy and epinephrine. Blood gas data demonstrated that oxygenation was adequate in both groups and no differences were observed between groups on autopsy. The intrathoracic pressures were significantly lower in the ITD group. Subsequent studies have demonstrated that the use of the ITD also lowers intracranial pressures (ICPs ) more rapidly than in animals treated with standard CPR alone. It is hypothesized that these observations help to explain the markedly improved neurological outcomes in this porcine survival study [8].
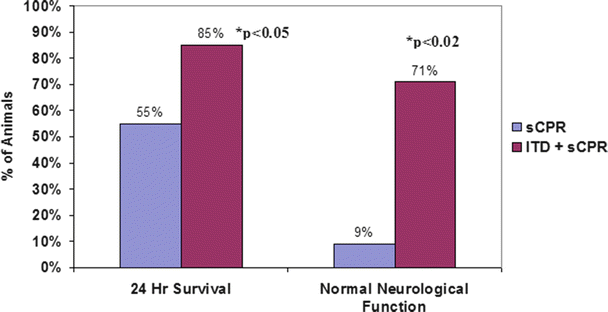
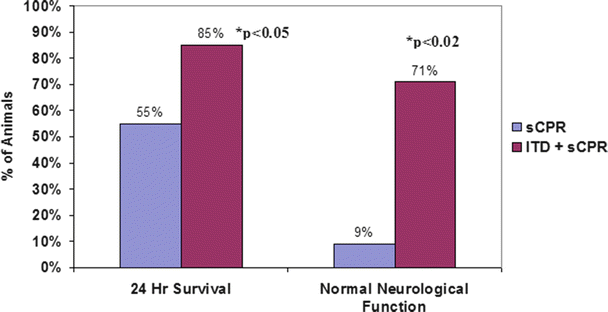
Fig. 38.3
Percentage of animals with 24-h survival and normal neurological function comparing standard CPR (sCPR) to impedance threshold device (ITD) + standard CPR after cardiac arrest in pigs
Importantly, the first randomized double-blinded prospective clinical trial showed that the use of the ITD during standard CPR resulted in an increase in systolic blood pressure. However, in that study nearly all of the patients also received excessively high ventilation rates. Follow-up animal studies demonstrated that excessive ventilation (termed hyperventilation ) during cardiac arrest actually inhibited venous return, compromised hemodynamics, and resulted in increased mortality rates [12, 13]. In addition, frequent incomplete decompression of the chest was also witnessed and recorded in that study. Follow-up studies in animals demonstrated that incomplete chest wall recoil resulted in positive intrathoracic pressures, which in turn decreased venous return and caused markedly poorer cardiac and cerebral blood flows; incomplete decompression is thus a key component in the deficiency of standard CPR [14]. These observations also demonstrate how important the cardiopulmonary cerebral interactions are during CPR.
In the first US double-blinded randomized survival study of the ITD, there was nearly a threefold increase in 1-h and 24-h survival rates in patients with an initial rhythm of PEA when comparing treatment with a sham versus active ITD. Figure 38.4 shows the relative percent survival in all subjects initially presenting with PEA. It is important to emphasize that the results from this study were observed in the setting of real-world CPR, prior to the use of tools to help control ventilation frequency or the adequacy of compression rates, depths, and complete chest wall decompressions. Figure 38.5 shows the percent survival in all subjects presenting with various types of cardiac rhythms.
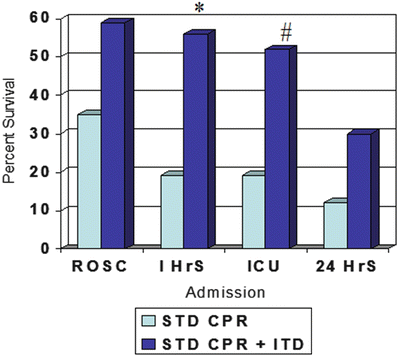
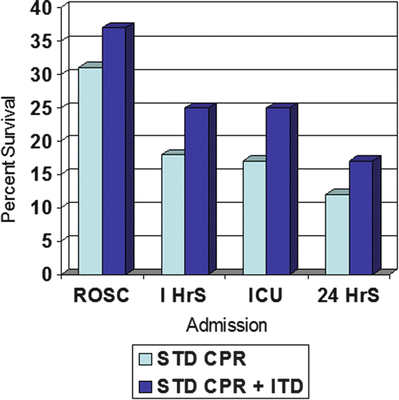
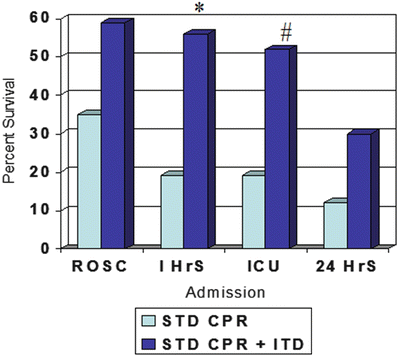
Fig. 38.4
Outcomes for all subjects initially presenting with pulseless electrical activity. *p = 0.01, #p = 0.02. ROSC return of spontaneous circulation, 1 HrS 1-hour survival; ICU ICU admission rate, 24 HrS 24-hour survival
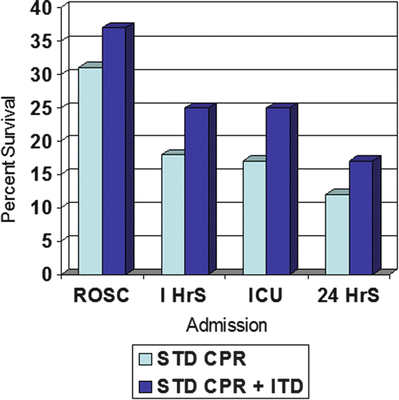
Fig. 38.5
Outcomes for all subjects presenting in all rhythms. ROSC return of spontaneous circulation, 1 HrS 1-hour survival, ICU ICU admission rate, 24 HrS 24-hour survival
The benefits of the ITD in patients with PEA can be better understood by examining the hemodynamic tracings of one of the substudy group patients, as shown in Fig. 38.6. When CPR was discontinued, there was a persistent regular electrical activity, but the aortic pressures were inadequate to generate a palpable pulse. This example of PEA demonstrates that although there were small rises in the arterial pressure with each cardiac contraction, this was insufficient to cause a palpable pulse or effective vital organ blood flows. Importantly, without effective vital organ perfusion, return of spontaneous circulation (ROSC ) is not possible. We speculate that the known increase in circulation and blood pressures associated with the use of the ITD, in both animals and humans, is enough to increase the aortic systolic and diastolic pressures to a sufficient level to allow for effective vital organ perfusion. It should be noted that the number of patients in VF treated with the ITD in that study was too low to definitively determine if the ITD would benefit this patient subgroup. Nonetheless, hospital discharge rates were 6 % in the control group and 14 % with the active ITD in that study.
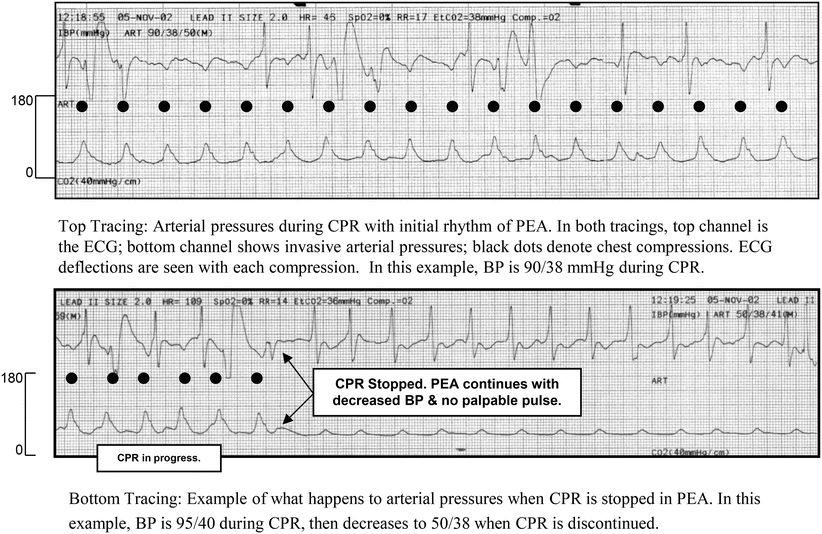
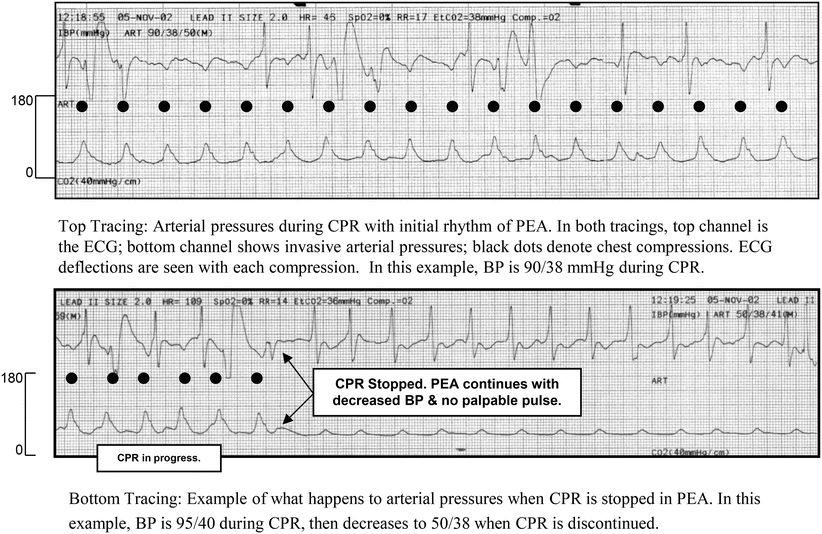
Fig. 38.6
Hemodynamic tracing of a patient with pulseless electrical activity (PEA). BP blood pressure, CPR cardiopulmonary resuscitation
One of the most important findings from the first clinical study when the ITD was used with standard CPR was that the overall quality of administered CPR was very poor. Based on the hemodynamic substudy data collected in this clinical trial, hyperventilation was recorded in 100 % of cases, and incomplete chest wall recoil was recorded in approximately 50 % of cases. These critical findings demonstrated the challenges of performing standard CPR with a pair of human hands alone, and this resulted in a change in the initial design of the ITD; a timing light was added that flashes at 10 times per minute to provide the rescuer with guidance on when to ventilate the patient and how to assure that compressions are performed 100 times per minute (compress 10 times per light flash). In the future, the use of the ITD will be even more effective once improved standard CPR is practiced and performed uniformly.
It is also important to emphasize that one of the very exciting recent advances in CPR research has been the rediscovered benefit of therapeutic hypothermia after successful resuscitation. Several studies have demonstrated a 50 % increase in long-term survival rate and improved neurological function in survivors of cardiac arrest with VF who received therapeutic hypothermia in the hospital shortly after resuscitation [15–17]. Therefore, when hypothermia is applied to patients resuscitated after PEA, there should be a similar increase in long-term survival rate with good neurological function. Fortunately, a growing number of patients are now routinely treated with therapeutic hypothermia after successful resuscitation.
38.4 Effects of Incomplete Chest Wall Recoil and Hyperventilation on the Quality of Standard CPR
The AHA recognized the inefficiencies of standard CPR in 2000, 2005, and 2010 when they issued new guidelines for performing CPR. The latest guideline reinforces the importance of the chest decompression phase in teaching CPR: Rescuers should allow complete recoil of the chest after each compression, to allow the heart to fill completely before the next compression [18].
As mentioned previously, Aufderheide et al. observed rescuers frequently leaning on the chest during the decompression phase, thereby maintaining some residual and continuous pressure on the chest wall during the decompression phase of CPR. This prevented complete chest wall recoil. More specifically, airway pressures were consistently measured as positive during the decompression phase (>0 mmHg) in 6/13 (46 %) of consecutive adults. This was caused by incomplete chest wall recoil alone or combined with prolonged, positive ventilations. With standard CPR and incomplete chest wall recoil, insufficient intrathoracic vacuum pressures are achieved. In contradistinction, when active compression and decompression are performed in conjunction with the use of an ITD, a significant intrathoracic vacuum results.
In 2005, Yannopoulos et al. conducted an animal study to address the question of the physiological impact of incomplete chest wall recoil [14]. Nine pigs in VF for 6 min were treated with an automated CPR device with compressions at 100/min, a compression depth of 25 % of the anteroposterior diameter, and a compression to ventilation ratio of 15:2. After complete (100 %) chest wall decompression for 3 min during standard CPR, the decompression depth was reduced to 75 % of complete decompression for one minute of CPR and then restored for another one min of CPR to 100 % decompression. CPP was calculated as the diastolic aortic—right atrial (RA) pressure. Cerebral perfusion pressure (CePP) was calculated by measuring the area between the aortic pressure curves and the ICP curves. Figure 38.7 summarizes the results of this study including the differences in aortic systolic pressure, CPP, intratracheal pressure (ITP), and CePP. With 100 %–75 %–100 % chest wall recoil, the CPP was 24.2 ± 2.0, 15.0 ± 1.2, and 15.6 ± 1.3 mmHg (p < 0.05); CePP was 320 ± 120, 95 ± 15, and 150 ± 30 mmHg min (p < 0.05); diastolic aortic pressure was 26.8 ± 2.8, 18.9 ± 2.3, and 18.2 ± 2.1 mmHg (p < 0.05); ICP during decompression was 18.1 ± 2.8, 21.6 ± 2.3, and 17.4 ± 2.6 mmHg (p < 0.05); RA diastolic pressure was 2.7 ± 1.9, 3.9 ± 1.9, and 2.7 ± 1.6 mmHg (p < 0.05); and mean arterial pressure (MAP) was 41.4 ± 2.8, 32.5 ± 2.2, and 36.6 ± 1.9 mmHg (p < 0.05). The CPP and CePP never fully recovered after treatment with the 75 % incomplete chest wall decompression. It is striking that a small reduction of chest wall recoil (1 cm), which is a common occurrence during the performance of CPR, resulted in such a marked reduction in cerebral and CPPs.
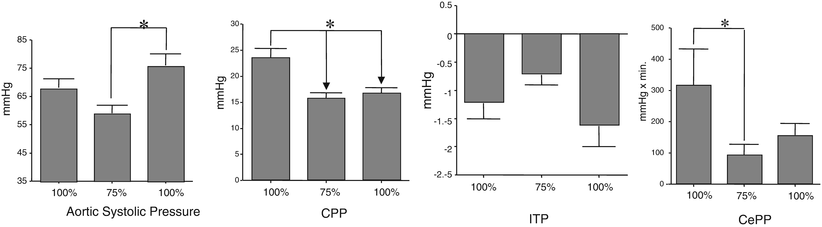
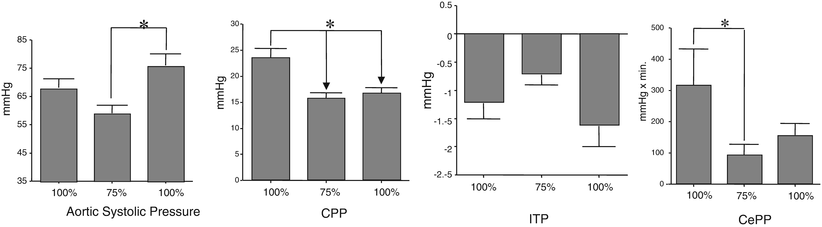
Fig. 38.7
Aortic pressures, coronary perfusion pressure (CPP) , intrathoracic pressure (ITP ), and cerebral perfusion pressure (CePP) decreases when complete decompression is not allowed (75 %). *p < 0.05
The effect of incomplete chest wall recoil on reducing cerebral perfusion can be seen graphically in Figs. 38.8 and 38.9 [14]. Figure 38.8a represents, condensed in time, the sequential 100 % chest recoil, 75 % chest wall recoil, and return to 100 % chest wall recoil. Tracings of ITP, aortic pressure (AoPr), and ICP with 200 ms per division are indicated with arrows. Piston throw (in cm) is also shown to sequentially demonstrate the complete (100 %) chest wall recoil (38.8b), 75 % chest wall recoil (38.8c), and return to complete chest wall recoil (38.8d). The positive area between the AoPr and ICP tracing represents cerebral perfusion (marked as black). Note how the area decreases, especially during decompression with incomplete chest wall recoil (75 %) and that it partially recovers when full recoil was restored. Figure 38.9 shows the effect of positive pressure ventilation on CePP. The first tracing shows the aortic and ICP waveforms with full chest wall recoil after a ventilation cycle, while the second tracing shows the aortic and ICP waveforms with incomplete chest wall recoil after a ventilation cycle. Positive pressure gradient (Ao-ICP) is colored black. Note the marked difference in total area during each compression-decompression cycle with and without a positive pressure breath. The bar graphic shows the mean 4-beat area of all animals during and after a ventilation cycle. The mean ± SEM values during 100 and 75 % decompression have been graphed. During positive pressure ventilation, ICP rises and the positive gradient disappears. There was effectively no blood flow to the brain (Fig. 38.9, second panel). This study demonstrated that incomplete decompression has significant deleterious effects on both CPP and CePP. The residual positive intrathoracic pressure during the decompression phase associated with incomplete chest wall recoil decreased forward blood flow, impeded venous return, increased ICP, and undermined the efficiency of CPR. These recent animal studies underscore the fundamental hemodynamic importance of complete chest wall decompression during CPR. Whether rescuers can be retrained to allow for complete chest wall decompression during standard CPR remains an important issue.
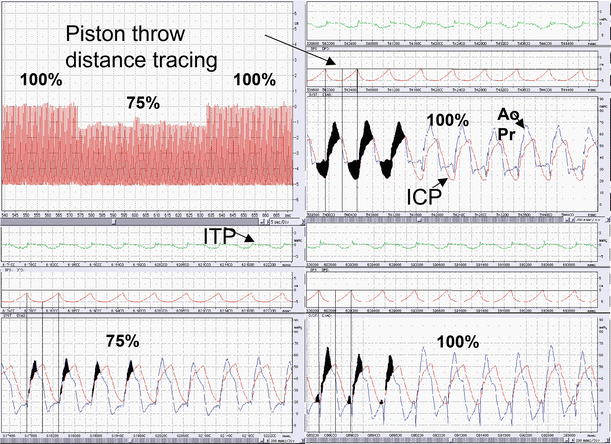
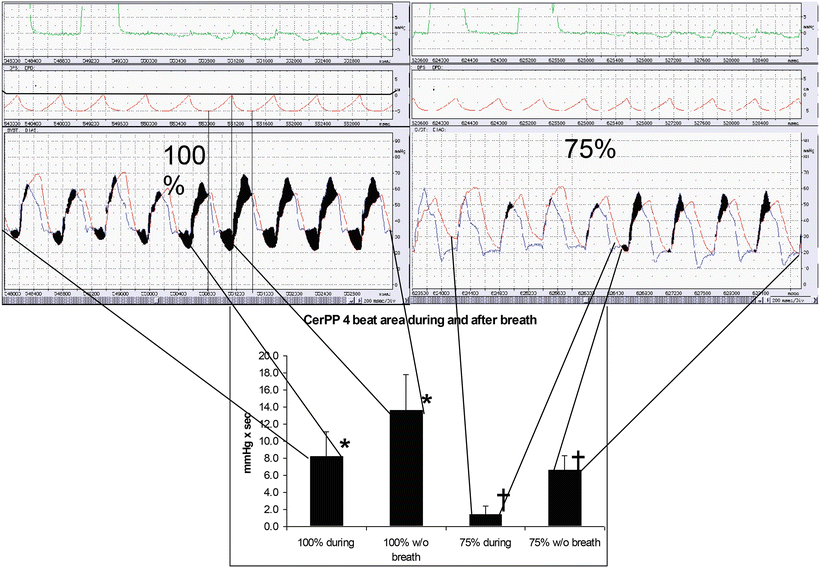
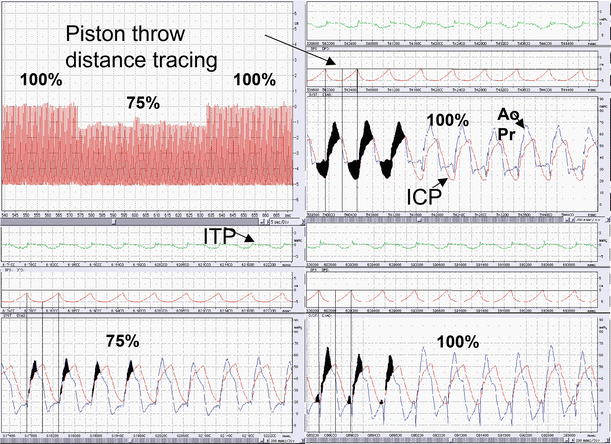
Fig. 38.8
Effect of incomplete chest wall recoil on reducing cerebral perfusion (see text for details). AoPR aortic pressure, ICP intracranial pressure, ITP intrathoracic pressure, blue tracings = aortic pressure, pink tracings = intracranial pressures
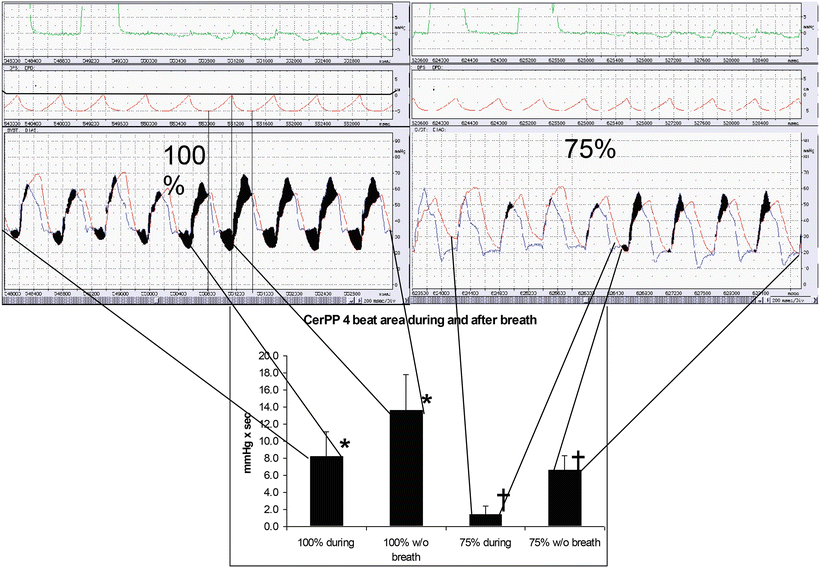
Fig. 38.9
Effect of incomplete chest wall recoil on reducing cerebral perfusion (see text for details). CerPP cerebral perfusion pressure
A change in CPR technique to allow for the palm of the compressing hand to lift off the chest at the end of decompression may be important to assure full chest wall recoil during standard CPR. Accordingly, Aufderheide et al. implemented a randomized prospective clinical trial using an independent group of 30 actively practicing and certified EMS providers, not aware of the ongoing trial, in a controlled setting using a recording CPR manikin. The purpose of the study was to evaluate three alternative CPR techniques to determine if they would improve complete chest wall recoil compared with standard CPR while maintaining adequate compression depth and proper hand position placement. The three alternative CPR techniques were: (1) two-finger fulcrum technique (lifting the heel of the hand slightly but completely off the chest during the decompression phase of CPR while using the thumb and little finger as a fulcrum), (2) five-finger fulcrum technique (lifting the heel of the hand slightly but completely off the chest during the decompression phase of CPR, using all five fingers as a fulcrum), and (3) hands-off technique (lifting the heel and all fingers of the hand slightly but completely off the chest during the decompression phase of CPR).
In this study, during standard CPR using the traditionally taught hand position (standard hand position), complete chest wall decompression was recorded in only 16.3 % of all compression-decompression cycles, adequate depth of compression in 48.5 %, and acceptable hand placement in 85.0% of compression-decompression cycles. When compared with standard CPR, the hands-off technique achieved the highest rate of complete chest wall recoil (95.0 % versus 16.3 %, P < 0.0001) and was 129 times more likely to provide complete chest wall recoil (OR: 129.0; CI: 43.4–382.0) [19]. There were no significant differences in the accuracy of hand placement, depth of compression, or reported increase in fatigue or discomfort with its use compared with the standard hand position. The hands-off technique was easily learned and applied by participating EMTs, because it uses the same hand configuration as is currently recommended by the AHA.
38.5 Optimizing Outcomes with Standard CPR and the Impedance Threshold Device
Aufderheide and colleagues have recently analyzed and applied the combined lessons learned from the initial clinical trials of the ITD and standard CPR . They analyzed data from seven EMS systems that serve a population of more than 3 million patients and reported that, when CPR is performed correctly with the ITD and the mistakes described above are reduced or eliminated by rigorous training and correct ITD used, survival rates increased from 7.9 to 15.7 % for all patients who presented with cardiac arrest; survival rates for those with an initial rhythm of VF increased from 17 to 28 % [11]. Therapeutic hypothermia was not yet in use in these EMS systems and their associated hospitals when these data were obtained. Similar benefits from performing CPR according to the AHA 2005 and 2010 guidelines and the use of the ITD have also been reported for patients in in-hospital cardiac arrest. For example, Thigpen reported that hospital discharge rates in one large Mississippi hospital increased from 17 to 28 % with the administration of this new resuscitation approach [20, 21]. Similar data from St. Cloud Hospital in Minnesota are shown in Fig. 38.10; data from before the change in practice in July 2006 were compared to data after implementation of the new CPR techniques and the ITD. Importantly, the survival rates after an in-hospital cardiac arrest nearly doubled.
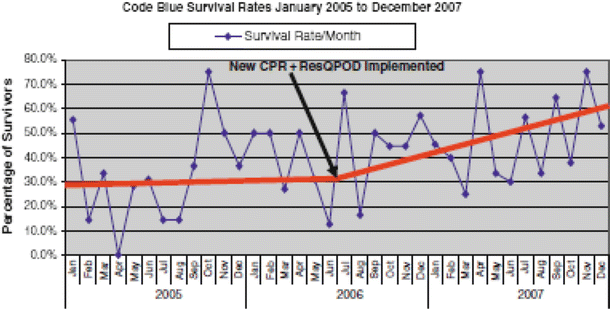
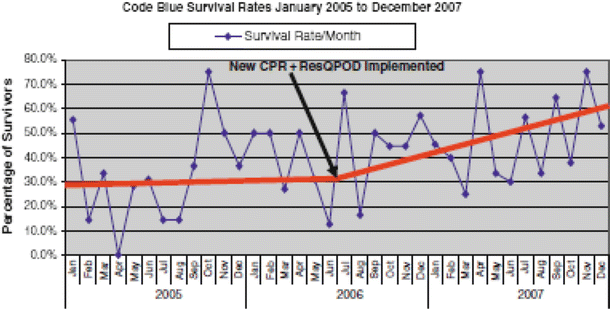
Fig. 38.10
Code blue survival rates in St. Cloud, Minnesota (January 2005 to December 2007). CPR cardiopulmonary resuscitation
A study by Lick et al. investigated the effects of stricter adherence to the AHA guidelines for CPR which included the recommended use of the ITD. The Take Heart America initiative was started in an attempt to increase survival from cardiac arrest by focusing on the implementation of the 2005 and 2010 AHA guidelines. It is centered not on a single treatment but rather on a bundle of care approach including community-wide initiatives, including: (1) increased cardiac arrest awareness, (2) increased bystander CPR rates, (3) promoting the use of automated external defibrillator, and (4) the administration of immediate high-quality CPR with the use of the ITD throughout the duration of the code. Further, additional in-hospital treatments such as therapeutic hypothermia, revascularization, and implantable cardiac defibrillators were also emphasized. Survival and outcomes data for patients receiving the bundled interventions were compared to control data prior to implementation of the initiative. Importantly, survival to hospital discharge increased significantly from 8.5 to 19.0 %. These differences were especially striking in the subset of patients who had VF as the initial arrest rhythm; their numbers increased from 17.0 % versus 41.0 % [22]. These results show that when focus is applied to the AHA guidelines including recommended use of the ITD, cardiac arrest survival rates can be greatly improved. In summary, with greater attention to enhancing circulation, based on the newly discovered mechanisms underlying circulation during CPR, significant progress has been made by simply using a pair of hands and the ITD.
It should be noted that a large multicenter study conducted by the Resuscitation Outcomes Consortium (ROC) group looked at outcomes from cardiac arrest using an active ITD versus a sham ITD. The results, originally published in 2011, reported no statistical differences in survival with good neurological function in the active group and the sham group [23]. Additional analyses revealed that the chest compression rates varied widely throughout the study, ranging from 50 compressions/minute to 240 compressions/minute [24]. When the subset of patients received compressions at a rate of 100 ± 10 compressions/minute, the active device showed a marked benefit on survival with good neurological function compared to the sham device. There was also a significant benefit identified for subjects who suffered VF arrests. This second look at the ROC data shows that when CPR is performed correctly, per AHA guidelines, the ITD can increase the rate of survival to hospital discharge with improved neurological function.
Another analysis of the ROC data was independently performed by Yannopoulos et al. [25]. In addition to investigating compression rates as a surrogate for quality CPR, they also included only subjects receiving CPR at a depth of 4–6 cm (AHA recommends depth of 2 in or 5 cm) and a compression fraction (% of time performing chest compressions in a given minute) of >50 %. When these filters were applied, 7.2 % of subjects receiving CPR with the active ITD survived to hospital discharge with good neurological function, while the rate was only 4.1 % for subjects receiving CPR with the sham ITD (p = 0.006). This represents a 43 % increase in survival rate with good neurological function with the active ITD, over the sham ITD. Again, when quality CPR is delivered according to guidelines, the use of an ITD provides benefits to patients.
38.6 Active Compression-Decompression CPR
It has been shown that despite training, it is difficult to perform standard manual CPR correctly, e.g., allowing for the chest to fully recoil following each compression. These problems result in significantly less blood flow back to the heart and reemphasize that perhaps another device is needed to correct this widespread problem. Correction of this basic flaw (incomplete chest wall recoil) through the use of a technique that ensures full chest wall recoil and user guidance has the potential to significantly improve the chance for survival after cardiac arrest. One such technique is ACD-CPR which is performed with an ACD-CPR device.
More specifically, ACD-CPR increases the naturally occurring negative intrathoracic pressure by physically lifting the chest wall and helping it to return to its resting decompressed position. During standard CPR, the chest wall’s natural elasticity will partially recoil from compression. Several factors can contribute to less than optimal recoil: (1) patient age, (2) brittle or broken ribs, (3) a separated or broken sternum, (4) a barrel-shaped chest, (5) the presence of chest concavity, and/or (6) the tendency for rescue personnel to lean on the chest and thus cause incomplete chest wall recoil during performance of CPR. The use of an ACD-CPR helps ensure that the chest re-expands to generate the negative intrathoracic pressure needed to allow passive filling of the heart.
For example, ACD-CPR can be performed with a handheld suction device (ResQPUMP®, Advanced Circulatory Systems) fixed on the anterior chest wall. During the compression phase, the chest is compressed, and blood is forced out of the heart to perfuse the vital organs, as with standard CPR. Next, when the chest is actively pulling up with the device, a vacuum is created within the thorax, drawing more blood back into the heart. This technique improves hemodynamics [26, 27] and, in some studies, long-term survival rates with patients in cardiac arrest, as compared with patients receiving standard CPR alone [28, 29]. The ACD-CPR device is currently being used in many countries throughout the world including France and Israel, as well as parts of China, Japan, and Germany (Fig. 38.11). ACD-CPR in combination with the ITD, known as the ResQCPRTM System, received regulatory clearance in May of 2015 from the Food and Drug Administration (FDA) with an indication for use as a CPR adjunct to improve the likelihood of survival in adult patients with non-traumatic cardiac arrest.
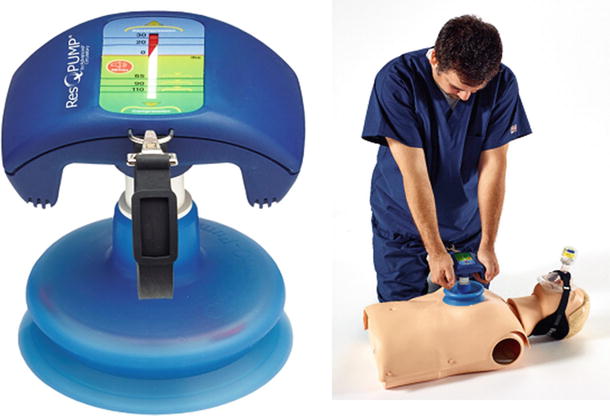
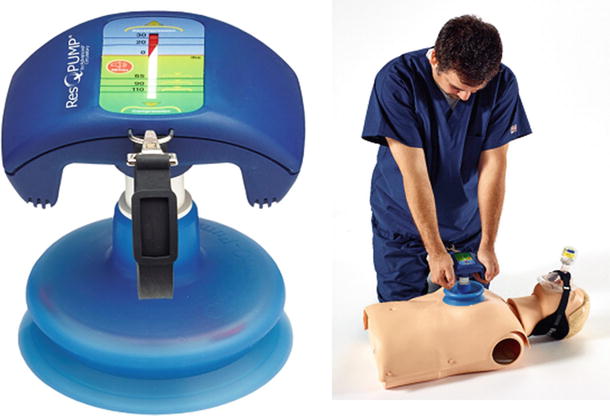
Fig. 38.11
ResQPUMP®, the US version of the active compression-decompression cardiopulmonary resuscitation device. The force gauge and metronome are used to guide the rescuer in the proper performance
It should also be noted that ACD-CPR + ITD has been evaluated in multiple animal and clinical trials and is currently recommended in the AHA guidelines as an alternative to standard CPR. Importantly, the device combination has been shown to quadruple blood flow to both the heart and brain, compared with manual standard CPR alone. This device combination also significantly increases blood pressures and survival rates [29–31].
Lurie et al. specifically studied the effects of ACD-CPR and an ITD on blood flow; the results are summarized in Fig. 38.12. Preclinical animal studies demonstrated that left ventricle and cerebral blood flows were markedly improved with ACD-CPR + ITD [10, 32]. In these studies, CPPs were >20 mmHg, the minimum CPP thresholds needed to optimize the chance for survival in both humans and in a porcine model of cardiac arrest [33, 34]. The device combination also optimized perfusion within the brain, which was found to be even greater than baseline levels after a prolonged arrest, when comparing standard CPR to the combination of ACD-CPR + ITD [10]. The investigators believe that one of the reasons that the clinical trials with ACD-CPR + ITD have been successful is secondary to the marked increases in cerebral perfusion that can be achieved with this new approach. These findings have been reproduced by several other investigators using both pediatric and adult pigs in cardiac arrest [4, 35]. Improved forward blood flow and vital organ perfusion with use of ACD-CPR + ITD also enhances drug efficacy during CPR [36]. For example, it was shown that the effects of exogenous vasopressin were significantly enhanced with ACD-CPR + ITD for hypothermic pigs, as reflected by higher coronary and CePPs and improved cerebral metabolic profiles.
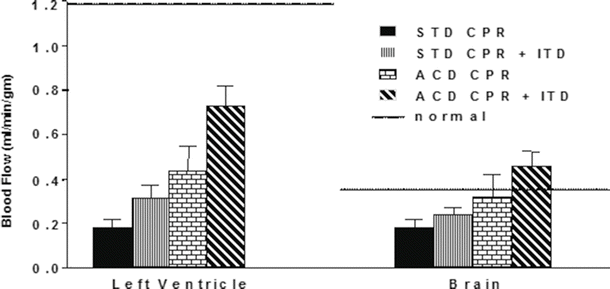
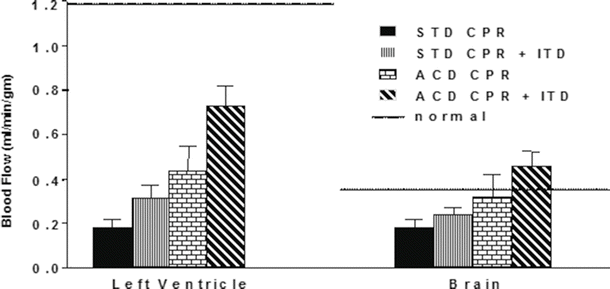
Fig. 38.12
Blood flow in a porcine model . The cumulative effect of ACD-CPR and ITD devices [9]. Solid horizontal line represents normal baseline values. STD standard, ACD active compression-decompression, CPR cardiopulmonary resuscitation, ITD impedance threshold device
In general, the use of the combination of ACD-CPR and the ITD can be considered to be synergistic. To date, four randomized clinical trials have been performed to evaluate the relative effectiveness and safety of the ACD-CPR + ITD in humans [30, 31, 37, 38]. The first blinded randomized clinical trial focused on resultant hemodynamics in patients with out-of-hospital cardiac arrest [37]. Eleven patients were treated with an active (functional) ITD and 10 with a sham (placebo) ITD. In that study, end-tidal carbon dioxide (ETCO2) levels rose more rapidly and reached higher levels with the active ITD; systolic and diastolic blood pressures were nearly normal in the active ITD group (109/57 mmHg) versus the sham ITD group (89/35 mmHg, P < 0.01). In addition, ROSC occurred more rapidly in the active ITD group compared with the sham ITD group. Based upon these data, the use of ACD-CPR + ITD was recommended as an alternative to standard CPR in the 2000 AHA guidelines [39].
Another study demonstrated that the ITD augments negative intrathoracic pressure when applied to a face mask [38]. This is important because it indicates that inspiratory impedance can be added during BLS airway management (by first responders and perhaps even lay rescuers prior to intubation). Patients with out-of-hospital cardiac arrest were randomized prior to endotracheal intubation to either a sham or active ITD, and intrathoracic pressure tracings were recorded. Addition of the active ITD to the face mask resulted in an immediate decrease in intrathoracic pressures during ACD-CPR. Each time the active ITD was used, there were significant reductions in the decompression phase intrathoracic pressures. These studies demonstrated, for the first time, the degree of negative intrathoracic pressures achieved with ACD-CPR + ITD in humans. The average maximum negative intrathoracic pressure was −7.3 mmHg with the active ITD on an endotracheal tube versus only −1.3 mmHg with the sham ITD. A second important finding was that it took up to 5 compression-decompression cycles to achieve the maximum negative intrathoracic pressures, as respiratory gases are expelled from the chest and prevented from reentry. This mechanism plays a key role in the function of the ITD. Each time an active, positive pressure, ventilation was delivered, the decompression phase intrathoracic vacuum was lost and required regeneration. Thus, the less frequently the ventilation rate was employed, the greater the blood flow back to the heart. A recent study using standard CPR with the ITD in pigs confirmed this important observation [12]. This has become an important theme for all types of CPR; ventilations interrupt CPP and should be reduced to the minimum required to maintain oxygenation and transpulmonary circulation.
It is generally accepted that ACD-CPR with an ITD improves short-term survival rates after cardiac arrest. A recent prospective controlled trial was performed in Mainz, Germany [37]; patients with out-of-hospital arrest of presumed cardiac etiology were sequentially randomized to ACD-CPR + ITD or standard CPR (control subjects) by the advanced life support team after intubation. Patients with an identified initial heart rhythm of VF (42 % of the total), who could not be resuscitated by BLS early defibrillation, were enrolled in this clinical trial, as well as patients with an initial rhythm of asystole or PEA. The primary endpoint was 1-h survival after a witnessed arrest. With ACD-CPR + ITD (n = 103), ROSC, 1-h and 24-h survival rates were 55 %, 51 %, and 37 % versus 37 %, 32 %, and 22 % for standard CPR alone (n = 107; p = 0.016, 0.006, and 0.033), respectively (shown in Fig. 38.13).
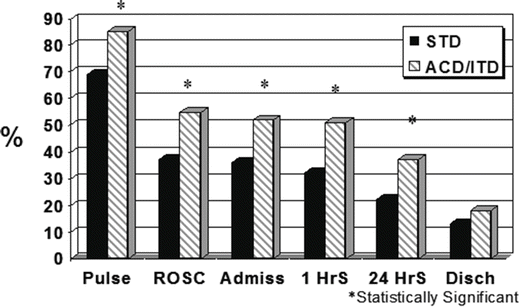
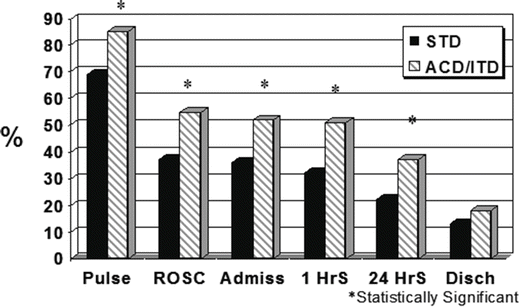
Fig. 38.13
Outcomes associated with comparison of standard cardiopulmonary resuscitation (STD) and ACD-CPR + ITD (n = 210) in Mainz, Germany. 1HrS 1-hour survival, 24HrS 24-hour survival, ACD active compression-decompression, Admis hospital admission, CPR cardiopulmonary resuscitation, Disch hospital discharge, ITD impedance threshold device, ROSC return of spontaneous circulation
One-hour and twenty-four-hour survival rates in patients with a witnessed arrest were dramatically higher after ACD = CPR + ITD—68 and 55 %, respectively, versus 27 and 23 % with standard CPR (p = 0.002 and 0.009) (shown in Fig. 38.14).
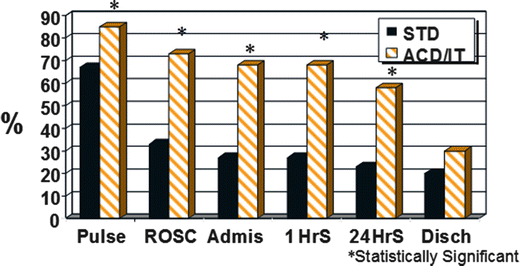
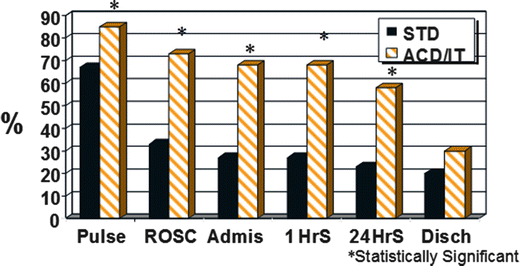
Fig. 38.14
Outcomes in patients randomized with either standard CPR (STD) or ACD-CPR + ITD with witnessed ventricular fibrillation in Mainz, Germany (n = 70). 1HrS 1-hour survival, 24HrS 24-hour survival, ACD active compression-decompression, Admis hospital admission, CPR cardiopulmonary resuscitation, Disch hospital discharge, ITD impedance threshold device, ROSC return of spontaneous circulation
Hospital discharge rates were 18 % after ACD-CPR + ITD versus 13 % in control subjects (P = 0.41). Overall neurological function trended higher with ACD-CPR + ITD versus control subjects (P = 0.07).
Importantly, patients randomized >10 min after the call for help to the ACD + ITD CPR group had a greater than three times higher 1-h survival rate (44 %) than control subjects (14 %) (P = 0.002). These time-related benefits were observed regardless of presenting rhythm. It should be noted that neurological outcomes in the survivors with delays to treatment with ACD-CPR + ITD were similar to those who were treated with ACD-CPR + ITD more rapidly.
Another prospective blinded study performed in France also demonstrated significantly increased 24-h survival rates with use of ACD-CPR + ITD [31]. In one arm of this study, 200 patients were treated by advanced life support personnel with ACD-CPR and an active ITD, and another 200 patients were randomized to the control group and received treatment with ACD-CPR and a sham ITD. As in other studies from France, most of the patients had an initial rhythm of asystole [28, 29]. The group treated with ACD-CPR and an active ITD had 24-h survival rates of 32 % compared with 24-h survival rates of 22 % in the control population (P < 0.05). Because of long EMS response times, survival rates in both groups were very low, but differences in neurological function in the survivors trended in favor of the ACD-CPR + ITD group. Only 1/8 (12 %) of survivors treated with the sham device had normal cerebral function at the time of hospital discharge, versus 6/10 (60 %) in the functional ITD group (p < 0.07).
< div class='tao-gold-member'>
Only gold members can continue reading. Log In or Register a > to continue
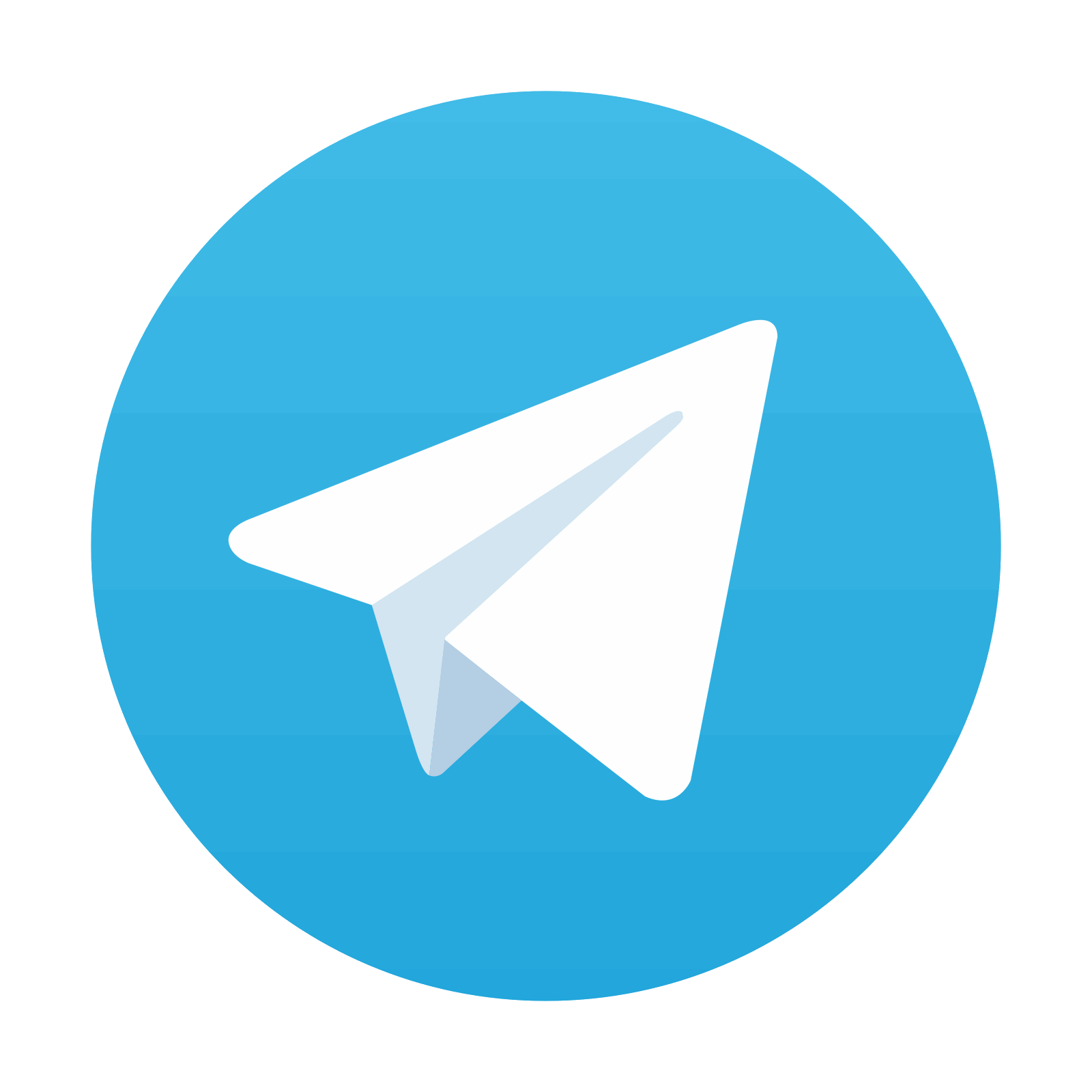
Stay updated, free articles. Join our Telegram channel

Full access? Get Clinical Tree
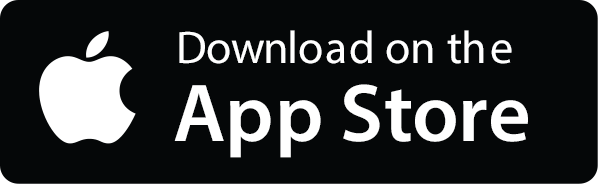
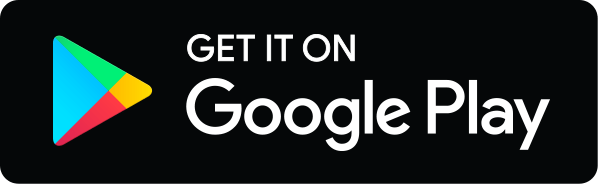