Fig. 1.1
The major components of the cardiovascular system: circulating blood, the blood vessels, the heart, and the lymphatic system. (Left) Major vessels that return deoxygenated blood to the heart (blue) and major arteries carrying oxygenated blood that leave the heart (red). (Right) Shown is the relative extent of the lymphatic system within the human body
1.2.1 Blood
Blood is composed of formed elements (cells and cell fragments) which are suspended in the liquid fraction known as plasma. Blood, often considered as the only liquid connective tissue in the body, has three general functions: (1) transportation (e.g., O2, CO2, nutrients, waste, hormones), (2) regulation (e.g., pH, temperature, osmotic pressures), and (3) protection (e.g., against foreign molecules and diseases, as well as for clotting to prevent excessive loss of blood). Dissolved within the plasma are many proteins, nutrients, metabolic waste products, and various other molecules being transported between multiple organ systems.
The formed elements in blood include red blood cells (erythrocytes), white blood cells (leukocytes), and the cell fragments known as platelets. These are all formed in bone marrow from a common stem cell. In a healthy individual, the majority of bloods cells are red blood cells (~99 %) which have a primary role in O2 exchange. Hemoglobin, the iron-containing heme protein which binds oxygen, is concentrated within the red cells; hemoglobin allows blood to transport 40–50 times the amount of oxygen that plasma alone could carry. The white cells are required for the immune process, e.g., to protect against infections and also cancers. Platelets play a primary role in blood clotting. In a healthy cardiovascular system, the constant movement of blood helps keep these various cells and plasma constituents well dispersed throughout the larger-diameter vessels.
The hematocrit is defined as the percentage of blood volume that is occupied by the red cells (erythrocytes). It can be easily measured by centrifuging (spinning at high speed) a sample of blood, which forces these cells to the bottom of the centrifuge tube. The leukocytes remain on the top and the platelets form a very thin layer between the cell fractions (other more sophisticated methods are also available for such analyses). Normal hematocrit is approximately 45 % in men and 42 % in women. The total volume of blood in an average-sized individual (70 kg) is approximately 5.5 L; hence, the average red cell volume would be roughly 2.5 L. Since the fraction containing both leukocytes and platelets is normally relatively small or negligible, in such an individual, the plasma volume can be estimated to be 3.0 L. Approximately 90 % of plasma is water which acts: (1) as a solvent, (2) to suspend the components of blood, (3) in the absorption of molecules and their transport, and (4) in the transport of thermal energy. Proteins make up 7 % of the plasma (by weight) and exert a colloidc osmotic pressure. Protein types include albumins, globulins (antibodies and immunoglobulins), and fibrinogen. To date, more than 100 distinct plasma proteins have been identified, and each presumably serves a specific physiologic function. The other main solutes in plasma include: electrolytes, nutrients, gases (some O2, large amounts of CO2 and N2), regulatory substances (enzymes and hormones), and waste products (urea, uric acid, creatine, creatinine, bilirubin, and ammonia).
1.2.2 Blood Vessels
Blood flows throughout the body’s tissues within blood vessels via bulk flow (i.e., all constituents together and in one direction). An extraordinary degree of vascular branching exists within the human body, which ensures that nearly every cell in the body lies within a short distance from at least one of the smallest branches of this system—a capillary. Nutrients and metabolic end products move between the capillary vessels and the surroundings of the cell through the interstitial fluid by diffusion. Subsequent movement of these molecules into a cell is accomplished by both diffusion and mediated transport. Nevertheless, blood flow through all organs can be considered as somewhat passive and occurs only because arterial pressure is kept higher than venous pressure via the pumping action of the heart.
In an individual at rest at any given moment, approximately 5 % of the total circulating blood is actually within the capillaries. Yet, this volume of blood can be considered to perform the primary functions of the entire cardiovascular system, specifically the supply of nutrients and removal of metabolic end products. The cardiovascular system, as reported by the British physiologist William Harvey in 1628, is a closed-loop system, such that blood is pumped out of the heart through one set of vessels (arteries) and then returns to the heart in another (veins).
More specifically, one can consider that there are two closed-loop systems which both originate and return blood to the heart—the pulmonary and systemic circulations (Fig. 1.2). The pulmonary circulation is composed of the right heart pump and the lungs, whereas the systemic circulation includes the left heart pump which supplies blood to the systemic organs (i.e., all tissues and organs except the gas exchange portion of the lungs). Because the right and left heart pumps function in a series arrangement, both will circulate an identical volume of blood in a given minute (one’s cardiac output, normally expressed in liters per minute).
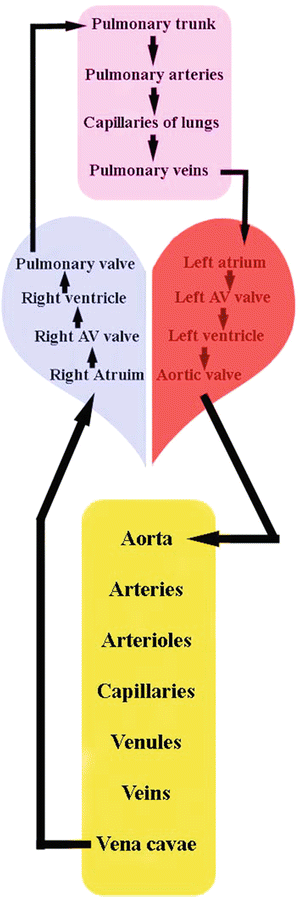
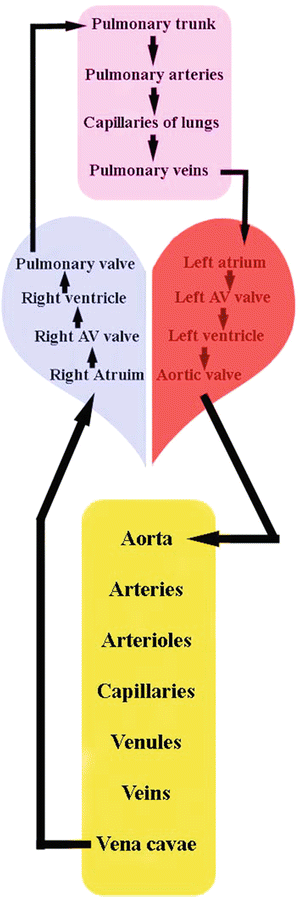
Fig. 1.2
The major paths of blood flow through pulmonary and systemic circulatory systems. AV atrioventricular
In the systemic circuit, blood is ejected out of the left ventricle via a single large artery—the aorta. All arteries of the systemic circulation branch from the aorta (this is the largest artery of the body, with a diameter ranging from 2 to 4 cm) and divide into progressively smaller vessels. The aorta’s four principle divisions are the ascending aorta (begins at the aortic valve where, close by, the two coronary artery branches have their origin), the arch of the aorta, the thoracic aorta, and the abdominal aorta.
The smallest of the arteries eventually branch into arterioles. They, in turn, branch into an extremely large number of the smallest diameter vessels—the capillaries (with an estimated ten billion in the average human body). Next, blood exits the capillaries and begins its return to the heart via the venules. Microcirculation is a term coined to collectively describe the flow of blood through arterioles, capillaries, and the venules (Fig. 1.3).
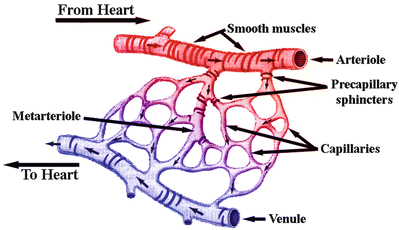
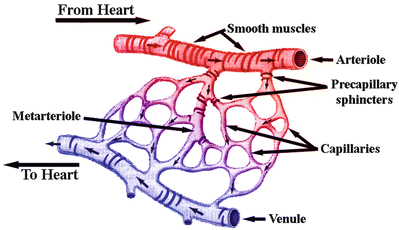
Fig. 1.3
The microcirculation including arterioles, capillaries, and venules. The capillaries lie between, or connect, the arterioles to the venules. They are found in almost every tissue layer of the body, but their distribution varies. Capillaries form extensive branching networks that dramatically increase the surface areas available for the rapid exchange of molecules. A metarteriole is a vessel that emerges from an arteriole and supplies a group of 10–100 capillaries. Both the arteriole and the proximal portion of the metarterioles are surrounded by smooth muscle fibers whose contractions and relaxations regulate blood flow through the capillary bed. Typically, blood flows intermittently through a capillary bed due to the periodic contractions of the smooth muscles (5–10 times per minute, vasomotion), which is regulated both locally (metabolically) and by autonomic control (Figure modified from [5])
Importantly, blood flow through an individual vascular bed is profoundly regulated by changes in activity of the sympathetic nerves innervating the arterioles. In addition, arteriolar smooth muscle is very responsive to changes in local chemical conditions within an organ (i.e., those changes associated with increases or decreases in the metabolic rate of that given organ).
Capillaries, which are the smallest and most numerous blood vessels in the human body (ranging from 5 to 10 μm in diameter and again numbering around ten billion), are also the thinnest walled vessels; an inner diameter of 5 μm is just wide enough for an erythrocyte to squeeze through. Further, it is estimated that there are 25,000 miles of capillaries in an adult, each with an individual length of about 1 mm.
Most capillaries are little more than a single cell layer thick, consisting of a layer of endothelial cells and a basement membrane. This minimal wall thickness facilitates the capillary’s primary function, which is to permit the exchange of materials between cells in tissues and the blood within. As mentioned above, small molecules (e.g., O2, CO2, sugars, amino acids, and water) are relatively free to enter and leave capillaries readily, promoting efficient material exchange. Nevertheless, the relative permeability of capillaries varies from body region to body region, with regard to the physical properties of their formed walls.
Based on such differences, capillaries are commonly grouped into two major classes: continuous and fenestrated capillaries. In the continuous capillaries, which are more common, the endothelial cells are joined together such that the spaces between them are relatively narrow (i.e., narrow intercellular gaps). These capillaries are permeable to substances having small molecular sizes and/or high lipid solubilities (e.g., O2, CO2, and steroid hormones) and are somewhat less permeable to small water-soluble substances (e.g., Na+, K+, glucose, and amino acids). In fenestrated capillaries, the endothelial cells possess relatively large pores that are wide enough to allow proteins and other large molecules to pass through. In some such capillaries, the gaps between the endothelial cells are even wider than usual, enabling quite large proteins (or even small cells) to pass through. Fenestrated capillaries are primarily located in organs whose functions depend on the rapid movement of materials across capillary walls, e.g., kidneys, liver, intestines, and bone marrow.
If a molecule cannot pass between capillary endothelial cells, then it must be transported across the cell membrane. The mechanisms available for transport across a capillary wall differ for various substances depending on their molecular size and degree of lipid solubility. For example, certain proteins are selectively transported across endothelial cells by a slow, energy-requiring process known as transcytosis. In this process, the endothelial cells initially engulf the proteins in the plasma within capillaries by endocytosis. The molecules are then ferried across the cells by vesicular transport and released by exocytosis into the interstitial fluid on the other side. Endothelial cells generally contain large numbers of endocytotic and exocytotic vesicles, and sometimes these fuse to form continuous vesicular channels across the cell.
The capillaries within the heart normally prevent excessive movement of fluids and molecules across their walls, but clinical situations have been noted where they may become “leaky.” For example, capillary leak syndrome may be induced following cardiopulmonary bypass and might last from hours up to days. More specifically, in such cases, the inflammatory response in the vascular endothelium can disrupt the “gatekeeper” function of capillaries; their increased permeability will result in myocardial edema.
From capillaries, blood throughout the body then flows into the venous system. It first enters the venules which then coalesce to form larger vessels—the veins (Fig. 1.3). Then veins from the various systemic tissues and organs (minus the gas exchange portion of the lungs) unite to form two major veins—the inferior vena cava (lower body) and superior vena cava (above the heart). By way of these two great vessels, blood is returned to the right heart pump, specifically into the right atrium.
Like capillaries, the walls of the smallest venules are very porous and represent the sites where many phagocytic white blood cells emigrate from the blood into inflamed or infected tissues. Venules and veins are also richly innervated by sympathetic nerves and thus the smooth muscles within constrict when these nerves are activated. Therefore, increased sympathetic nerve activity is associated with a decreased venous volume, which results in increased venous return and hence cardiac filling and ultimately an increased cardiac output (via Starling’s law of the heart).
Many veins, especially those in the limbs, also feature abundant valves (which are notably also found in the cardiac venous system) which are thin folds of the intervessel lining that form flap-like cusps. The valves project into the vessel lumens and are directed toward the heart, thus promoting unidirectional flow of blood. Because blood pressure is normally low in veins, these valves are important for aiding venous return, by preventing the backflow of blood (which is especially true in the upright individual). In addition, contractions of skeletal muscles (e.g., in the legs) also play a role in decreasing the size of the venous reservoir and thus the return of blood volume to the heart (Fig. 1.4).
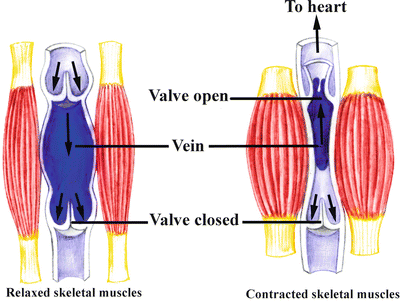
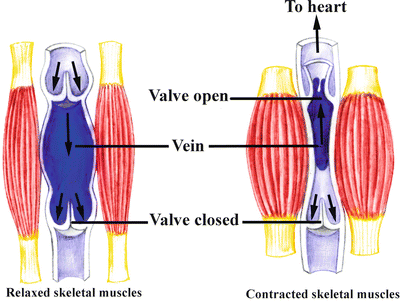
Fig. 1.4
Contractions of the skeletal muscles aid in returning blood to the heart—skeletal muscle pump. While standing at rest, the relaxed vein acts as a reservoir for blood; contractions of limb muscles not only decrease this reservoir size (venous diameter) but also actively force the return of more blood to the heart. Note that the resulting increase in blood flow due to the contractions is only toward the heart due to the valves that are present within the veins
The pulmonary circulation is comprised of a similar circuit. Blood leaves the right ventricle in a single great vessel, the pulmonary artery (trunk), which, within a short distance (centimeters), divides into the two main pulmonary arteries, one supplying the right lung and another the left. Once within the lung proper, the arteries continue to branch down to arterioles and then ultimately form capillaries. From there, the blood flows into venules, eventually forming four main pulmonary veins which empty into the left atrium. As blood flows through the lung capillaries, it picks up oxygen supplied to the lungs by breathing air; hemoglobin within the red blood cells is loaded up with oxygen (oxygenated blood).
< div class='tao-gold-member'>
Only gold members can continue reading. Log In or Register a > to continue
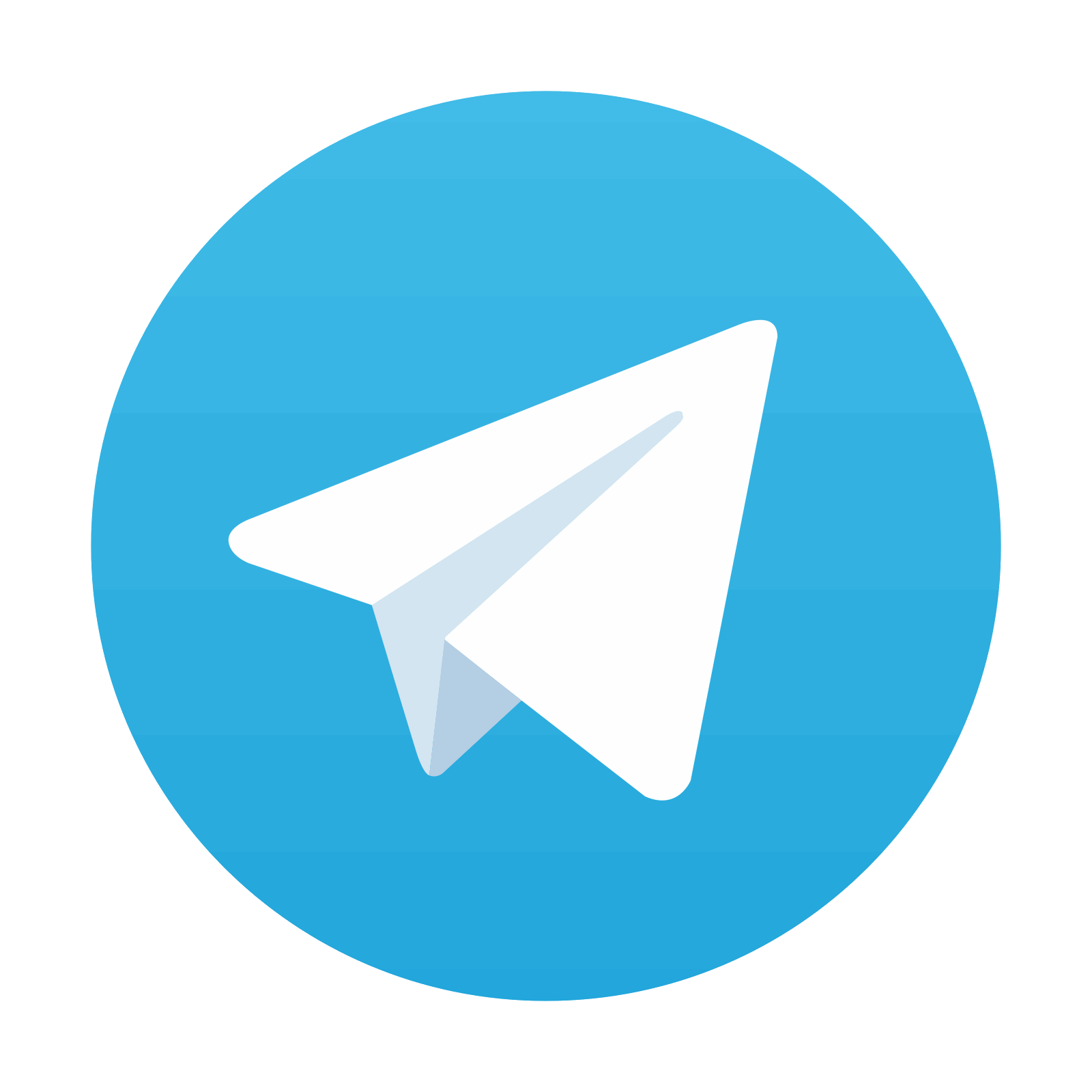
Stay updated, free articles. Join our Telegram channel

Full access? Get Clinical Tree
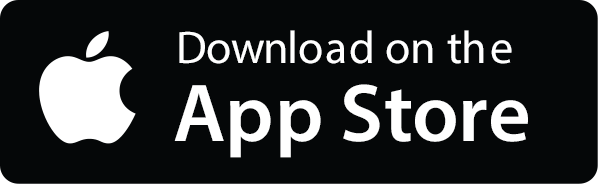
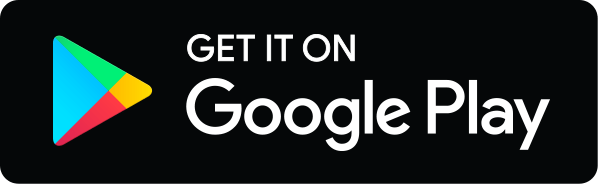