CHAPTER
1
Fundamentals
THE CARDIAC ACTION POTENTIAL
Myocardial tissue contains 5 main properties that integrate the electrical and mechanical activity.
○ Automaticity: The ability of a cell/tissue to initiate an impulse or stimulus. In the absence of external stimulation, the pacemaker cells spontaneously depolarize. This property generates sinus rhythm at a rate appropriate to the body’s needs.
○ Excitability: The ability of a cell/tissue to respond to an impulse or stimulus. Myocardial cells respond to the impulse generated by the pacemaker cells of the cardiac conduction system through depolarization and repolarization.
○ Conductivity: The ability of a cell or tissue to transmit impulses to other areas. While conductivity is much more efficient in the conduction system, the cells in the conduction system and the myocardium also have this property.
○ Refractoriness: The property that governs the time following excitation until the tissue can be re-excited. This property prevents tissue from being re-excited too soon after the previous excitation, thereby protecting against dangerously rapid rates and reentrant arrhythmias.
○ Contractility: The ability of a cell or tissue to respond to electrical stimulation with mechanical action.
The “Fast-Channel” Cardiac Action Potential
Characteristic of action potentials found in the atrial and ventricular myocardium, as well as the rapidly conducting His-Purkinje system—consisting of the bundle of His, right and left bundle branches, fascicular Purkinje cells, and endocardial Purkinje-cell conduction system.
These action potentials have a true resting potential, a rapid depolarization phase, and a prolonged plateau phase.
Depolarization
When the cardiac muscle cell is stimulated, the cell undergoes an electrical event called depolarization.
○ Phase 0: Rapid depolarization phase (“upstroke”)
▪ With stimulation, the fast sodium (Na) channels are activated, resulting in a rapid increase in sodium membrane conductance (GNa) and/or rapid influx of sodium ions (INa) into the cell.
▪ The entry of positively charged sodium ions produces a rapid change in the electrical charge of the interior of the cell, providing the energy for rapid impulse propagation (conduction velocity of ~1 m/s).
Repolarization
Almost immediately after depolarization, the inactivation of the fast sodium channels arrests Na+ movement into the cell, allowing the cell to initiate the restoration of its (inactive) resting state.
○ Phase 1: Early repolarization
The early repolarization phase starts with the opening of rapid, outward potassium current (Ito). This currents result in rapid repolarization to about 0 mV (with time-dependent inactivation).
○ Phase 2: “Plateau” phase
A “stable” membrane potential is observed resulting from a balance of the inward movement of calcium through L-type calcium channels (ICa,L) and the outward movement of K+ through the delayed rectifier (rapid and slow components: IKr and IKs) and the inward rectifier (IK1) potassium channels. The sodium–calcium exchange current (INa/Ca) and the sodium–potassium pump current (INa/K) also play minor roles in the maintenance of the current, and major roles in the maintenance of physiological intracellular sodium, potassium, and calcium concentrations.
○ Phase 3: Rapid repolarization at the conclusion of the plateau phase
Initially, the net negative change in membrane potential is driven by the inactivation of L-type Ca channels. The rapid delayed rectifier K+ channel (IKr) and inwardly rectifying K+ current (IK1) activate, causing a more rapid net outward current, causing the cell to repolarize to baseline. This phase governs refractoriness by controlling action potential duration (APD). INa is inactivated at voltages positive to –60 mV, so following the phase 0 upstroke, the cell cannot be reactivated until it returns to –60 mV during phase 3. The APD to –60 mV determines the “effective refractory period” (ERP) of fast-channel tissue.
○ Phase 4: Resting phase
The resting phase constitutes a steady, stable, polarized membrane (~–90 mV in working myocardial cells). When membrane potential is restored to baseline, the delayed rectifier K+ channels close. Voltage-regulated inward rectifiers (IK1) remain open, regulating resting membrane potential.
Ventricular Action Potential
Compared to the atrium, the ventricular action potential has a:
○Longer duration
○Higher phase 2 (absent IKur)
○Shorter phase 3, with faster repolarization
Action Potential of the His-Purkinje System
Compared to the myocardium, the action potential of the His-Purkinje system displays the following differences:
○More prominent early (phase 1) repolarization
○Longer plateau phase (phase 2)
○Automaticity: Spontaneous, phase 4 depolarization
The “Slow-Channel” Cardiac Action Potential
Characteristic of action potentials found in the sinoatrial (SA) and atrioventricular (AV) nodes. The key features of these action potentials are the property of automaticity, as well as the fact that the depolarization phase is slower, and with a shorter APD than “fast-channel” action potentials.
○ Phase 0: Rapid depolarization phase (“upstroke”)
This phase is slower (conduction velocity of ~0.02 to 0.05 m/s) due to a smaller inward current that governs activation (generated by ICa,L, rather than INa).
○ Phase 1: Early repolarization phase
Early repolarization is not perceptible in slow-channel tissue, because Ito is small and partially inactivated by the relatively positive resting potential.
○ Phase 2: “Plateau” phase
In principle, this phase is similar to that of fast-channel tissue, except that because of the small phase 0 current, the transitions from phase 0 to phase 1 and subsequently to phase 2 are much less defined.
○ Phase 3: Rapid repolarization at the conclusion of the plateau phase
This phase has similar ionic mechanisms to fast-channel phase 3. Because ICa,L is smaller and recovers much more slowly than INa, the main factor determining ERP in slow-channel tissue is slow, time-dependent recovery of ICa,L. Therefore, unlike fast-channel tissue, APD is not the main determinant of ERP and changes in APD have relatively little effect on ERP. The time-dependent recovery of ICa,L results in reduced current at fast rates, greatly limiting the maximum follow frequency of the AVN. This is an important protective property that prevents excessively rapid ventricular rates during very rapid supraventricular tachyarrhythmias like atrial flutter (AFL) and atrial fibrillation (AF).
○ Phase 4: “Resting” phase
Automaticity results from the combination of: (1) spontaneous diastolic depolarization due to phase 4 If (a poorly selective, inward current carried mainly by Na and activating upon repolarization), activation of T-type Ca2+ current (ICa,T), and inactivation of delayed rectifier K+ currents; and (2) the maximum negative “resting” potential (“maximum diastolic potential” or MDP) being closer to the depolarization threshold (–50 to –60 mV vs. –80 mV for fast-channel potentials). As a result, the SA node acts as the predominent cardiac pacemaker, with the AVN acting as the primary backup “escape” if the SA node fails.
ARRHYTHMIA MECHANISMS
General Classification of Arrhythmia Mechanisms
Table 1.1 Characteristics of Arrhythmia Mechanisms
Focal | ||||
Macroreentry | Microreentry | Triggered Activity | Automaticity | |
Initiation by programmed stimulation | Yes | Yes | Yes | No |
Termination by programmed stimulation | Yes (Abrupt) | Yes | Yes (Gradual) | No (Transient suppression) |
Resetting | Yes | Yes | Yes | Yes |
Entrainment | Yes | Yes | No | No |
Site specificity of entrainment | Yes | Yes | No | No |
Overdrive suppression | No | No | Yes | Yes |
Overdrive acceleration | Yes | Yes | Yes | No |
Adenosine sensitivity | No | No | Yes (Terminates) | No (Transient suppression) |
Catecholamine sensitivity | Maybe | Maybe | Yes | Yes |
Activation recorded throughout the cycle | 100% | 100% (50% local EGM) | <50% | <50% |
Size | >2 atrial segments | ≤2 atrial segments | 1 atrial segment | 1 atrial segment |
Ablation target | Bidirectional block across critical isthmus | Origin |
Altered Impulse Formation
Automaticity
The ability of a cell to depolarize itself to threshold and spontaneously generate an action potential.
○Driven by spontaneous, phase 4 depolarization in the absence of external stimulation.
○May be influenced by neurohormonal (sympathetic and parasympathetic) input.
○While all cardiac tissues can initiate depolarization, only certain cell or tissue types routinely possess this pacemaker function (in ascending order of importance):
▪ Sinus node
▪ Specialized atrial cells, such as the AVN
▪ Bundle of His
▪ Right and left bundle branches
▪ Fascicular Purkinje cells
▪ Ventricular endocardial Purkinje conduction system
○ Abnormal automaticity (accelerated phase 4 upslope) can lead to extrasystoles or sustained tachyarrhythmias.
▪ Most often these arrhythmias are due to metabolic causes:
• Acute ischemia or hypoxia
• Myocardial stretch
• Electrolyte abnormalities (hypoxemia, hypokalemia, hypomagnesemia, acid-base disorders)
• High sympathetic tone
▪ Arrhythmias arising from abnormal automaticity
• May exhibit a rate increase at onset (“warm-up”) or a rate decrease at termination (“cool-down”).
• They generally cannot be induced by pacing, and therefore are not amenable to provocative electrophysiology (EP) testing.
Triggered Activity
Refers to a situation where heart tissue is stimulated once, but the stimulus results in the production of more than one conducted beat.
○Arrhythmias can have features characteristic for automaticity and reentry.
▪ Automaticity: A new action potential can be generated by the leakage of positive ions into the cell.
• The arrhythmia displays typical warm-up and cool-down and may respond to calcium- or sodium-channel blockers.
▪ Reentry can be induced by premature beats and programmed pacing.
• In contrast to typical reentry, the induction of triggered activity may require the introduction of a pause into the pacing sequence (“pause-dependent” arrhythmias).
○The two most common forms of triggered activity are:
▪ Early afterdepolarization (EAD), which occurs before complete cellular repolarization.
• EADs result from the reactivation of ICa,L and/or spontaneous Ca2+ release from the sarcoplasmic reticulum (SR).
• Plateau EAD occurs during phase 2.
• Late EADs occur during phase 3 and are caused by reactivation of INa or spontaneous Ca2+ release from the SR.
▪ Delayed afterdepolarization (DAD) occurs after complete repolarization of the cell during phase 4 of the action potential.
• DAD is caused by intracellular Ca2+ overload or RyR2 dysfunction, with spontaneous release of Ca2+ from the SR resulting in a depolarizing current via Na+/Ca2+ exchange (NCX) current. When extruding excess Ca2+ from inside the cell, NCX-mediated efflux of 1 calcium ion in exchange for 3 sodium ions results in membrane depolarization during the diastolic interval.
Altered Impulse Conduction
Reentry
Inhomogeneous conduction results in reentry of an impulse into an area that was just depolarized and has since repolarized.
○Requires 2 discrete parallel conducting “pathways” that:
▪ Connect proximally and distally
▪ Are separated by a barrier
• Anatomic: Valve, vessel, scar
• Functional: A central core that is either continuously depolarized by centripetal waves (leading circle) or excitable, but not excited (spiral wave)
▪ Contain different electrophysiologic properties
• One of the pathways must have a refractory period substantially longer than the refractory period of the other (“fast” β pathway), and the pathway with the shorter refractory period must conduct electrical impulses more slowly than the other pathway (“slow” α pathway).
○ Mechanism of reentry (anatomical)
▪ In sinus rhythm, the wavefront depolarizes both pathways simultaneously (Panel 1).
▪ An event (i.e., atrial or ventricular extrasystole) exposes the presence of the pathways due to their differing conduction velocity and recovery.
• Premature impulse enters the circuit when the β (fast) pathway is refractory and at a time when the α (slow) pathway has recovered and is able to accept the impulse (Panel 2).
• While the α pathway slowly conducts the impulse, the β pathway has the chance to recover.
• By the time the impulse reaches the distal end of the α pathway, the β pathway has recovered and is able to conduct retrograde back up to the atria (echo beat: Panel 3).
• If the α (slow) pathway has recovered, then the impulse can reenter the α (slow) pathway and initiate a circular impulse movement arrhythmia.
▪ Note: There is no single point of origin of activation.
• Reentrant activation can be recorded throughout the entire cycle length (CL); a point of earliest activity does not exist.
• Atrial myocardium surrounding the circuit is activated from various parts of the circuit.
Terminology of Reentry
○ Cycle length: Time required for depolarization to complete the circuit and return to its spatial origin
○ Wavelength: The minimum path length that can support reentry
▪ Wavelength is the product of the conduction velocity and the refractory period (the shortest coupling interval that captures the reentrant circuit).
○ Temporal excitable gap: The time interval during the CL when the circuit may be “excited”
▪ The temporal excitable gap is the difference between the CL and the refractory period.
○ Spatial excitable gap: The spatial distance within the circuit that is excitable
▪ The spatial excitable gap is the difference between the total circuit distance and the wavelength.
Classification of Reentry
○ Microreentry: A reentry circuit too small to be seen on the surface electrocardiogram (ECG) (e.g., atrioventricular nodal reentry tachycardia [AVNRT])
○ Macroreentry: A reentry circuit large enough to be seen on the surface ECG (e.g., AFL)
ENTRAINMENT
Demonstration of entrainment is proof that an arrhythmia is due to a reentrant circuit with an excitable gap between the tail of one wavefront and the head of the next.
○A reentrant tachycardia may be reset by premature extrastimuli.
○If a train of pacing stimuli is delivered with a pacing CL shorter than the tachycardia cycle length (TCL), the tachycardia may be continuously reset with each successive pacing stimulus.
▪ Continuous resetting is called entrainment.
▪ Entrainment is present when at least two consecutive stimuli are conducted orthodromically through the circuit with the same conduction time.
Criteria of Entrainment
○There is constant fusion at a pacing rate and the last beat is entrained but not fused.
▪ Pattern of activation represents a combination of pacing and tachycardia.
▪ When pacing is terminated, the last paced beat is early (entrained) but propagates around the circuit (activation pattern similar to spontaneous arrhythmia).
○There is progressive fusion as the pacing rate increases:
▪ At a pacing CL just short of the tachycardia, there is minimal invasion of the circuit and activation mainly resembles the spontaneous tachycardia (fusion).
▪ With progressive shortening of the pacing CL, the circuit is invaded to a greater extent, leading to an alteration in activation closer to that of pacing.
○Tachycardia interruption is accompanied by a block to a recording site, followed by activation at the same site from a different direction.
○ There is demonstration of a change in conduction time and electrogram (EGM) morphology at one recording site when pacing from another site at 2 different constant pacing rates, each of which is faster than the spontaneous rate of tachycardia but fails to interrupt it.
Uses of Entrainment
○ Proves the arrhythmia mechanism is reentry
▪ Note: Entrainment does not prove that the pacing site is within the circuit, because if the excitable gap is large, then entrainment can be achieved by pacing at a large distance from the circuit.
○ Indicates the location is part of the reentrant circuit
○ May also indicate a good site for ablation (i.e., concealed fusion with latency and good post-pacing interval [PPI])
Entrainment and Specific Arrhythmias
○ AFL
▪ Entrainment of the cavotricuspid isthmus (CTI) is important to show it is part of the circuit.
○ Supraventricular tachycardia (SVT)
▪ Ventricular entrainment is useful to distinguish atrial tachycardia (AT) (a VAAV response) from AVNRT/atrioventricular reciprocating tachycardia (AVRT) (a VAV response).
▪ His synchronous VPBs may reset AVRT but not AVNRT.
○ Ventricular tachycardia (VT)
▪ Entrainment mapping is used to complement activation mapping, substrate mapping, and pace mapping.
▪ The diastolic pathway is a target for ablation and may be identified by concealed entrainment with latency and a PPI-TCL of <30 ms.
RESETTING
The Physiologic Basis of Entrainment
○During sustained arrhythmia, a premature impulse may enter the excitable gap of the circuit before dividing into two wavefronts:
▪ Antidromic/retrograde wavefront (opposite in direction to the reentrant tachycardia), which collides with the preceding tachycardia wavefront
▪ Anterograde/orthodromic wavefront, which conducts through the reentrant circuit and exits at an earlier time (compared to the next expected beat of the tachycardia)
○Resetting is defined as:
▪ The advancement of the tachycardia by at least 20 ms
▪ The return tachycardia has the same activation/morphology and CL
▪ The premature impulse should find the excitable gap and invade the reentrant circuit
Post-pacing Interval (PPI)
○The return CL as measured from the last stimulus to the return EGM in the pacing electrode
○Represents the time taken for the impulse to travel from the pacing site to the tachycardia circuit, around the circuit, and back to the pacing site
▪ An in-circuit response is defined as a PPI of less than 30 ms.
○Note:
▪ The range of coupling intervals from onset of resetting to arrhythmia termination reflects the duration of the excitable gap.
▪ Resetting response may be characterized by plotting the return CL against the coupling intervals.
• Flat, increasing (increasing return cycles with decreasing coupling intervals), or mixed flat and increasing curves are typical for reentrant tachycardia.
• Flat or decreasing curves may be seen with triggered activity.
• Flat response may be seen with automatic tachycardia.
▪ Pacing from different sites may affect the resetting response in a reentrant tachycardia.
•
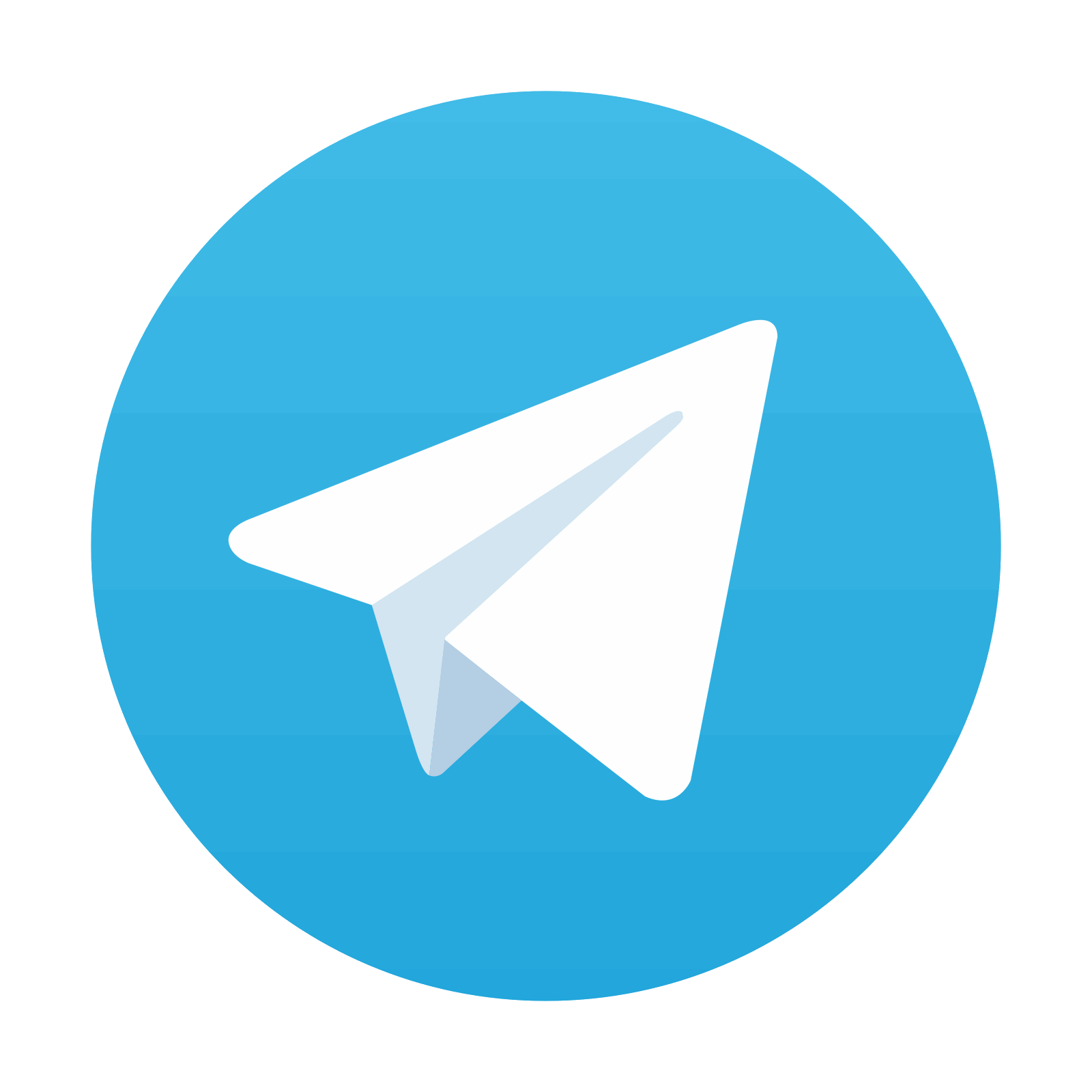
Stay updated, free articles. Join our Telegram channel

Full access? Get Clinical Tree
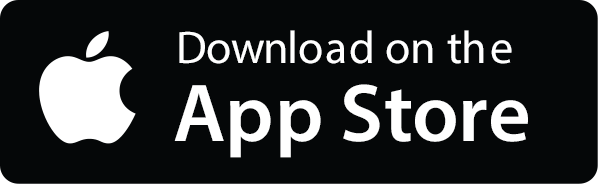
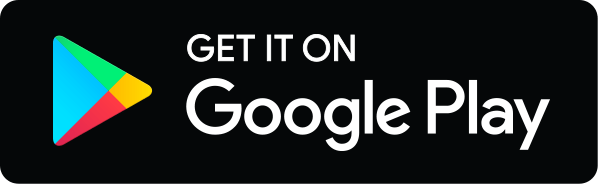