Exercise Electrocardiography
Peter M. Okin
Overview
Exercise electrocardiography (ECG) remains the most widely used method for assessment of the presence and severity of coronary artery disease. Clinical confidence in the exercise ECG has been eroded by the limited sensitivity and predictive value of standard ST-segment depression criteria and by the overapplication of bayesian principals to interpretation of the exercise ECG in comparison with other noninvasive modalities. However, the development of new approaches to analysis of the ST-segment response to exercise, including heart rate (HR) adjustment of ST-segment depression (the ST/HR slope and ST/HR index) and treadmill exercise scores, has produced a resurgence of clinical and research interest in the exercise ECG. These methods improve the accuracy of the exercise ECG for the identification and quantification of coronary disease and for risk stratification in both asymptomatic low-risk subjects and symptomatic patients with coronary disease. Application of HR-adjusted techniques has been supported by theoretic and experimental evidence relating the magnitude of ST-segment depression at peak exercise to both the area of ischemic territory and the degree of myocardial oxygen supply-demand mismatch as reflected by changing HR. Novel approaches to analysis of the ECG during exercise, including changes in QRS complex duration and high-frequency content, and assessment of HR responses during both exercise and recovery hold promise for future improvements in test performance.
Glossary
Bayesian analysis
Relation of test performance to population prevalence of disease.
Chronotropic response index
Fraction of heart rate reserve achieved with exercise adjusted by the fraction of metabolic reserve achieved.
ECG
Electrocardiogram or electrocardiography.
ETT
Exercise tolerance test (or exercise electrocardiogram).
J-Point
Junction of the end of the QRS complex and beginning of the ST segment.
Rate-recovery loop
Plot of ST-segment depression as a function of heart rate during both exercise and recovery.
ST/HR index
Maximal change in ST-segment depression with exercise divided by the total change in heart rate.
ST/HR slope
Linear regression-based calculation of maximal rate of change in ST-segment depression as a function of change in heart rate during exercise.
Introduction
Exercise ECG, or the exercise tolerance test (ETT), remains the most widely used and available technique for the investigation of known or suspected coronary artery disease. It has been estimated that 6 to 8 million treadmill tests are performed annually in the United States. Nonetheless, clinical confidence in the simple ETT has been eroded by the limited sensitivity and predictive value of standard ECG criteria. However, newer approaches to analysis of the ETT have been developed since the mid-1980s that improve accuracy of the exercise ECG to levels found for more expensive and less widely available imaging methods. This chapter reviews the development of exercise ECG, gives a brief review of the pathophysiologic basis for exercise-induced ST-segment depression, provides detailed information on the performance, interpretation, and
applications of the ETT, and addresses the controversies and future directions in exercise ECG.
applications of the ETT, and addresses the controversies and future directions in exercise ECG.
Performing an Exercise Electrocardiogram
Indications and Contraindications
The most widely accepted indications for performing an ETT are in patients with known or suspected coronary disease, to determine the likelihood of coronary disease, to assess the likelihood of anatomic or functionally severe disease that may be of prognostic importance, to determine functional capacity, and to assess the effects of therapy (1,2). Although indications for the exercise ECG continue to evolve, a joint committee of the American College of Cardiology and American Heart Association has published categories of indications for the ETT based on the literature (Table 48.1) (1). Clinical contraindications to performing diagnostic exercise ECG include acute myocardial infarction (MI), unstable angina before a period of stabilization, uncompensated severe congestive heart failure, advanced atrioventricular (AV) block or life-threatening arrhythmias, acute myocarditis or pericarditis, severe aortic stenosis, severe resting hypertension, and any medical condition that precludes the patient from being able to walk safely on the treadmill. The presence of left bundle branch block, left ventricular hypertrophy with strain, ventricular preexcitation (Wolf-Parkinson-White syndrome), or permanent ventricular pacing on the ECG are contraindications to use of the exercise ECG for diagnostic purposes, owing to the uncertain diagnostic value of additional ST-segment changes in these settings, but these conditions do not preclude use of the ETT to assess exercise performance or evaluate the risk of arrhythmia when indicated.
Patient Preparation and Technique
The American Heart Association has published guidelines for clinical ETT laboratories that provide recommendations regarding the performance of clinical ETTs (3). A directed physical examination should be performed to exclude the presence of significant valvular or myopathic disease that could preclude exercise evaluation. The risks and benefits of the procedure should be carefully explained and informed consent obtained before beginning the study.
Attention to adequate skin preparation before electrode placement is the most important technical aspect in obtaining high-quality ECG recordings. The areas where electrodes are to be placed should first be cleaned with alcohol to remove surface oils and then abraded with a scouring pad or other rough material to remove the most superficial layer of skin. Abrasion should be performed to reduce resistance at the skin-electrode interface to 5000 ohm or less. Silver-silver chloride electrodes should be used to produce high-quality tracings.
Before exercise, a supine 12-lead ECG should be obtained with the limb electrodes on the extremities in the normal positions; this tracing should be carefully examined for any signs of active ischemia, interval MI, or abnormalities that would prohibit performance of the ETT. Limb electrodes should then be shifted to the more proximal positions recommended by Mason and Likar (4), and additional ECG tracings should be obtained in the supine and standing positions.
A 12-lead ECG should be obtained each minute during exercise, at peak exercise, and every minute during the postexercise recovery period. Blood pressure should be measured immediately before exercise in the standing position, immediately before the end of each stage of exercise, and for each minute of recovery until the blood pressure has returned to baseline. Patient position in the postexercise recovery phase varies among laboratories. Many laboratories have the patient assume the supine position immediately after peak exercise; this will increase venous return and increase end-diastolic volume, and it may augment ST-segment changes that are present at the end of exercise. In our laboratory, we have the patient remain in the upright position, if possible, and have used a cool-down phase of 3 minutes immediately after peak exercise during which the patient continues to walk at 1.7 mph at a 0% grade. This allows patients a more gradual decrease in HR after exercise and appears to decrease the incidence of postexercise vagally mediated hypotension.
TABLE 48.1 Indications for exercise electrocardiography | ||
---|---|---|
|
TABLE 48.2 Comparison of standard Bruce protocol and the cornell protocol | ||||||||||||||||||||||||||||||||||||||||||||||||||||||||||||||||||||||||||||
---|---|---|---|---|---|---|---|---|---|---|---|---|---|---|---|---|---|---|---|---|---|---|---|---|---|---|---|---|---|---|---|---|---|---|---|---|---|---|---|---|---|---|---|---|---|---|---|---|---|---|---|---|---|---|---|---|---|---|---|---|---|---|---|---|---|---|---|---|---|---|---|---|---|---|---|---|
|
Equipment and Personnel
Although excellent-quality stress tests can be obtained using standard 12-lead ECGs that conform to the recommendations of the American Heart Association, most ETTs currently performed utilize equipment specifically designed for ETT. These computerized systems combine an ECG with a display monitor for continuous rhythm analysis and are capable of controlling treadmills and bicycle ergometers according to specific exercise protocols. The stress laboratory should be equipped with a defibrillator with the ability to perform synchronous cardioversion, an Ambu-bag and oxygen supply, and a cardiac arrest cart.
ETTs should be performed under the supervision of a physician who has been trained to conduct them. The degree of supervision required primarily depends on the type of patient tested, and it can range from direct performance of the test in patients who are at higher risk of complications to assigning the performance of the test to an appropriately trained exercise physiologist or specialist in patients at lower risk. In all cases, a physician should be immediately available during the ETT.
Treadmill Protocol
Although numerous excellent treadmill protocols are available for use, by far the most commonly used are the original protocol of Bruce (5) and modifications of this protocol. The Cornell protocol, originally developed in our laboratory to produce the smaller HR increments between stages necessary for calculation of the ST/HR slope (6), is a more graded modification of the Bruce protocol that divides each standard stage into 2-minute half-stages (Table 48.2).
Lead Systems
The number and type of leads used in exercise ECG vary widely, but with the advent of modern recording devices, most stress laboratories monitor and record at least three leads during the test. Although the use of all 12 conventional leads is becoming more common, almost all the useful diagnostic ST-segment information from these leads is present in limb leads II, III, aVF, and precordial leads V3 to V6. Bipolar lead systems that include one electrode in the V5 position provide additional diagnostic information; bipolar lead CM5 is the most sensitive for ST-segment changes (7) and is particularly important in optimal performance of the ST/HR slope (8). Preliminary results in 245 patients suggest that incorporation of ST-segment depression and elevation in right precordial leads may improve sensitivity of the ETT with no apparent decrease in specificity (9). Further validation of these findings will be necessary before routine use of right precordial leads can be recommended.
Indications for Test Termination
The general indications for terminating an ETT are outlined in Table 48.3. In the absence of symptoms or signs that warrant termination of the exercise ECG, patients should be exercised with 100% of age-predicted target HR as the goal. However, in general, achievement of greater than 85% of target HR provides an adequate level of stress for diagnostic exercise ECG (10). Various equations have been used to relate target HR to age, but the simplest and most commonly used are 220—age and 200—(age/2). Although angina alone is not an absolute indication for test termination, progressively worsening typical anginal symptoms should be considered a reason to stop the test, as are any other symptoms that limit further walking on the treadmill. ST-segment elevation in the absence of Q waves, which should be considered consistent with possible transmural injury, or marked ST-segment depression (>3 mm of additional ST-segment depression compared with baseline) warrants test termination in the absence of other findings.
Other Applications of Stress Testing
Performance of an ETT for indications other than routine assessment of the presence or severity of coronary disease or evaluation of functional capacity deserves comment. After uncomplicated MI, an ETT is frequently performed to assess functional capacity and to help stratify risk (11). When performed early after MI (i.e., before discharge or within 2 to 3 weeks of the infarction), submaximal symptom-limited testing is recommended (2) to a target HR of 140 beats per minute or a metabolic equivalent (MET) of 7 in patients less than 40 years old and to an upper rate of 130 beats per minute or a MET level of 5 in patients 40 years old and older. A full symptom-limited maximal test is appropriate more than 3 weeks after MI. Exercise ECGs are also commonly performed to evaluate the risk of exercise-induced tachyarrhythmias in patients with exercise-related presyncope or syncope.
Exercise ECG is also utilized after percutaneous coronary interventions to stratify risk and to aid in the assessment of
restenosis, and it appears to be well tolerated (12,13). However, reports of acute stent thrombosis associated with ETT soon after stent placement (14) raise the question whether ETT should be performed in the immediate poststent period. Until further data become available, caution should be employed in the utilization of ETT soon after the stent placement, with strong consideration given to the use of pharmacologic stress if early noninvasive assessment is indicated (14).
restenosis, and it appears to be well tolerated (12,13). However, reports of acute stent thrombosis associated with ETT soon after stent placement (14) raise the question whether ETT should be performed in the immediate poststent period. Until further data become available, caution should be employed in the utilization of ETT soon after the stent placement, with strong consideration given to the use of pharmacologic stress if early noninvasive assessment is indicated (14).
Interpretation of the Exercise Electrocardiogram
ST-Segment Depression Criteria
To appreciate fully the ST-segment excursions that occur during the transient ischemia associated with exercise in patients with coronary disease requires some understanding of the ST-segment changes that occur during exercise in physiologically normal subjects (Fig. 48.1). At baseline, most normal subjects have ST segments that are either at the isoelectric level or slightly elevated owing to physiologic early repolarization of the J-point. As HR increases, progressive J-point depression occurs, with resultant rapidly upsloping ST segments (15,16). After exercise is terminated, the J-point depression tends to resolve quickly (usually within the first 2 minutes), as does the rapidly upsloping ST-segment depression. In contrast, the ST-segment depression associated with subendocardial ischemia is more likely to be horizontal or downsloping, but it may also be slowly upsloping (Fig. 48.2) (10,16). With progressive exercise, increasing HR, and thus increasing myocardial oxygen demand, the magnitude of ischemic ST-segment depression will increase. Placing the patient in the supine position immediately after exercise may accentuate ischemic ST-segment depression; however, ischemic ST-segment depression may occur during exercise only; in a small percentage of cases, ischemic ST-segment depression will be seen during recovery only (17). In the rare instances of exercise-induced transmural ischemia or injury, ST-segment elevation similar to that seen during the acute injury of MI may also occur.
Differences in the patterns of ST-segment depression in normal subjects and patients with coronary disease highlight the importance of choosing the correct point on the ST segment to measure the magnitude of ST-segment depression (15,16,18). Recommendations for the measurement of ST-segment depression have varied from the J-point (19) to between 60 and 80 milliseconds after the J-point (1,10). Performance of standard ST-segment depression criteria vary with the timing of ST-segment measurement relative to the J-point, but measurements made at 60 milliseconds after the J-point have generally outperformed J-point measurements for the identification of coronary disease (15,16,18). We previously found that performance characteristics of HR-adjusted ST-segment depression criteria are optimized when ST-segment depression is measured at 60 milliseconds after the J-point, whereas use of J-point depression reduces performance of HR-adjusted criteria to the level of standard criteria (16). Thus, we currently recommend that ST-segment depression be measured at 60 milliseconds after the J-point (8).
![]() FIGURE 48.2. Comparison of the different patterns of ST depression. A: Slowly upsloping ST-segment depression. B: Horizontal ST-segment depression. C: Downsloping ST-segment depression. |
The actual measurement of ST-segment deviation on the exercise ECG is most accurately performed by the computerized systems that are widely in use today, but accurate ST-segment measurements can be performed by hand with careful attention to proper selection of the correct measurement points. When measuring ST-segment deviation, the PQ segment is usually chosen as the isoelectric point, and ST-segment depression should be measured at 60 milliseconds after the J-point. Computer systems are clearly capable of accurately measuring ST-segment deviation to the nearest 10 μV, and careful hand measurements using calipers or a magnifying graticle can readily achieve a precision of 25 μV for ST-segment measurements (20). However, when using computer measurements, it is important to recognize that motion artifact, electrical interference, and changes in QRS or AV conduction can interfere with PQ-segment and J-point determinations and can introduce error into the computerized ST-segment measurements. Therefore, physicians should routinely compare the raw ECG signal with the computer-averaged or median beats used to perform ST-segment measurements before accepting the computer measurements as accurate.
Standard Test Criteria
Assessment of the magnitude and configuration of ST-segment depression on the exercise ECG provides the basis for standard ST-segment depression criteria that are commonly in use. A positive test by standard ST-segment depression criteria has been routinely defined as the achievement of ≥0.1 mV (100 μV) of horizontal or downsloping ST-segment depression at peak exercise or in early recovery compared with the baseline before exercise ECG (1,2,10). However, as discussed later, these criteria have only limited test sensitivity for the presence of coronary disease and variable performance for identification of anatomically severe disease and for risk stratification (7,8,9,10, 18,21). Additional factors that can increase the likelihood of coronary disease in the presence of traditional criteria and also
make multivessel disease more likely include the magnitude, time of onset, duration, and number of leads with ST-segment depression. Although inclusion of upsloping ST-segment depression of ≥0.1 mV or 0.15 mV has been suggested as an additional component of standard criteria to increase sensitivity for the detection of coronary disease (22,23), the incorporation of upsloping ST-segment depression also lowers test specificity (10,21,22,24). As a consequence, we consider the presence of upsloping ST-segment depression of 1 mm (0.1 mV) or more to be an “equivocal” test response by standard criteria.
make multivessel disease more likely include the magnitude, time of onset, duration, and number of leads with ST-segment depression. Although inclusion of upsloping ST-segment depression of ≥0.1 mV or 0.15 mV has been suggested as an additional component of standard criteria to increase sensitivity for the detection of coronary disease (22,23), the incorporation of upsloping ST-segment depression also lowers test specificity (10,21,22,24). As a consequence, we consider the presence of upsloping ST-segment depression of 1 mm (0.1 mV) or more to be an “equivocal” test response by standard criteria.
Heart Rate–Adjusted Criteria
HR adjustment of the magnitude of ST-segment depression is a rational, physiologic approach to interpretation of the ETT. Two related but distinct methods of HR adjustment of ST-segment depression during exercise have evolved: the linear regression–based ST/HR slope and the simpler ST/HR index. The ST/HR slope is calculated from the maximal rate of change of ST-segment depression relative to HR during the period of active ischemia that accompanies the end of exercise (Fig. 48.3) (6,8,16,20,24,25,26,27,28,29,30). The ST/HR index represents the average change of ST-segment depression relative to HR change over the entire course of exercise, which therefore underestimates the maximal ST/HR slope because it includes a large change in HR before any ischemia occurs (see Fig. 48.3). Both these methods specifically do not consider any ST-segment depression that occurs during the postexercise recovery period.
Calculations of the ST/HR slope and ST/HR index are illustrated in Figure 48.3. Measurement of the ST/HR slope is determined by linear regression analysis to relate the magnitude of ST-segment depression in each lead to the HR at the end of each stage of exercise and at peak exercise. Because the maximal rather than the average rate of change is sought, linear regression analysis is performed from the end of exercise to progressively earlier intermediate stage data points using HR as the independent variable and ST-segment depression as the dependent variable. The highest ST/HR slope with a statistically significant correlation coefficient is taken as the test finding for that lead (in μV/beats per minute). After calculation of the maximal ST/HR slope in each lead, the highest ST/HR slope among all the leads (including bipolar lead CM5 but excluding aVR, aVL, and V1) is taken as the final test result (8,20). The ST/HR index is derived by dividing the maximal change in ST-segment depression during exercise by the total change in HR from rest to peak effort (8,31). Initial studies from our laboratory (24) established partition values with specificities of 95% in normal subjects of more than 2.40 μV/beat per minute for the ST/HR slope and more than 1.60 μV/beat per minute for the ST/HR index for the identification of coronary disease. Partition values of more than 6.0 μV/beat per minute and more than 3.3 μV/beat per minute, respectively, have been established for the identification of three-vessel, left main, or functionally severe coronary artery disease (8).
Analysis of the behavior of ST-segment depression as a function of HR during both exercise and recovery using the rate-recovery loop (8,25,26) can provide additional diagnostic and prognostic information from the ETT. Physiologic correlates of rate-recovery loop patterns can be found in the initial observations of Detry and Bruce (32,33), comparing myocardial ischemia during exercise and recovery. These investigators found a close linear relation of ST-segment depression during exercise to myocardial oxygen demand, as reflected by the tension-time index, in patients with coronary disease. During recovery, however, this relation was nonlinear, with similar ST-segment depressions observed at lower tension-time indexes than during exercise. Additional support for this approach is found in observations of subendocardial ischemia continuing into the recovery period with persistent ST-segment depression that remains greater relative to HR during early recovery than during the development of ischemia (34,35). Rate-recovery loops are constructed by plotting ST-segment deviation with reference to changing HR throughout treadmill exercise and recovery (Fig. 48.4). Normal subjects typically exhibit a clockwise loop of ST-segment depression as a function of HR during exercise and recovery, whereas patients with coronary disease commonly exhibit a counterclockwise loop. Quantification of the degree of abnormal rate-recovery loop behavior by integrating the difference in ST-segment depression between exercise and recovery phases from peak exercise to the end of 3 minutes of the recovery phase can further stratify the presence and severity of coronary disease (36).
Treadmill Exercise Scores
Numerous investigators have used either multivariate analyses or bayesian theory to produce clinical scores in an attempt to improve accuracy of the ETT
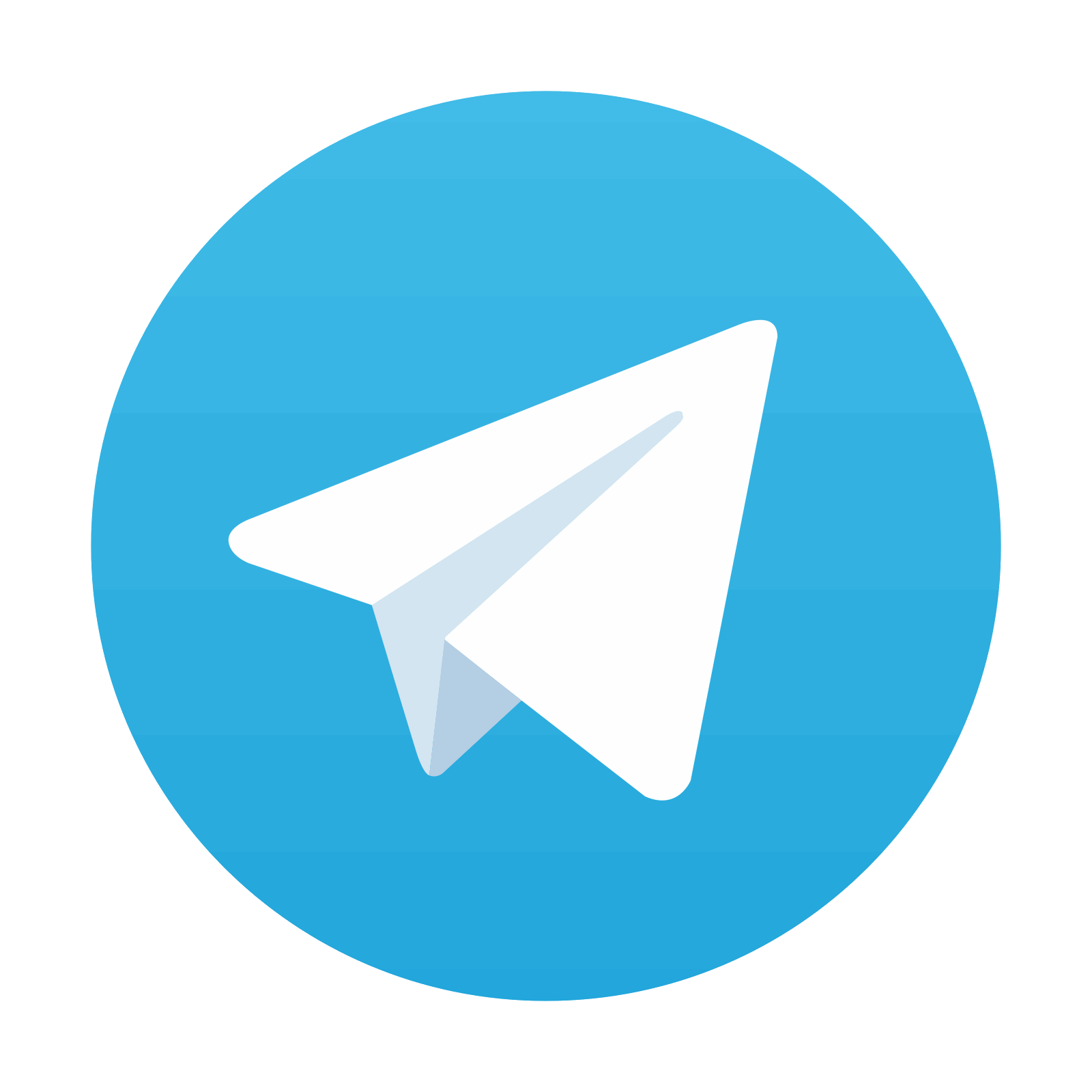
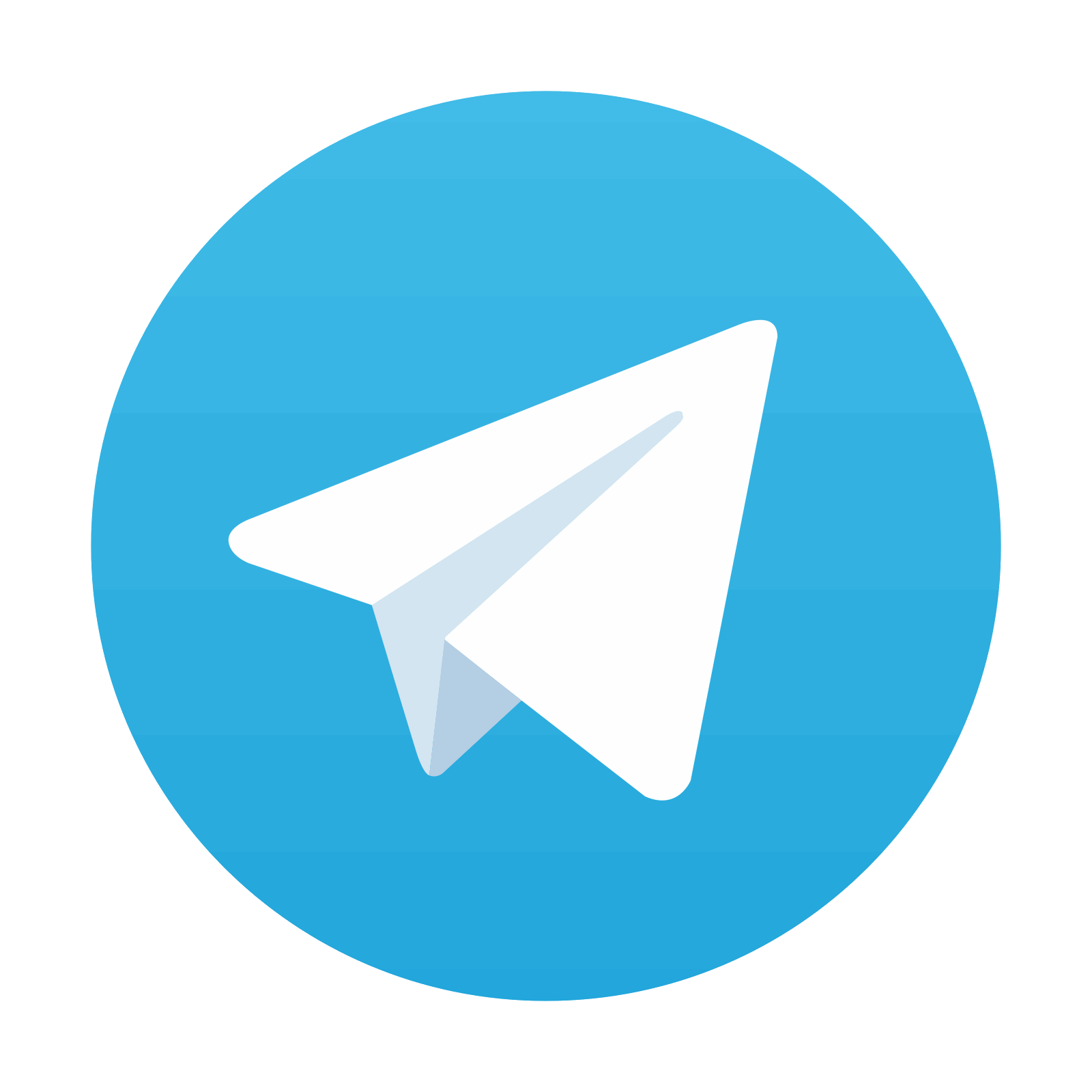
Stay updated, free articles. Join our Telegram channel

Full access? Get Clinical Tree
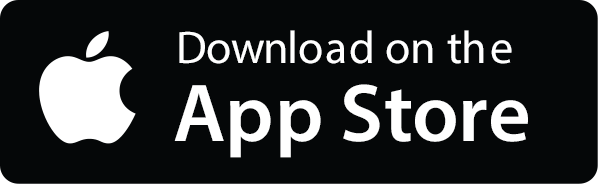
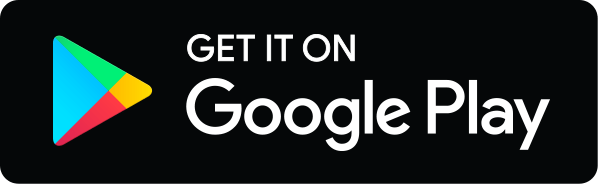
