Evaluation of Tamponade, Constrictive, and Restrictive Physiology
Mauro Moscucci
Barry A. Borlaug
The pericardium separates the heart from the surrounding structures, and it has important mechanical, membranous, biofeedback, and barrier functions. As a relatively inelastic sac, it contributes to maintenance of normal atrial and ventricular compliance and optimal ventricular shape, provides protection against excessive ventricular-atrial valve regurgitation, and limits excessive acute dilation or mismatch between right- and left-side chamber volume.1 A small amount of pericardial fluid is usually present and has the important role of reducing friction during cardiac contraction, and mechano-receptors in the pericardium may provide biofeedback regulating heart rate and blood pressure. In addition, the negative pericardial pressure and its changes during respiratory cycles contribute to enhancing venous return to the right atrium to aid filling during inspiration.
While the diagnosis of pericardial tamponade is relatively straightforward, the differentiation between constrictive pericardial disease and restrictive physiology often presents challenges requiring the integration of hemodynamic measurements, imaging, and occasionally an endomyocardial or pericardial biopsy. In this chapter, we will review the pathophysiology of the complex pericardial-myocardial interaction leading to these different hemodynamic profiles. Chapters 38 and 44 provide additional information on pericardial interventions and on clinical profiles in pericardial disease.
NORMAL HEMODYNAMICS DURING THE RESPIRATORY CYCLE AND THE ROLE OF THE PERICARDIUM
The hemodynamic changes that occur in the setting of pericardial tamponade, pericardial constriction, and restrictive physiology can be explained on the basis of the complex interactions between cardiac chambers, pericardial restraint, and pressure gradients. The normal pericardial pressure is subatmospheric, and it tracks intrapleural pressure during the respiratory cycle. The negative pericardial pressure has the important role of maintaining a positive transmyocardial pressure gradient (intracavitary pressure – pericardial pressure), resulting in a net chamber-distending pressure that is slightly higher than the intracavitary pressure. This transmyocardial pressure gradient facilitates diastolic filling, particularly in the low-pressure right heart. During inspiration, there is a reduction in intrapleural pressure that affects all structures within the thorax, associated with a fall in chamber pressures and pulmonary wedge pressure. The reduction in intrapericardial pressure tends to be larger than the fall in systemic venous pressure, and with descent of the diaphragm, intra-abdominal pressure increases, resulting in an increase in the pressure gradient from extrathoracic veins to the right atrium. These forces collectively serve to enhance right atrial and right ventricular filling during inspiration.
Because the decrease in intrapleural pressure with inspiration is more effectively transmitted to the pulmonary venous bed than to the left ventricle, the pressure gradient from pulmonary vein to left atrium decreases slightly, resulting in a slight drop in the transmitral pressure gradient and a mild reduction of left ventricular diastolic volume and stroke volume. Hence, the mild decrease in systemic arterial pressure observed during normal inspiration is attributable to the slight reduction in left ventricular preload and transmission of the negative intrathoracic pressure to the aorta and peripheral arteries. As ventricular systole begins, the rapid reduction in ventricular volume causes a drop in pericardial pressure, which increases atrial transmyocardial gradients to further enhance atrial filling.
Because the decrease in intrapleural pressure with inspiration is more effectively transmitted to the pulmonary venous bed than to the left ventricle, the pressure gradient from pulmonary vein to left atrium decreases slightly, resulting in a slight drop in the transmitral pressure gradient and a mild reduction of left ventricular diastolic volume and stroke volume. Hence, the mild decrease in systemic arterial pressure observed during normal inspiration is attributable to the slight reduction in left ventricular preload and transmission of the negative intrathoracic pressure to the aorta and peripheral arteries. As ventricular systole begins, the rapid reduction in ventricular volume causes a drop in pericardial pressure, which increases atrial transmyocardial gradients to further enhance atrial filling.
The right and left ventricles share the intraventricular septum and are contained in the relatively indistensible pericardial sac, creating the substrate for the hemodynamic phenomenon known as diastolic ventricular interaction when the pericardium becomes diseased or when the heart becomes enlarged to the pericardial limits.2 Within the setting of this enhanced ventricular interaction, an increase in volume of one ventricle (in the setting of a compliant septum and an intact pericardium) will affect filling and volume of the other ventricle. While the pericardium has limited role in ventricular systolic coupling, it plays an important role in diastolic coupling,3 and this effect is markedly enhanced by any abnormality of the pericardium or the pericardial space. As described below, the compliance of the septum can explain at least in part the differences in ventricular interdependence observed with pericardial tamponade, pericardial constriction, and restrictive physiology.
TAMPONADE PHYSIOLOGY
Under normal conditions the pericardial sac contains 15 to 35 mL of fluid. Given the relatively inelastic characteristics of the pericardium, rapid accumulation of pericardial fluid leads to a marked increase of intrapericardial pressure. In contrast, over a prolonged period of time (as with eccentric cardiac remodeling or slowly growing malignant effusion), the pericardium can stretch and accommodate larger volumes, shifting the pressure-volume curve to the right4 (Figure 23.1). Tamponade physiology develops when the size of the effusion becomes sufficient to increase total pericardial volume from the shallow, compliant portion of the pericardial pressure-volume relationship to the steep, non-compliant portion (arrows, Figure 23.1). If venous return remains unchanged, an increase in intrapericardial pressure will decrease transmural diastolic filling pressures in all heart chambers, resulting in typical changes in the atrial pressure waveform. As described in Chapter 10, the normal atrial waveform is characterized by three positive deflections (a, c, and v waves) and three negative deflections (x, x′, and y descents). The x descent corresponds to atrial relaxation. During ventricular systole, continuous atrial relaxation and the descent of the atrioventricular valve annulus result in further reduction of atrial pressure and in the corresponding x′ descent. After the nadir of the x′ descent, the atrium fills during ventricular systole while the atrioventricular valve is closed (v wave). This phase is followed by the y descent, which corresponds to opening of the atrioventricular valve and rapid emptying of the atrium. In pericardial tamponade, as intrapericardial pressure increases, an increase in venous return will initially serve to maintain cardiac filling and prevent diastolic collapse of cardiac chambers. Further increase in pericardial pressure will lead to a progressive impairment in atrial emptying and ventricular filling, with blunting or disappearance of the y descent while the x descent is typically preserved or enhanced (Figure 23.2). There is also loss of the early dip in minimal LV diastolic pressure and equalization between right atrial and LV pressure at the onset of diastole (Figure 23.3).
As pericardial pressure continues to increase, diastolic filling pressures will equalize across the four cardiac chambers, eventually culminating in diastolic compression of the right-side and then the left-side cardiac chambers. Diastolic filling dynamics throughout the cardiac cycle essentially become a
zero-sum game—for any increase in ventricular volume there is an obligate decrease in atrial volume, and conversely when right-side filling volume increases (as during inspiration) there is a matched drop in left-side volume. This enhancement of ventricular interdependence explains the pathophysiology and most physical findings in tamponade. As described above, under normal conditions inspiration causes a decrease in intrapericardial and intrathoracic pressure, which is coupled with an increase in systemic venous return to the right heart. There is also an increase in the capacitance of the pulmonary vasculature and reduction in the pressure gradient from pulmonary vein to left atrium, which results in a decrease in left ventricular filling, stroke volume, and systolic pressure. In pericardial tamponade, these effects are magnified dramatically, and increased systemic venous return to the right side of the heart is coupled with acute reduction in leftside filling owing to reduction in the pulmonary capillary to LV diastolic gradient (Figure 23.4). The converse then occurs during expiration, when left-side filling is augmented at the cost of right heart filling—such that the diastolic volumes, stroke volumes, and accordingly developed pressures within one ventricle are 180° out of phase with those in the other ventricle (Figure 23.5). The reduction in left ventricular filling and thus stroke volume reduces aortic systolic blood pressure by >10 mmHg during inspiration (pulsus paradoxus, Figure 23.6). As stated above, this is really not a paradox but an exaggeration of the normal systemic pressure changes during respiration.
zero-sum game—for any increase in ventricular volume there is an obligate decrease in atrial volume, and conversely when right-side filling volume increases (as during inspiration) there is a matched drop in left-side volume. This enhancement of ventricular interdependence explains the pathophysiology and most physical findings in tamponade. As described above, under normal conditions inspiration causes a decrease in intrapericardial and intrathoracic pressure, which is coupled with an increase in systemic venous return to the right heart. There is also an increase in the capacitance of the pulmonary vasculature and reduction in the pressure gradient from pulmonary vein to left atrium, which results in a decrease in left ventricular filling, stroke volume, and systolic pressure. In pericardial tamponade, these effects are magnified dramatically, and increased systemic venous return to the right side of the heart is coupled with acute reduction in leftside filling owing to reduction in the pulmonary capillary to LV diastolic gradient (Figure 23.4). The converse then occurs during expiration, when left-side filling is augmented at the cost of right heart filling—such that the diastolic volumes, stroke volumes, and accordingly developed pressures within one ventricle are 180° out of phase with those in the other ventricle (Figure 23.5). The reduction in left ventricular filling and thus stroke volume reduces aortic systolic blood pressure by >10 mmHg during inspiration (pulsus paradoxus, Figure 23.6). As stated above, this is really not a paradox but an exaggeration of the normal systemic pressure changes during respiration.
Cardiac tamponade is a clinical diagnosis based upon typical symptoms including fatigue, dyspnea, and air hunger together with physical findings including elevated venous pressure, sinus tachycardia, and pulsus paradoxus. The diagnosis can be confirmed by echocardiography which typically shows pericardial effusion, right atrial and right ventricular diastolic collapse, abnormal increase in blood flow velocity across the tricuspid valve and corresponding decrease in flow velocity across the mitral valve during inspiration (Figure 23.7), and dilated inferior vena
cava without collapse during inspiration. Confirmation of tamponade physiology is obtained through hemodynamic measurements during cardiac catheterization and through documentation of elevation of pericardial pressure above atmospheric pressure, its equalization with right atrial pressure, and its decrease to subatmospheric levels following pericardiocentesis. Detailed understanding of the hemodynamic changes in tamponade and a high index of suspicion are especially important in the evolving era of advanced percutaneous treatments for structural heart disease and cardiac arrhythmias, where cardiac perforations may be more commonly observed.
cava without collapse during inspiration. Confirmation of tamponade physiology is obtained through hemodynamic measurements during cardiac catheterization and through documentation of elevation of pericardial pressure above atmospheric pressure, its equalization with right atrial pressure, and its decrease to subatmospheric levels following pericardiocentesis. Detailed understanding of the hemodynamic changes in tamponade and a high index of suspicion are especially important in the evolving era of advanced percutaneous treatments for structural heart disease and cardiac arrhythmias, where cardiac perforations may be more commonly observed.
Constrictive-Effusive Physiology
In rare cases, following pericardiocentesis the pericardial pressure returns to subatmospheric (<0 mmHg) levels, while the right atrial pressure and the right ventricular diastolic pressures remain elevated, with a typical dip and plateau pattern in the right ventricular pressure tracing (Figure 23.2).5,6 This pattern is consistent with effusive-constrictive physiology, and it has been suggested that this represents evolution of acute pericarditis with pericardial effusion toward pericardial constriction.5, 6, 7
Low-Pressure Tamponade
A clinical syndrome characterized by low pericardial pressure leading to cardiac compression because of low filling pressures, without the typical clinical findings associated with tamponade, has also been described.8, 9, 10, 11, 12 In an analysis of 279 patients undergoing pericardiocentesis at a single institution, 29 patients met the criteria for low-pressure tamponade (defined as intrapericardial pressure of < 7 mmHg before pericardiocentesis and right atrial pressure of <4 mmHg after pericardiocentesis, Group 1), whereas 114 patients met the criteria for classic tamponade (intrapericardial pressure of >7 mmHg before pericardiocentesis and right atrial pressure of ≥4 mmHg after pericardiocentesis, Group 2).12 Typical findings of tamponade were present in only 24% of patients in group 1, as compared to 71% in group 2. When compared with classic tamponade cases, patients with low-pressure tamponade had lower pulsus paradoxus
(10.41 ± 5.33 versus 23.7 ± 12.47 mmHg), lower intraperi-cardial pressure (2.5 ± 1.68 versus 12.93 ± 5.42 mmHg), and lower right atrial pressure (3.59 ±1.57 versus 14.63 ± 5.42 mmHg), but similar transmural atrial pressure (0.07 ± 0.6 versus 0.35 ± 1.70 mmHg) and similar cardiac index (2.96 ± 1.26 versus 2.69 ± 1.79 L/min). Both patient groups had a significant increase in cardiac index following pericardiocentesis. Overall, these data support the importance of transmural or transmyocardial pressure as the primary determinant of atrial filling and highlight the importance of a high degree of suspicion in patients who might have symptoms or findings consistent with hemodynamic compromise and in whom classic findings of tamponade might be absent.
(10.41 ± 5.33 versus 23.7 ± 12.47 mmHg), lower intraperi-cardial pressure (2.5 ± 1.68 versus 12.93 ± 5.42 mmHg), and lower right atrial pressure (3.59 ±1.57 versus 14.63 ± 5.42 mmHg), but similar transmural atrial pressure (0.07 ± 0.6 versus 0.35 ± 1.70 mmHg) and similar cardiac index (2.96 ± 1.26 versus 2.69 ± 1.79 L/min). Both patient groups had a significant increase in cardiac index following pericardiocentesis. Overall, these data support the importance of transmural or transmyocardial pressure as the primary determinant of atrial filling and highlight the importance of a high degree of suspicion in patients who might have symptoms or findings consistent with hemodynamic compromise and in whom classic findings of tamponade might be absent.
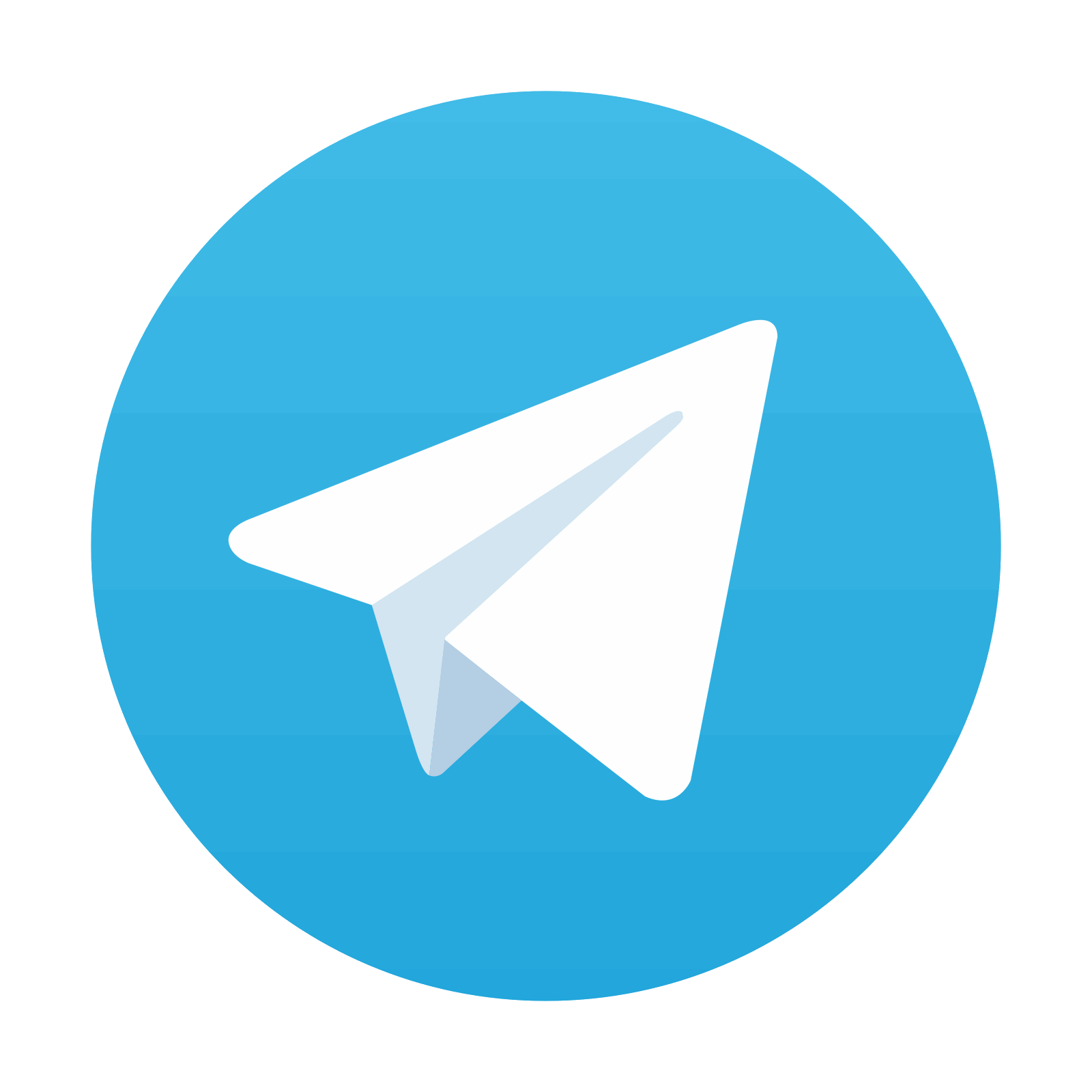
Stay updated, free articles. Join our Telegram channel

Full access? Get Clinical Tree
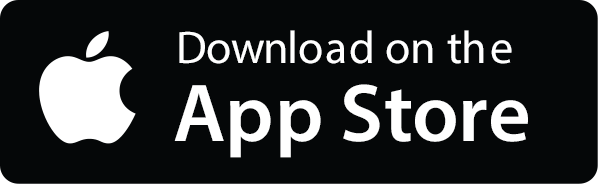
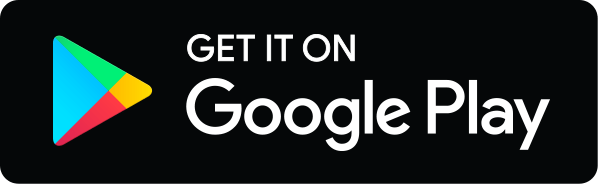