Chapter 46 Endovascular Approach to Vascular Trauma
The past decade has witnessed the near complete adoption of endovascular techniques as alternative therapies for management of vascular disease in virtually all vascular beds. With advanced training paradigms for practicing vascular surgeons and incorporation of catheter skills at every level of the experience for doctors in training, endovascular therapy has permeated all aspects of the contemporary vascular practice. Clinicians find themselves faced with the real problem of creating training strategies that provide sufficient open surgical experience for the next generation of vascular surgeons.1
Endovascular management of trauma is gaining acceptance. The volume of endovascular repairs of arterial trauma as identified by the National Trauma Data Bank increased twenty-sevenfold between 1997 and 2003. However, open surgical management remains vastly more common for this patient group. The overall utilization rate of endovascular interventions during this time period was only 3.7%.2 In most other areas of vascular surgical practice, when endovascular alternatives exist and can be determined to be safe, effective, and durable, open surgery is reserved for failures of endovascular therapy.
An additional paradigm shift that is occurring naturally is the transition of endovascular procedures from the interventional radiology suite to the hybrid operating room (OR), equipped with state-of-the-art imaging, racks of endovascular tools and the potential for concomitant or staged open surgical procedures. Previously, only hemodynamically stable patients were deemed eligible for angiography, with injury severity score (ISS) for patients undergoing endovascular interventions significantly lower than that of patients undergoing open surgery for arterial injuries (13 vs. 20; p < 0.001).2 With these minimally invasive techniques being more universally available in the same room as the multidisciplinary trauma team, the OR may prove to be where endovascular therapy reaches its true potential in helping to treat vascular injury in the polytrauma patient.
Developing an Endovascular Trauma Program
Tools
Closure Devices
In practice, closure devices are used routinely to reduce access site complications and provide for secure hemostasis and earlier ambulation. For access larger than 8 French, use a “preclose” technique with one or two Proglide suture closure devices (Abbott Vascular, Redwood City, Calif.) or the larger Prostar suture closure device (Abbott Vascular, Redwood City, Calif.).3 Brachial artery access is usually performed using a 4-French sheath. Use of percutaneous closure devices in the brachial artery, while not standard therapy, is possible4 and occasionally indicated. Starclose (Abbott Vascular, Redwood City, Calif.), an extraarterial nitinol clip, has been used on the brachial artery with satisfactory results.3,4 Successful use of the preclose technique has been described for 14-French venous access as well; it is noteworthy that venous backbleeding from the marker port is less brisk than with arterial access and can be augmented with a Valsalva maneuver. Valsalva maneuver can be also be used effectively to validate the integrity of the closure.5
Balloon Catheters
Balloon catheters have two principal applications in trauma: angioplasty of stents or stent grafts in order to ensure their adequate molding to the vessel wall, and temporary occlusion of blood flow for hemorrhage control. In the case of the former, balloons of 5 to 14 mm in diameter, 60 to 80 mm in length, and on 80- and 135-cm long catheters are most frequently used. The aortic occlusion balloon, as described later, is used to maintain cerebral and coronary perfusion in the hemodynamically unstable patient and requires large-bore access. Examples of available occlusion balloons are listed in Table 46-1.
Stent Grafts
Fabric-covered stents include:
• The Wallgraft (Boston Scientific) self-expanding stent covered with Dacron polyester, with pullback deployment system
• Viabahn (WL Gore) self-expanding nitinol exoskeleton covered with thin polytetrafluoroethylene (PTFE) with pull-string deployment
• Fluency (Bard, Tempe, Ariz.) self-expanding nitinol with PTFE
• iCast (Atrium medical corporation, Hudson, N.H.) balloon-expandable stainless-steel stent with an encapsulated cover made of expanded PTFE
Embolization Elements
Hemorrhage control and vascular occlusion are typically achieved with embolization using coils. Alternative embolization techniques using polyvinyl alcohol (PVA), Gelfoam (Upjohn, Kalamazoo, Mich.) or acrylic copolymer microspheres such as Embospheres and EmboGold (Biosphere Medical, Rockland, Mass.)6 are not routinely used in patients with active extravasation. Coils come in standard size configurations, such as the platinum-based 0.035-inch Tornado coils (Cook, Bloomington, Ind.) or variable size configurations found in the Vortex coil (Boston Scientific), available in 0.018-, 0.021-, and 0.038-inch diameters.
Initial Evaluation: Rethinking the Trauma Algorithm
In the traditional trauma paradigm, the unstable patient or patient in extremis will often not survive to undergo evaluation by a vascular surgeon, being managed by standard open surgical techniques in a trauma bay or OR with limited imaging or endovascular capabilities. Exsanguination from noncompressible injury to the torso remains a major cause of death in both military and civilian trauma. The use of endovascular techniques to affect these potentially preventable mortalities has been targeted by the U.S. Department of Defense as an important area for clinical research.7 After patent airway and breathing are established, the critically ill hypotensive patient may require emergent thoracotomy for proximal control of the aorta before rapid transfer to the OR for emergent laparotomy or thoracotomy to address injury. A growing body of experimental8,9 and clinical10,11 evidence supports the placement of an aortic occlusion balloon rather than thoracotomy. Emergency department thoracotomy for penetrating trauma has a survival rate of 8.8%; the procedure, when performed for blunt trauma, has an even more dismal record with survival of 1.4%.12 Clearly an improved approach is warranted. It is common practice to perform all trauma surgery in a setting that provides as many therapeutic options as possible for patients with potential vascular injuries. By using a hybrid operating suite with advanced imaging, anesthesia support and appropriate trauma personnel involvement, it is possible to simultaneously stabilize, image, and treat many injuries with endovascular techniques or a combination of open and catheter-based surgical alternatives. For example, patients with hypotension can undergo placement of an aortic occlusion balloon under fluoroscopic guidance before induction of general anesthesia, which can often precipitate arrest because of vasodilatory effects of agents used during induction.7,12
Technique
A micropuncture system is used to gain access to the vascular system using ultrasound guidance and is then upsized to a 6-French introducer sheath. A 0.035-inch, 260-cm angled Glidewire is then passed into the thoracic aorta under fluoroscopy. Angiographic visualization of the arch anatomy is performed using a pigtail catheter and power injector, if indicated. A Proglide closure device is used to preclose the artery; a 10-cm, 6-French sheath is placed; and an angled Glidecath advanced into the thoracic aorta. The wire is exchanged for an Amplatz wire, and a 12-French sheath is advanced under fluoroscopy. The aortic occlusion balloon is inflated in the descending thoracic aorta13 until hemodynamics improve with blood products and crystalloid resuscitation. The balloon is then deflated and removed, and additional diagnostic and therapeutic interventions are performed. Alternatively, the contralateral groin is accessed with a 6-French sheath for diagnostic and therapeutic catheter placement, and the aortic occlusion balloon is left in place for intermittent inflation or partial deflation as needed. The Gore Dryseal sheath allows multiple cannulations with devices of varying diameters while limiting leakage from the hemostatic valve, thereby decreasing the need for multiple access sites. When using this technique, however, the fluid-filled valve may decrease the maneuverability of the therapeutic catheter placed alongside the occlusion balloon, complicating the ability to selectively cannulate branch vessels.
Management of Specific Injuries
Physical examination or radiographic imaging will inform the decision to perform arteriography of certain anatomic areas. Table 46-2 summarizes methods of contrast arteriography.
Carotid and Vertebral Artery
The reported incidence of carotid injuries varies widely, ranging between 0.3% and 20%.14 Blunt trauma to the carotid occurs at 0.1% to 0.45%, with mortality rates as high as 20% to 40%.15 Penetrating carotid injuries account for approximately 3% of injuries.16 Vertebral artery injuries are much less common because of bony protection. Clinical findings that support diagnostic workup include mandibular or facial fractures, C2-C3 fractures, neck contusion or laceration, neck hematoma, and mechanism of injury (deceleration). Injuries constitute transection, dissection, thrombosis, pseudoaneurysm, and arteriovenous fistula (AVF) leading to stroke and death.17 Cervical injuries are initially classified according to their location along the neck in order to facilitate decision-making. Anatomic zones in the neck were initially described by Monson and colleagues18 and have implications for management of cervical vascular injuries: guiding traditional open surgical alternatives for achieving proximal and distal vascular control. Zone I is located below the cricoid cartilage, zone II is between the cricoid cartilage and angle of the mandible, and zone III injuries occur above the angle of the mandible. Penetrating zone II trauma with hard signs, such as bleeding, expanding hematoma, or loss of carotid pulses and acute neurologic deficits have traditionally mandated immediate surgical exploration without further studies. Classically, proximal control in zone I injuries would require a median sternotomy. For patients with an injury in zone III, distal vascular control is difficult without subluxation of the mandible. However, classical limitations in vascular control have evolved with current endovascular solutions. Balloon occlusion of the proximal cervical vessels in the chest can be accomplished via femoral access. Similarly, proximal control can be obtained in zone III injuries with compliant balloons placed in the proximal internal carotid or vertebral arteries. This also provides the opportunity to obtain imaging to better define and potentially manage the cervical vascular injury endovascularly.
Duplex ultrasound can identify dissection as well as pseudoaneurysm. Any abnormal findings, such as turbulent flow, reduced flow velocity, absence of flow, or presence of an echolucent intraluminal lesion, should lower the threshold for further investigation with angiography. CTA has been used for penetrating injuries of the neck, with a 75% sensitivity to diagnose venous vascular injury.19,20 However, digital subtraction angiography remains the gold standard for carotid artery injuries and offers the opportunity for endovascular therapy. Traumatic carotid lesions amenable to endovascular treatment are intimal dissection, pseudoaneurysm, and AVF.21–24 External carotid artery injuries have been managed safely with endovascular ligation using coils, N-butyl 2-cyanoacrylate embolization and balloon occlusion for endovascular ligation.17 Similarly, vertebral artery injuries may be amenable to coiling and balloon occlusion as well as with covered stent deployment, allowing for maintenance of inline flow.25
Treatment options for carotid dissections with intact distal flow include surgical repair, endovascular stenting, or observation with repeat diagnostic studies 1 to 2 weeks after the injury. Systemic heparinization in patients without any contraindications is typically indicated and may be all that is required.16 Open surgical approaches are rare. Uncovered stents may be placed to treat short lesions.26,27 If the site of entry is identified, an attempt at placement can be considered for even more extended dissections if anticoagulation is contraindicated.
Pseudoaneurysms can result from direct injury or as a sequelae of a maturing dissection. When large, they can expand and cause compressive symptoms, requiring operation or embolization.28,29 Surgical intervention can be complex, especially for lesions near the skull base, where temporomandibular joint subluxation or vertical ramus osteotomy may be required to obtain adequate exposure. Ligation of the carotid artery carries an associated mortality of up to 44% and neurologic deficit in 100%.30 Restoration of flow with open repair of the internal and common carotid arteries is associated with persistent neurologic deficit in up to 30% of cases.30 Covered stents have been used to exclude pseudoaneurysms. Coils have also been used to treat these lesions and, in some cases, both have been applied.28,29,31 The major risk in treating a pseudoaneurysm in the carotid artery is distal embolization and stroke; however, data on the use of distal protection devices are limited. Stent grafts were placed successfully in 19 patients with penetrating injuries (10 pseudoaneurysms, 9 AVFs). At follow-up, ranging from 1 to 56 months, 14% had stent graft occlusion with one associated stroke.32 A literature review of stent-graft placement indicates a 15% postoperative occlusion rate.33 When stent-graft placement is planned, premedication is done with antiplatelet agents, preferably clopidogrel and aspirin. Traumatic carotid lesions pose a unique challenge with acute, possibly unstable thrombus susceptible to embolization during wire manipulation. Although no specific data exist for it, the use of an embolic protection device may reduce the potential for embolic complications.
Technique
Proximal injuries may require direct cervical exposure for a retrograde approach with the role of the distal protection device played by an internal carotid artery clamp. However, most injuries are approachable from the femoral artery. A 5-French, 90-cm Omniflush catheter is placed in the ascending aorta, and a left anterior oblique aortogram is taken to demonstrate the ostia of the arch vessels. Based on this image, an end-hole catheter is selected for cannulation of the common carotid artery. In uncomplicated arch anatomy, the 5-French, 100-cm angled Glidecath is used. Once the ostium of the carotid artery is engaged, 2 mL of contrast is injected to corroborate position. The 0.035-inch Glidewire is advanced gently into the target artery, and the angled catheter is advanced, with particular care taken not to advance the wire too distally into the internal carotid artery. Carotid angiography is performed in anteroposterior (AP) and lateral positions. Additional oblique views may be needed to properly assess the anatomy and extent of injury. The intracranial circulation is also evaluated with AP and lateral views. Up to 30% of blunt carotid injuries are bilateral, mandating a complete four-vessel angiogram. Once the injury has been defined, the wire is exchanged for the 260-cm Amplatz wire; a 6-French, 90-cm–long Destination sheath (Terumo, Somerset, N.J.) is placed with its tip above the clavicle. A distal embolization protection filter may be placed. Self-expanding bare metal stents or a Viabahn-covered self-expanding stent may be advanced directly over the 0.014-inch filter wire. If coil embolization of pseudoaneurysms is performed in conjunction with distal embolization protection, a 7-French Destination sheath may be used to facilitate advancement of both the filter wire and a 45-French catheter into the common carotid artery. Endovascular occlusion of the severely damaged carotid or vertebral artery has been described. Glue, microbeads, or other liquid agents such as N-butyl 2-cyanoacrylate are not typically used in the common or internal carotid artery, but have been used in the external carotid. Amplatzer plug placement has also been described. The preferred method for hemorrhage control for injury to branches of the external carotid artery uses a 6-French sheath, a 4-French Glidecath, and 0.035-inch embolic coils. Vertebral artery injuries can be treated similarly. Figure 46-1A to C shows management of a traumatic arteriovenous fistula from the vertebral artery to the internal jugular vein treated with placement of a 5 mm × 2.5 cm covered stent.
Innominate Artery and the Descending Thoracic Aorta
Thoracic Aorta
Only 15% of patients with severe blunt thoracic aortic trauma survive to reach the hospital. Only 50% of those who reach the hospital will ultimately survive the aortic injury.34 Typically, patients who survive the initial injury have a contained rupture with tissue tamponade and a hematoma that is constrained. When patients convert to free rupture, the mortality rate is 100%. The mechanism of injury involves stress, applied to transition points between fixed and relatively mobile segments of the aorta. These forces are compounded by sudden internal changes in blood pressure and pulse pressure. Aortic injury of this kind is most often due to a motor vehicle crash (MVC) or a fall, with attendant sudden deceleration. In patients with contained rupture, control of blood pressure and the resultant reduction in sheer forces associated with hypertension and tachycardia is important. Administration of β-blockers, including permissive hypotension, is useful in the initial management of patients with aortic injury and normal or hyperdynamic hemodynamics. The minority of patients (15%-19%) who survive the initial injury and period of transport to the hospital are in various stages of shock because of other concurrent injuries, with systolic blood pressures ranging from 50% to 98% of normal.35,36 Both hypotension and hypothermia (body temperature <35°C) are associated with early mortality, with an adjusted odds ratio of 8.54.35 Major factors contributing to increased mortality include the severity of associated injuries and the timing of repair. The primary initial management of blunt aortic injuries requires early and efficient resuscitation and the treatment of associated injuries.37,38 Some centers have moved to delayed endovascular repair of stable (without contrast extravasation) blunt aortic injuries in order to treat associated injuries first.39,40
The mean ISS of traumatic thoracic aortic transections is 39.41 Associated injuries, including head injury, pulmonary contusion, long bone injury, and solid organ injury, occur in 90% of blunt aortic traumas. The abdomen and chest have the highest associated injury severity score.41 Open surgical management of thoracic aortic trauma is complicated by the physiologic stresses associated with single lung ventilation and aortic cross-clamping. Open repair is associated with a mortality rate of 15% to 40% and resultant paraplegia rates of 8% to 20%.
Endovascular management for these injuries has the potential to mitigate some of the risks associated with open surgical alternatives. Thirty-day mortality rates associated with endovascular repair of thoracic aortic injuries have been reported as high as 8.3% with an associated 1-year mortality of 14.4%.41 Major adverse events associated with endovascular repair include, stroke (10%), respiratory failure (3.3%), and paralysis or paraparesis (1.7%) at 30 days with no additional events recorded at 1-year follow-up.
The early reports of endovascular repair of blunt aortic injury described the use of endovascular cuffs that were designed for proximal extension of infrarenal aortic endografts.42–44 Currently, appropriately sized thoracic stent-grafts are available for traumatic thoracic aortic injuries made by a number of manufacturers including the Talent graft (Medtronic, Minneapolis, Minn.), the TAG graft (an acronym for thoracic aortic graft; WL Gore), the TX2 graft (Cook, Bloomington, Ind.) and the AneuRx graft (Medtronic, Minneapolis, Minn.). More commonly available grafts, sized for thoracic aortic aneurysm repair, are often too large for the normal-sized aorta of the typical trauma patient. Careful sizing is essential to provide for a satisfactory seal and to prevent infolding.
Technique.
General anesthesia is standard. Placement of a spinal catheter is useful for pressure reduction by spinal fluid drainage in patients exhibiting signs of spinal cord ischemia perioperatively. Given the larger size of endovascular devices designed for thoracic aortic intervention, open exposure of the external iliac arteries may be required to facilitate device delivery and closure. Alternatively, common femoral artery cutdown or percutaneous access using the previously described preclose technique may be performed.42 An arch aortogram is performed using a marker pigtail catheter. If left subclavian artery coverage is required to obtain a proximal landing zone of 2 cm, the left subclavian artery may be occluded proximal to the vertebral artery, through left brachial artery access using an Amplatzer plug or coils. Retrospective reviews of blunt thoracic injuries have reported subclavian artery coverage rates ranging from 0% to 80%.43 The issue of carotid-subclavian bypass is controversial. Bypass should be performed for patients with a dominant left vertebral artery, a blind posterior inferior cerebellar artery, or a left internal mammary artery–left anterior descending coronary artery bypass.44 If more arch is required for an adequate landing zone, then a carotid to carotid bypass can be performed for further debranching.
The major challenge of endograft placement is sizing. Most companies recommend 10% to 15% oversizing of the endograft compared to the native aortic diameter either measured from inner lumen to inner lumen (WL Gore) or outer adventitia to adventitia (Medtronic). In otherwise normal aortas without dilatation or aneurysmal deterioration, the aortic diameter is generally 18 mm. Oversizing can jeopardize both the proximal and distal seal zones secondary to pleating and in-folding of the stent graft; this can lead to displacement and slippage of the stent graft, compromise of luminal flow, or “bird-beaking” of the proximal graft, with resultant endoleak and device fracture. Therefore accurate sizing of the stent-graft to aortic diameter is required. Accurate sizing may be compromised by hypovolemia and an associated reduction of aortic diameter by 30%.45
Significant morbidity and death have been reported in endovascular repair of thoracic aortic transection associated with iliac artery complications. These injuries might not be recognized until sheath removal (Figure 46-2A). In patients with iliac arteries less than 8 mm in diameter (24 French), whether due to spasm in the young or atherosclerotic disease in the older patient, retroperitoneal exposure of the abdominal aorta or left common iliac artery, with a plan for either direct cannulation or creation of a prosthetic conduit through which the endograft may be delivered, is a safe option.
Innominate Artery
Injury from blunt trauma to thoracic arch branches is rare, encompassing 5% of traumatic vascular injuries, a rate significantly lower than that for penetrating injuries in this region. However, the incidence is likely underestimated because of the high mortality associated with arch injury in the field (80%-90%). The innominate artery accounts for half of all arch vessel injuries, with a mortality ranging from 0% to 24%. The most common mechanism of injury is MVC (88.9%), followed by crush (8.9%) and fall (2.2%). Head-on collisions constitute the majority of MVC-associated innominate artery injuries (72%), followed by side (24%) and rear impacts (4%) at relative velocities greater than 60 mph or with a change in velocity of greater than or equal to 20 mph. Chest compression increases the intramediastinal pressure between the sternoclavicular joint and the vertebral column, pushing the heart posteriorly and to the left to stretch the aortic branches. Hyperextension of the cervical spine and lateral rotation of the head serve to maximize the shear stress at the origin of innominate artery. Key clinical indicators of injury include the seatbelt sign (see Figure 46-2B) and steering wheel imprint as well as dyspnea, dysphagia, interscapular and neck pain, absent upper extremity pulses, neurologic deficit, acute superior vena cava syndrome, pseudocoarctation, presence of a bruit from an arteriovenous fistula, and congestive heart failure. Diagnosis is suspected with widened mediastinum, rib fractures, tracheal or esophageal deviation, and right hemothorax or pneumothorax on chest radiography. The diagnosis can be made on chest CTA, angiography, transesophageal echocardiography (TEE; 86%-92% sensitive), magnetic resonance angiography, and intravascular ultrasound.15,46–53 Innominate artery injuries are most common at the orifice (81.8%), followed by the distal (9.1%) and midpoint (4.5%) of the vessel, with 2.3% of injuries occurring at two separate parts of the artery. These injuries encompass intimal tears (70%), pseudoaneurysms, and rupture. After efficient and aggressive resuscitation to normotension through femoral venous access, open and endovascular options for repair are considered. The decision for open repair is often predicated on the existence of concomitant injuries mandating thoracic exploration via median sternotomy or trap door thoracotomy. These approaches are inherently morbid, with significant risk of neurologic and respiratory injury.54–57 In cases without significant related injuries in proximity requiring exploration, endovascular repair can be accomplished with femoral access distant from the field of injury, with less pain, fewer respiratory and neurologic complications, and decreased length of stay.58,59 Dissection or intimal flap in the innominate artery can be treated with a bare metal or covered self-expanding stent. Pseudoaneurysm and transection or rupture are treated with covered stents. The primary endovascular limitation is the lack of adequate distal landing zone before the artery bifurcates. If necessary, the right subclavian artery can be excluded by the stent-graft with performance of a carotid-subclavian artery bypass, if needed for right arm ischemia. Premedication with clopidogrel and aspirin is ideal when the patient’s condition permits, and is continued indefinitely for patients receiving endovascular implants in this location.60 Although there is a paucity of data on the use of embolic protection devices in traumatic innominate artery injury, it is common to use a device placed within the distal common carotid artery during stent advancement and deployment.
Technique.
Percutaneous common femoral artery access is obtained, and arch arteriography is completed using the pigtail catheter. The innominate artery can be cannulated with an angled Glidecath in a type I arch. A type II or III arch may require a Simmons 1 or 2 Glidecath. A 0.035-inch Glidewire is carefully advanced to the lesion, taking care not displace an injury-associated soft thrombus. The wire is exchanged for the Amplatz wire, and the 90-cm, 6-French sheath is advanced to the innominate artery, as space permits, proximal to the lesion. An embolic protection device is then advanced through the lesion and deployed in the distal common carotid artery. A self-expanding stent can be deployed over the filter wire. A covered stent-graft, routinely oversized by no more than 10% for the normal artery, will usually require a 9- to 12-French sheath for diameters greater than 8.5 mm. Postdeployment balloon angioplasty is performed with a compliant balloon, selected to match the vessel diameter (see Figure 46-2C to E).
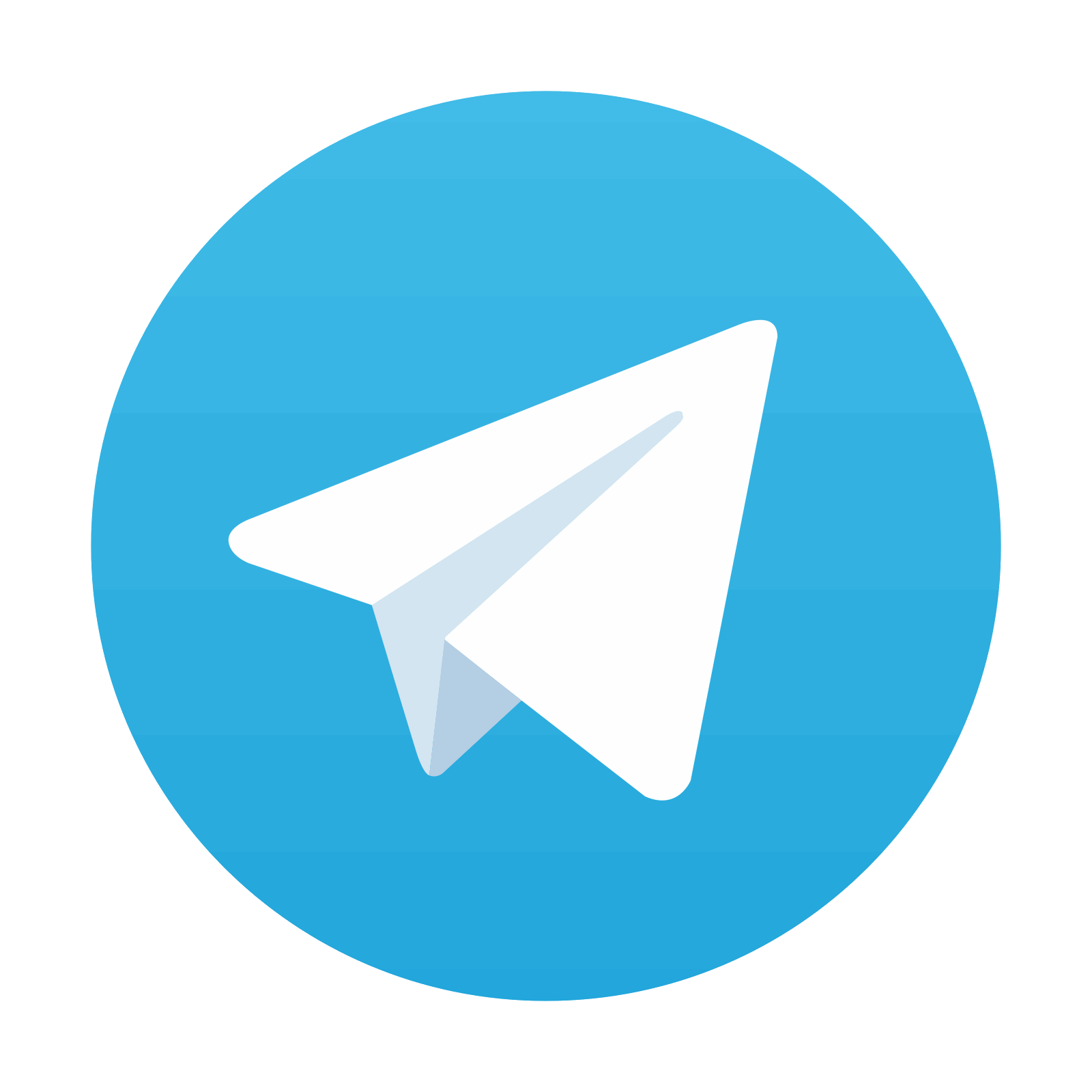
Stay updated, free articles. Join our Telegram channel

Full access? Get Clinical Tree
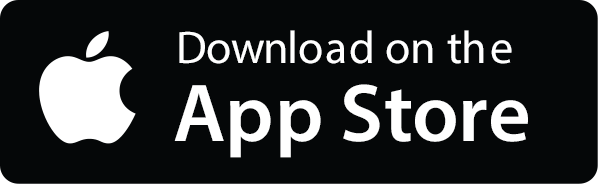
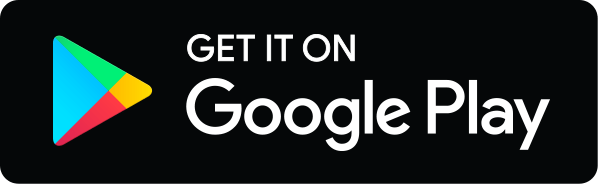