CHAPTER 6 Endoscopic Therapies for Thoracic Diseases
The first recorded bronchoscopic intervention is attributed to Gustav Killian, who in 1897 removed a piece of pork bone from the right main-stem bronchus with an esophagoscope.1 A few decades later, Chevalier Jackson was instrumental in the advancement of the field of rigid endoscopy of the airway and esophagus.1 Advancements in endoscopic technology have led to safer and more effective alternatives to surgery in nonoperative candidates. This chapter focuses on endoscopic esophageal and bronchial interventions, such as photodynamic therapy, radiofrequency ablation, stenting, laser ablation, and brachytherapy. Evolving endoscopic procedures for benign esophageal diseases (Zenker’s diverticulum and gastroesophageal reflux) are also discussed.
PHOTODYNAMIC THERAPY
Technique
The first step in PDT is the administration of the photosensitizer. Porfimer sodium (1.5 to 2.0 mg/kg) and m-THPC are injected intravenously; 5-ALA is given orally. Despite an improved side effect profile and increased tumor specificity, 5-ALA has yet to gain widespread clinical acceptance and has limited applications in thoracic surgery.2,3 At the current oral dosage, 5-ALA PDT produces necrosis only to a depth of 1 mm. Higher 5-ALA doses cannot be used because of severe side effects (e.g., nausea, vomiting, transient elevation of liver enzymes). On the other hand, m-THPC is 100 times more photoactive than porfimer sodium and has a shorter elimination half-life; however, it is not yet approved for clinical use in the United States.
Our preference has been to use conscious sedation and flexible endoscopy in most cases. A cylindrical diffuser fiber is used to deliver light therapy to the tumor. The sizes used are 1 cm, 2.5 cm, and 5 cm (Fig. 6-1). The diffuser fiber is placed alongside the tumor. If this is not possible because of occlusion of the lumen, the tumor can be impaled with the probe. Multiple light illumination cycles may be needed, depending on the length of the tumor relative to the length of the probe. The diffuser must be positioned to minimize light delivery to normal tissues. Endoscopy is repeated at 48 hours and sometimes a third time after another 48 hours. During the repeated endoscopic procedures, necrotic debris is removed by irrigation and suction. Usually, further light treatments are delivered to the tumor at the time of repeated endoscopy.
Lung Cancer
Curative Intent
PDT is approved for the treatment of small proximal endobronchial tumors. These small central tumors are rare, although some centers with active early screening programs, such as sputum cytology or light-induced fluorescence bronchoscopy, may encounter these more frequently.4 The results of PDT for these small lesions should be compared with the results of surgical resection. The central location of these tumors may necessitate complex airway reconstructions. PDT may be a suitable alternative to resection in patients who are not candidates for surgery. In most cases, these small endobronchial tumors are occult to standard radiologic imaging, such as chest radiography, computed tomography, and positron emission tomography scanning. Assessment of response is difficult and requires bronchoscopic evaluation. It is unclear whether a complete response is the result of tumor eradication or merely reflects the limitations of surveillance techniques. Superficially spreading tumors (<3 cm2) and small endobronchial tumors (<1 cm2) are best suited for PDT. Initial complete response can be expected in 65% (<3 cm2) to 85% (<1 cm2) of superficial cancers and in 30% (>0.5 cm nodule) to 92% (<0.5 cm nodule) of nodular cancers.5,6 Long-term response rates (i.e., >1 year) vary between 30% and 80%.5–9 At 5 years of follow-up, complete response rates of up to 29% to 66% have been reported.5,7
In a study of 21 operative candidates treated initially with two courses of PDT, 52% (11 of 21) were free of tumor at 1 year.7 Curative PDT failed in the remaining 48% (10 of 21), and they were offered surgery; 8 consented. Of the 11 responders, 9 (9 of 21; 43%) did not require surgery (mean follow-up, 68 months), and 2 were operated on for a second primary lung cancer. It is noteworthy that of the patients who eventually underwent resection, 30% were found to have N1 disease, supporting the role of resection, when possible. Our policy has been to reserve PDT for patients with significant comorbid factors or those who refuse pulmonary resection. In this group of patients, PDT is an excellent modality with an expected 1-year survival of 80%.10 In our initial experience of treating 10 superficial cancers, a complete response was seen in 70% at a follow-up of 30 months.8 In other prospective series, 80% to 93% of nonsurgical candidates were alive at 5 years of follow-up.5,11
Palliative Intent
A cohort of 175 patients with endobronchial non–small cell lung cancer treated with PDT was observed prospectively for a period of 14 years.11 Most patients had squamous cell carcinoma (89.3%), and treatment by conventional modalities, such as chemotherapy, radiotherapy, laser ablation, and surgery, had failed in 73.1%. The mean number of treatments was 2.8 per patient. Stage had the most significant impact on survival after multivariate analysis. Poor performance status (i.e., Karnofsky performance status < 50) had a negative impact on outcome only in advanced-stage tumors (IIIa-IV). However, patients with a low Karnofsky performance status (<50) secondary to pulmonary symptoms still derived benefit from PDT. Prolonged survival (12 to 37 months) was observed in 66% of stage IIIa-IV patients with poor performance. The median survival for the entire group was 7 months. Of the 44 patients with stage IV disease, 21% survived 12 months or longer.
Our initial experience with palliative PDT involved 44 lung cancer patients with symptoms related to obstruction and hemoptysis.10 Thirteen patients required a second treatment course at a mean of 2.3 months for recurrent symptoms. There were no fatalities, and 82% of treatments were performed without complications. Hemoptysis was effectively palliated in 90% of treatment courses. Obstruction was successfully palliated in 59% of treatments. Median survival was 2.3 months. PDT is also effective in palliating hemoptysis and dyspnea in patients with endobronchial metastases from nonpulmonary malignant neoplasms.12 An example of a patient who was treated for a complete lobar obstruction is demonstrated in Figures 6-2 through 6-6.
In a randomized prospective study, PDT was compared with standard laser techniques for inoperable non–small cell lung cancer.13 The bronchoscopic response rate (PDT, 38.5%; laser, 23.5%) and symptomatic improvement at 1 month were equivalent in each group. PDT provided significantly longer lasting relief (50 days versus 38 days) and improved median survival (265 days versus 95 days) compared with laser resection. However, this finding may be explained in part by the lower proportion of advanced-stage tumors in the PDT group.
In general, PDT is well tolerated with minimal morbidity. In the first 77 treatments that we performed for endobronchial disease, 82% were uneventful. Complications included respiratory failure (7.8%), pleural effusion (1.3%), and sunburn (5.2%). All bright light and sunlight exposure must be avoided initially. Patients remain photosensitive for a period of 4 to 8 weeks, after which they can be gradually reexposed to sunlight.14,15 Inadvertent direct sunlight exposure has been reported to cause first- and second-degree burns in up to 10% of patients.35 The restrictions imposed by photosensitivity and their potential impact on quality of life should be considered before treatment. Immediate postprocedure mortality for lung and esophageal PDT (30-day) ranges from 2.6% to 7.1%.11,13–15
Barrett’s Esophagus and Esophageal Cancer
Curative Intent
The management of high-grade dysplasia associated with Barrett’s esophagus is controversial. Most surgeons advocate esophagectomy because of the associated risks of occult malignant disease and lymph node metastases.16 On the other hand, concerns related to the morbidity and mortality of esophagectomy have led some clinicians to advocate alternative approaches, such as surveillance or mucosal ablation.17 In our experience, we have reported good results with a minimally invasive esophageal resection for patients with Barrett’s esophagus and high-grade dysplasia.18,19 Mucosal ablative methods include laser ablation, argon plasma coagulation, radiofrequency ablation, and PDT.20–23 All these techniques attempt to eradicate Barrett’s epithelium with the hope that it will be replaced by squamous epithelium. Unfortunately, in some instances, islands of columnar epithelium may recur under a normal-appearing squamous epithelial layer.24 This problem is addressed by other endoscopic techniques, such as endoscopic mucosal resection or endoscopic submucosal dissection, whereby the esophageal mucosa is actually resected piecemeal. An in-depth discussion of endoscopic resection techniques is beyond the scope of this chapter.
PDT is arguably the most established of these ablative techniques. In a large single-institution study, PDT was combined with the neodymium:yttrium-aluminum-garnet (Nd:YAG) laser to treat 73 patients with high-grade dysplasia and 14 with low-grade dysplasia.25 All patients were observed endoscopically for 12 months. Regression to low-grade dysplasia or no dysplasia was observed in 88% of high-grade dysplasia cases and 93% of low-grade dysplasia cases. Complete eradication of Barrett’s epithelium occurred in only 9% of patients after PDT alone. In focusing on the PDT-treated area, a 49% eradication rate was reported. The addition of laser ablation to residual areas of Barrett’s epithelium increased the eradication rate to 87%. This group used a transparent centering balloon for PDT delivery, which is no longer available commercially. In a prospective randomized multicenter international trial, omeprazole (20 mg twice daily) was compared with omeprazole plus PDT for the treatment of Barrett’s esophagus with high-grade dysplasia.26 The trial was initially planned for 2 years. However, at the end of 2 years, 65% of the omeprazole-alone group and 59% of the PDT group were enrolled in a long-term follow-up study. At 5 years, the addition of PDT with porfimer sodium to proton pump inhibitor therapy resulted in a 38% increase in Barrett’s high-grade dysplasia eradication rate, a 14% decrease in the incidence of invasive carcinoma, and a longer time to progression to cancer. PDT with a centering balloon was the only endoscopic modality used to ablate Barrett’s mucosa.
PDT can also be used to treat early-stage esophageal tumors (T1-T2) in patients who refuse or are not candidates for surgical resection. In a group of patients that included mostly T1 tumors (12 of 13), regression to Barrett’s esophagus with low-grade dysplasia or without dysplasia was observed in 77% of patients (10 of 13).25 At our center, PDT with curative intent is reserved for patients with high operative risk. All others undergo minimally invasive esophagectomy.18 We have reviewed our institutional experience, from 1997 to 2005, using PDT for patients with high-grade dysplasia or superficial esophageal cancer.27 A total of 50 patients, who either refused or were unfit for surgery, had PDT with curative intent. The majority of patients (70%) had high-grade dysplasia or small (≤T1) tumors. At a median follow-up of 28 months, 32% of patients were alive without recurrence, 30% were alive with recurrent disease, and 38% were deceased from cancer or other causes. The patients with recurrences were re-treated with PDT. There was no procedure-related mortality, and the stricture rate was 42%. Although these results are clearly inferior to esophageal resection, endoscopic ablation appears to be a suitable treatment option for nonsurgical candidates. In another cohort of 28 patients with high-grade dysplasia treated with minimally invasive esophagectomy, 96% remained free of recurrence at 13 months.18 These results were corroborated by other investigators who have observed excellent long-term survival (100%) after esophagectomy for high-grade dysplasia.28
In the treatment of Barrett’s esophagus with high-grade dysplasia, PDT is well tolerated with usually transient side effects, such as nausea, regurgitation, constipation, odynophagia, dysphagia, weight loss (average of 6 kg), and noncardiac chest pain.29,30 Other complications include stricture, tumor growth below the PDT-induced scarring, and Barrett’s esophagus and tumor recurrence.31 In our experience, approximately 30% of patients with high-grade dysplasia undergoing PDT will develop an esophageal stricture.27 Stricturing is minimized by decreasing the intensity of light therapy compared with doses used for larger tumors.
Another emerging endoscopic treatment modality for patients with Barrett’s esophagus is radiofrequency (RF) ablation. The components of the system include a sizing balloon (18 to 34 mm), a 3-cm balloon-based bipolar RF electrode, and a dedicated RF generator. The sizing balloon is used to ensure proper contact between the balloon electrode and the esophageal mucosa. The RF mucosal ablation is achieved by using a power of 300 W to deliver 10 to 12 joules/cm2. The coagulum is then removed from the esophageal surface by irrigation and suction. The maximum ablation depth is 1 mm, which translates to a depth of injury that is superficial to the muscularis mucosa.32 The depth of injury is highly reproducible and has been confirmed in the human esophagus. In addition to the balloon electrode, there is an RF ablation device that can be attached to the end of a flexible esophagoscope and used to ablate focal areas of esophageal mucosa. Encouraging results have been published showing complete eradication of Barrett’s mucosa and intramucosal carcinoma when RF ablation was used in combination with endoscopic mucosal resection.33 However, studies involving larger cohorts of patients with longer follow-up time have not yet been published.
Palliative Intent
Stenting of the esophagus is probably the most commonly used modality to palliate dysphagia.34 However, PDT offers some advantages in certain clinical situations. In addition to relieving obstruction, PDT can control or prevent bleeding from the tumor. It may also prevent the globus sensation associated with stents in the proximal esophagus and the reflux symptoms observed with stents bridging the gastroesophageal junction. PDT is a potential alternative to stenting for endoluminal cancers in both of these locations.
PDT has been compared with laser ablation in a randomized trial. PDT was equivalent to laser ablation for malignant dysphagia and was associated with a lower rate of perforation (PDT, 1%; laser, 7%).35 We reviewed our experience with PDT in 215 patients suffering from obstructing esophageal cancer.36 The most common pathologic process was adenocarcinoma (83%). The distal esophagus was involved in 71% of cases. Dysphagia improved in 85% of patients, and bleeding was successfully controlled in 90%. Stenting was subsequently required in 24% of patients. As expected, median survival was poor at 4.9 months; however, effective palliation was provided to these unfortunate patients. In another prospective series of 77 patients for whom conventional therapy had failed or who were deemed unfit for surgery, investigators reported a median survival of 6.3 months after PDT.14 The only significant predictor of mortality was clinical stage. Photosensitivity appears to be an acceptable tradeoff in these patients. In one study using satisfaction questionnaires, patients reported that the ability to swallow food was more important than the limitations of photosensitivity.29 In most cases, the potential benefits of PDT far outweigh the risk of complications.
In patients with obstructing aerodigestive tumors, PDT may result in adverse events. Esophageal strictures (4.8% to 5.2%), perforation (0% to 9.1%), and esophagorespiratory fistulas (0% to 5.2%) are among the most serious PDT-related complications.7,14,37–39 Esophageal perforation and fistula are probably best treated by esophageal stenting. Other factors contributing to the mortality rate and risk of esophageal perforation include the generally poor performance status of this particular group of patients and the fact that some patients with obstructing tumors receive concurrent radiation therapy and chemotherapy.
STENTS
Expandable Metal Stents
Expandable metal stents are constructed with cobalt alloys (Wallstent, Schneider), stainless steel (Gianturco, Cook), or a nickel-titanium alloy referred to as nitinol (EsophaCoil, Medtronics; Ultraflex, Microvasive). These materials are resistant to corrosion and are biologically inert, even in patients allergic to nickel. The wire stents can be woven (Wallstent), knitted (Ultraflex), or bent into a zigzag (Gianturco) or coil (EsophaCoil) configuration. The stent design influences its retraction properties (i.e., shortening). Retraction percentage is highest with the coil configuration and lowest with the zigzag configuration. The shape memory characteristics of the materials allow the stent to reexpand to its original tubular shape even when it is compressed into a delivery system. The available systems can deploy the stent from the proximal end, the center, or the distal end. Proximal delivery is better suited for proximal strictures, and distal delivery is best for strictures of the gastroesophageal junction. Expandable metal stents can also be partially covered with polyurethane or silicone (e.g., Alimaxx-E, Alveolus; Polyflex, Microvasive; Ultraflex). Covered designs help reduce tumor ingrowth but at the same time have a propensity for migration.40 The wide range of available diameters and lengths allows expandable metal stents to fit most upper aerodigestive strictures.
Silicone-Based Stents
Silicone-based stents are made of silicone rubber (Silastic, Dow Corning), a ubiquitous component of industrial, household, and medical equipment. Celestin esophageal tubes have fallen out of favor because of the need for an open gastrotomy for anchoring and the higher complication rate in comparison with expandable metal stents.41
Technique
Silicone-Based Stents
Unless the patient has a tracheal stoma, rigid bronchoscopy is needed to insert a silicone-based stent. A chest tube and the stent are passed over a rigid scope in sequence. The chest tube serves as a pusher to deliver the stent over the rigid scope. For Y-shaped stents, the right main-stem bronchus limb is invaginated and the rigid scope passed through the left main-stem bronchus limb. After it is positioned, the scope is pulled back within the tracheal limb, and biopsy forceps are used to push out the right-sided limb. An alternative technique is to guide the right-sided limb into the right main-stem bronchus by use of a Fogarty catheter while positioning the proximal portion of the stent endoscopically. A complete technical description of rigid endoscopic stent insertion has already been published.42 Table 6-1 compares some of the features of silicone and metal stents.
Indications
The recommended definitive treatment of benign airway strictures is surgical resection. Stenting is indicated for strictured or malacic airways not amenable to resection or in nonsurgical candidates in whom dilation or laser resection has failed. Stenting may also be used as a bridge to surgery in patients needing time for their general condition to improve. It should be clear that stenting of benign strictures is suboptimal therapy, as 40% of patients will need re-intervention.43
Benign Airway Strictures
Stenting of benign airway strictures should be regarded as second-line therapy, reserved for situations in which surgical resection is not possible. In comparison with the shorter survival expected with malignant strictures, patients with benign strictures live longer and require further intervention over time. For this reason, silicone stents are recommended. Previous experience with silicone stents for benign strictures in nonoperative candidates has been relatively good.44,45 Of 112 patients with T stents, only 5 (4.5%) required removal for obstructive problems, and 85% were managed successfully for periods of 3 months to more than 5 years.45 In another case series, 94% of patients with benign strictures had successful palliation of obstruction.43 Only 3 of 54 silicone stents (5.6%) needed removal or replacement for migration or compression, and the mortality rate was 12.5% at 4 years of follow-up. Other studies involving smaller numbers of patients (6 to 22) reported symptomatic and spirometric improvement after stenting.46–48 Only one group acknowledged that granulation tissue is a common finding (up to 80% of patients), which may lead to removal in 20% of cases.48
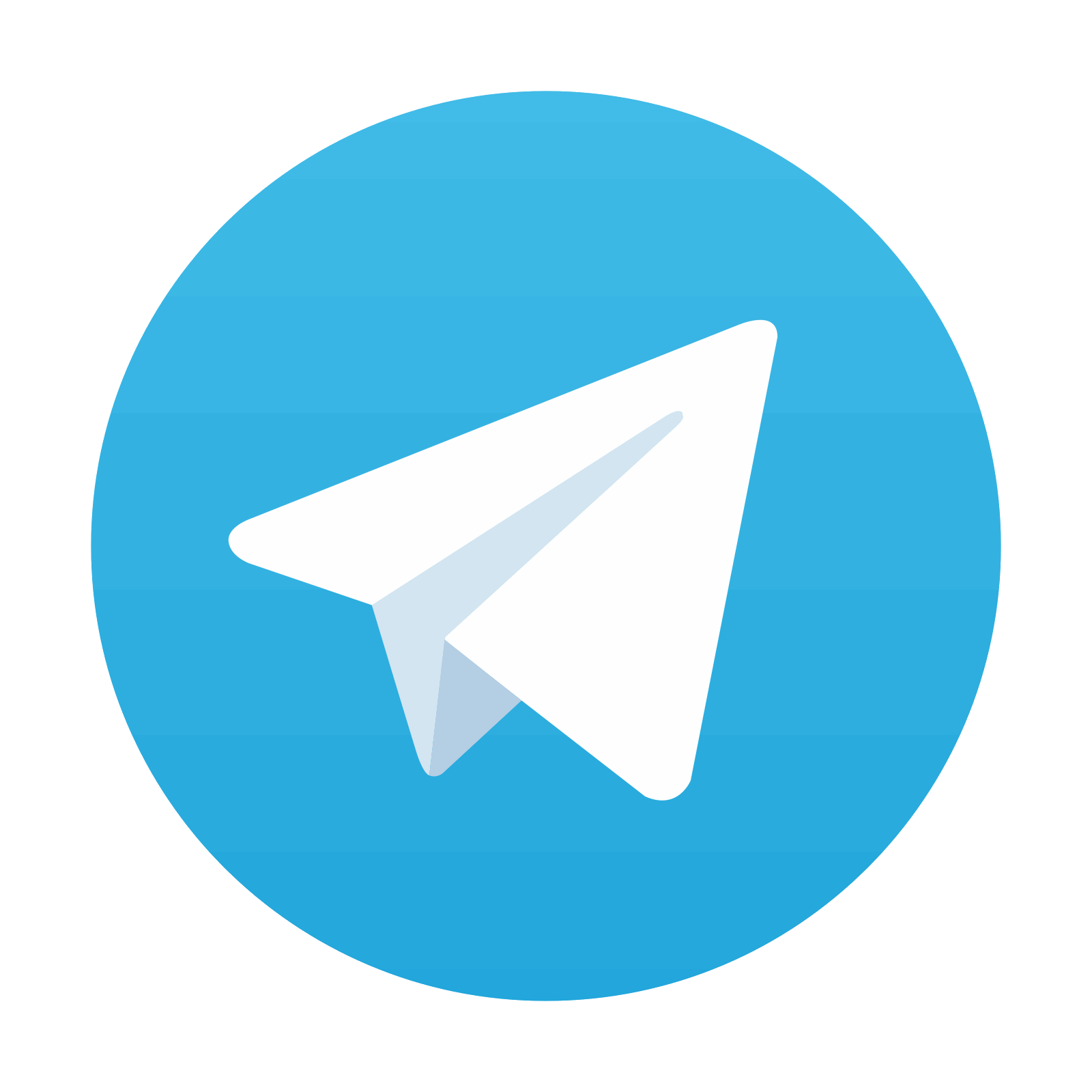
Stay updated, free articles. Join our Telegram channel

Full access? Get Clinical Tree
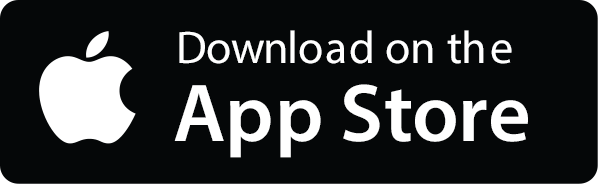
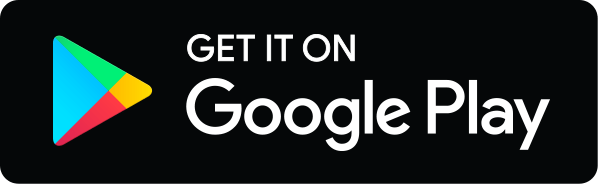