The nonosmotic release of arginine vasopressin, concurrent with the activation of the sympathetic nervous system and renin-angiotensin-aldosterone system, is thought to represent the maladaptive response that is central to the pathophysiology of heart failure (HF). The degree of neurohormonal activation correlates with the severity of the disease and can predict the outcomes. However, quantification of components of neurohormonal axis (e.g., serum arginine vasopressin level) is mainly reserved for research purposes rather than routine practice. The results of several recent HF trials have shed light on the differential role of blood urea nitrogen (BUN) and creatinine in predicting the outcomes in this setting. These studies suggest that BUN could indeed represent a surrogate marker for “renal response” to neurohormonal activation in this setting, above and beyond its role in the estimation of renal function. In this report, the relevant physiologic mechanisms underlying urea and water transport in the kidney are first reviewed. Then, the activation of the neurohormonal axis and the impact of its components on renal urea transport, independent of changes in renal function, are explained. Finally, the unique role of BUN as a biomarker of neurohormonal activation in the setting of HF is discussed, and the potential clinical implication of this novel concept is emphasized. In conclusion, this review explains the pathophysiologic basis for the emerging role of BUN as a biomarker in HF.
Over the past decade, our understanding of the pathophysiologic mechanisms underlying heart failure (HF) has dramatically increased. The neurohormonal axis has been shown to play a major role in the development and progression of the disease. Moreover, attempts have been made over the past few years to develop risk stratification tools to help quantitatively estimate prognosis in these patients. Several of these clinical risk stratification tools that are generated through analysis of population-based studies on patients admitted for decompensated HF have consistently pointed to blood urea nitrogen (BUN) as 1 of the strongest prognostic factors. The explanations and significance of this finding as well as its potential implications are the focus of this review.
Physiologic Considerations
Renal urea transport
Waste nitrogen is excreted by the kidney primarily as urea (90%), a small molecule that is highly soluble in water. Mechanisms involved in renal urea reabsorption play a crucial role in the urine-concentrating ability of the kidney. It has been shown that the rate of urea excretion is determined mostly by its rate of glomerular filtration and tubular reabsorption. Urea reabsorption is through 2 mechanisms; one is in the proximal tubules, where about 40% of the filtered urea is constitutively reabsorbed. Because the reabsorption of urea is “concentration dependent” in this segment, any process that increases water reabsorption can increase the concentration of the urea in this segment, hence increasing its passive reabsorption down a concentration gradient. The second mechanism takes place in the distal nephron (the inner medullary collecting duct) and is dependent on the level of antidiuresis; higher levels of arginine vasopressin (AVP) induce extensive absorption of urea in this segment. The terminal part of the inner medullary collecting duct possesses a high level of urea permeability that is dramatically increased in the presence of AVP, a process that has been found to be transporter mediated. So far, 2 urea transporter genes have been cloned: UT-A and UT-B. The urea transporters have a widespread tissue distribution (e.g., the heart), although their exact role in extrarenal tissues is not yet clear. In the kidney, the abundance of urea transporters is regulated by AVP, glucocorticoids, and mineralocorticoids. It is noteworthy that the urea reabsorption process in the distal nephron is known to be “flow dependent”; a decrease in distal fluid delivery can lead to slow tubular flow in the collecting duct, with a resultant increase in urea reabsorption. Interestingly, AVP-regulated water transport in the kidney also takes place in the distal nephron but through distinct transport mechanisms and channels.
Water transport
Aquaporin water channels provide a pathway for water movement across the lipid bilayers of the cell membranes. Aquaporin-2 is found exclusively in the principal cells of the collecting tubules and duct and is the main channel for water reabsorption across the apical membrane of the principal cells. Through V 2 receptors located on the basolateral membrane of the collecting duct, AVP increases the trafficking of vesicles containing aquaporin-2 to the apical surface and their fusion to luminal membrane, resulting in an increase in water permeability. As expected, higher levels of AVP are associated with greater abundance of aquaporins in the distal nephron and induce free water reabsorption; its suppression, in contrast, leads to reversal in the exocytosis of aquaporin-2 channels and significant increase in renal solute-free water clearance. Once AVP’s effect has worn off, the aquaporins are removed from the membrane by endocytosis and are returned to the cytoplasm.
AVP
AVP is a polypeptide synthesized in the neurosecretory cells of the paraventricular and supraoptic nuclei of the hypothalamus and is excreted by the posterior pituitary gland. Plasma hyperosmolality, perceived by osmoreceptors, is the major stimulus to AVP secretion. The osmotic threshold for AVP release is about 280 to 290 mosm/kg. The system is so efficient that despite significant variation in water intake, in normal circumstances, plasma osmolality remains quite stable within a 1% to 2% change range. Other (i.e., nonosmotic) stimuli for AVP release have also been identified, such as reductions in circulating blood volume and angiotensin II. Volume-sensitive AVP release is mainly mediated by baroreceptors located in the carotid sinus. In contrast to osmoreceptors, the volume receptors are not very sensitive; they respond only to significant changes in effective blood volume that are large enough to produce systemic hypotension. The actions of AVP are mediated through vasopressin receptors (i.e., V 1a , V 1b , and V 2 ). The V 2 receptors are primarily located on the basolateral surface of the cells in renal collecting ducts and regulate water transport through expression of aquaporin-2. In the presence of plasma hypo-osmolality (e.g., hyponatremia), serum AVP concentrations are normally undetectable and the urine is maximally diluted. However interactions between osmolal and volume receptors could portend clinically relevant consequences that are distinct from their isolated effects. For instance, concomitant presence of hypo-osmolality and low effective circulatory volume (e.g., in case of coexisting hyponatremia and HF) is associated with persistent AVP release, although low plasma osmolality under normal circumstances should inhibit the secretion of AVP. AVP is rapidly metabolized, with a circulatory half-life of <20 minutes.
The renin-angiotensin-aldosterone system (RAAS)
The RAAS has been recognized as one of the most complex regulatory systems in the body, with various hemodynamic, cardiovascular, and renal effects in health and disease. The renal cortical juxtaglomerular apparatus remains the main known source of renin release. However, the local generation of renin-angiotensin (e.g., in the brain and heart) has also been the focus of many recent studies, and there is evidence that the plasma renin activity alone might not correlate with angiotensin II activity. Angiotensin II interacts with ≥2 classes of angiotensin receptors: AT-1 and AT-2. Beside its indirect effect on sodium reabsorption via aldosterone release, angiotensin II has a direct antinatriuretic action throughout the nephron. Activation of AT-1 receptor increases the activity of the proximal tubular apical sodium-hydrogen exchanger as well as the basolateral sodium-bicarbonate cotransporter. In the distal tubules, it enhances the sodium-chloride cotransporter while activating the sodium epithelial channels in the collecting duct.
The sympathetic nervous system (SNS)
The SNS is an important regulatory mechanism for the conservation of sodium and water in physiologic and pathologic circumstances. Renal sympathetic nerve terminals are in direct contact with the peritubular basement membrane throughout the nephron. The juxtaglomerular granular cells are also innervated by sympathetic nerve fibers. Any alteration in renal sympathetic nerve discharge could directly affect renal tubular transport function as well as the release of renin and activation of the RAAS. Beside the tubular effects, sympathetic nerve fibers densely innervate afferent and efferent arterioles in the kidneys and can affect renal hemodynamics through differential vasoconstriction of these arterioles. Increased SNS activity in the kidney in various clinical settings such as decreased right atrial pressure leads to direct enhancement of tubular sodium reabsorption. It has been shown that increased renal sympathetic activity is associated with decreased renal excretion of the sodium, although the glomerular filtration rate (GFR) or renal blood flow could remain unchanged. Therefore, the increased sodium reabsorption is due to direct action of the sympathetic nerve terminals rather than a decrease in filtration of sodium due to reduced renal blood flow.
Arginine Vasopressin in Heart Failure
Consistent with the aforementioned mechanisms, left ventricular dysfunction and associated low effective circulatory volume lead to increased AVP release that is not mediated or modified by plasma osmolality. In high-output HF, the associated systemic arterial vasodilation can similarly activate the baroreceptors and initiate the same process. Several clinical trials have consistently demonstrated that AVP levels are inappropriately elevated in patients with HF. Moreover, data from Studies of Left Ventricular Dysfunction (SOLVD) indicate that there is a progressive incremental increase in serum AVP levels as congestive symptoms worsen in chronic HF. Although the impact of high serum levels of AVP on the development of progressive congestion, hyponatremia, and vasoconstriction is established, the significance of its effect on urea transport in HF is less well known. Excessive upregulation of urea transporters in the collecting duct by chronically elevated levels of AVP is in part responsible for the unexpected high levels of BUN that are not coupled with the degree of renal dysfunction in these patients. Because serum levels of AVP are correlated with the severity of HF, it is then conceivable that blood urea concentrations portend clinical relevance in this setting (see the following discussion). Other cardiovascular aspects of elevated AVP levels (e.g., role in cardiac remodeling or interference with normal coronary vasodilatory response) are beyond the scope of this review.
Arginine Vasopressin in Heart Failure
Consistent with the aforementioned mechanisms, left ventricular dysfunction and associated low effective circulatory volume lead to increased AVP release that is not mediated or modified by plasma osmolality. In high-output HF, the associated systemic arterial vasodilation can similarly activate the baroreceptors and initiate the same process. Several clinical trials have consistently demonstrated that AVP levels are inappropriately elevated in patients with HF. Moreover, data from Studies of Left Ventricular Dysfunction (SOLVD) indicate that there is a progressive incremental increase in serum AVP levels as congestive symptoms worsen in chronic HF. Although the impact of high serum levels of AVP on the development of progressive congestion, hyponatremia, and vasoconstriction is established, the significance of its effect on urea transport in HF is less well known. Excessive upregulation of urea transporters in the collecting duct by chronically elevated levels of AVP is in part responsible for the unexpected high levels of BUN that are not coupled with the degree of renal dysfunction in these patients. Because serum levels of AVP are correlated with the severity of HF, it is then conceivable that blood urea concentrations portend clinical relevance in this setting (see the following discussion). Other cardiovascular aspects of elevated AVP levels (e.g., role in cardiac remodeling or interference with normal coronary vasodilatory response) are beyond the scope of this review.
The Renin-Angiotensin-Aldosterone System in Heart Failure
The activity of the RAAS is increased in most patients with HF, although even normal levels of plasma renin activity and angiotensin are considered inappropriate in the setting of extracellular volume expansion. It has been shown that increased plasma renin activity is associated with deterioration in left ventricular function and adverse outcomes. In addition to its direct effects on the heart (e.g., remodeling of the left ventricle), increased activity of the RAAS can lead to enhancement of renal tubular reabsorption of urea via 2 distinct mechanisms. First, angiotensin II enhances absorption of the sodium and water in the proximal tubules and leads to an increase in the concentration of the filtered urea in the proximal tubules. This in turn causes significant passive reabsorption of the urea through a concentration gradient. Second, increased reabsorption of the water in the proximal nephron reduces the distal fluid delivery, resulting in slow tubular flow in the collecting duct, hence further increasing the flow-dependent reabsorption of the urea in this segment.
The Systemic Nervous System in Heart Failure
A number of complex mechanisms, including suppression of sympathoinhibitory cardiovascular reflexes (e.g., arterial baroreceptors), augmentation of sympathoexcitatory reflexes, and remodeling of cardiac neuronal hierarchy, result in SNS hyperactivity in patients with HF; they have significantly higher levels of circulating norepinephrine, which correlates directly with the severity of the cardiac dysfunction and mortality. In an analysis of >4,000 patients with from the Valsartan Heart Failure Trial (Val-HeFT), Anand et al found that patients with stable symptomatic HF with initial plasma norepinephrine concentrations in the highest quartile had a significantly higher mortality rate at 2 years compared to those with initial norepinephrine concentrations in the lowest quartile (24.2% vs 13.8%, p <0.0001). Moreover, effective treatment of HF and improvement in cardiac function reduce the degree of sympathetic activation. In a study by Sigurdsson et al, they demonstrated that 3 months of therapy with ramipril could significantly lower norepinephrine levels in patients with mild or moderate HF and the most pronounced degree of neurohormonal activation. Independent of its adverse impact on GFR and renal blood flow, SNS activation in HF can increase BUN with mechanisms that are similar to RAAS activation and mainly involve sodium and water transport: increase in passive urea reabsorption due to increased sodium and water reabsorption in the proximal tubule. The enhanced urea reabsorption also takes place in the distal tubules, mainly because of reduced delivery of sodium and water to this segment.
Kidney Function in Heart Failure
Abnormal kidney function is a strong and independent risk factor for adverse outcomes in a broad range of HF populations. Identification of the optimal method for evaluating renal function is challenging in these patients. Serum creatinine level has been shown not to be an accurate indicator of renal function in this setting. The Modification of Diet in Renal Disease (MDRD) study equation has been recommended by some investigators for prognostic purposes in patients with HF. However, the MDRD equation has also a number of limitations that make it less attractive in this setting. Advanced age, malnutrition, and low muscle mass can reduce creatinine generation, hence creating a potential bias in the interpretation of creatinine-based formulas such as the MDRD equation. In a recent study of 426 patients with systolic HF and normal serum creatinine levels, Scrutinio et al sought to identify the best estimator of renal dysfunction with regard to prognostic accuracy. They reported that estimated GFR on the basis of the Cockroft-Gault formula normalized for body surface area was the best estimator of renal function for the prediction of mortality. Of note, nearly 1/4 of the patients with chronic HF in that study had prognostically significant renal dysfunction, although their serum creatinine levels were within the normal range. Similar to serum creatinine level, exclusive use of BUN is in general not considered a reliable method of estimating renal function, because it is affected by protein intake, catabolism, and rate of renal urea reabsorption. However, BUN might have other clinical implications in HF populations that are prognostically meaningful (see the following discussion).
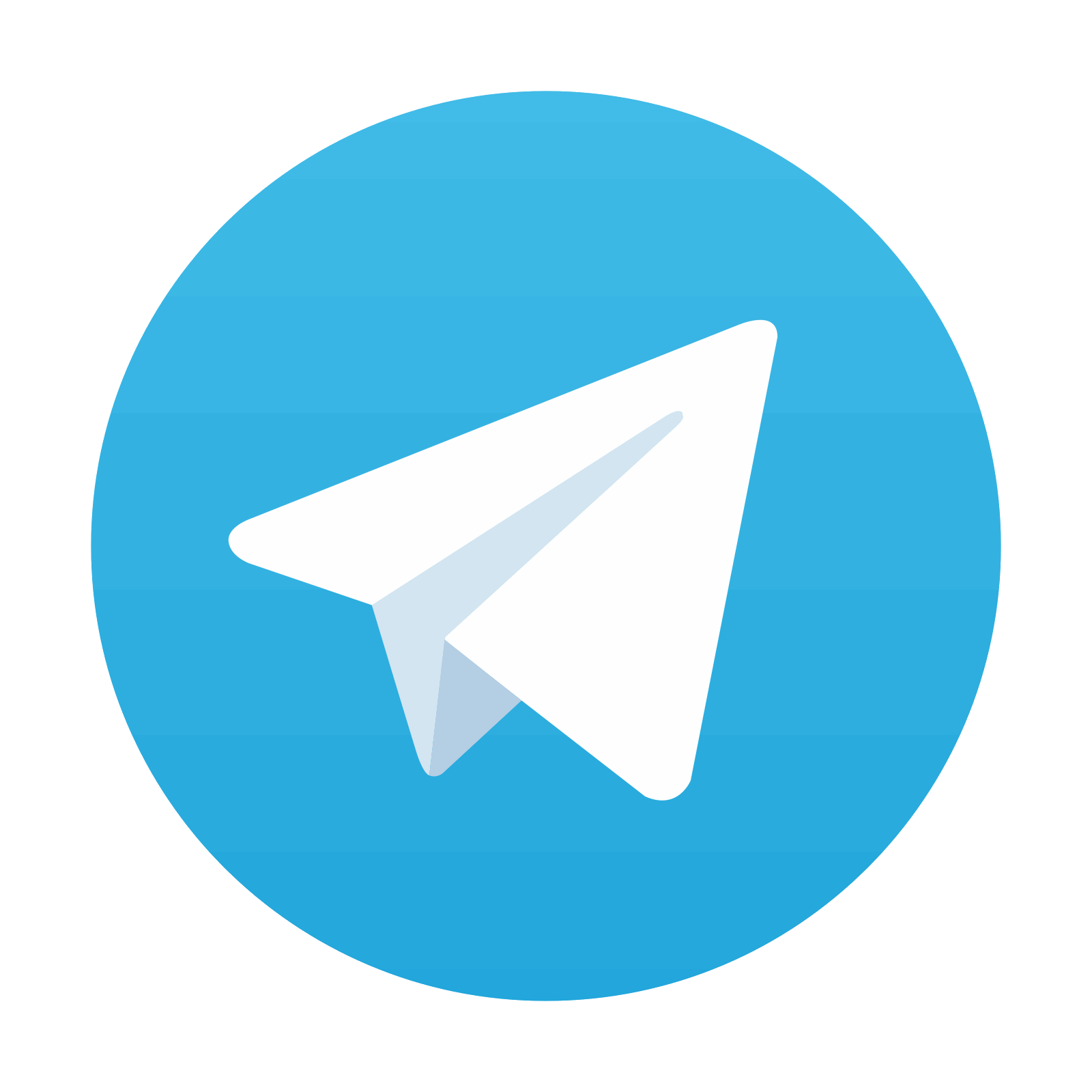
Stay updated, free articles. Join our Telegram channel

Full access? Get Clinical Tree
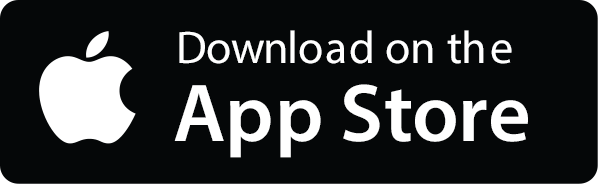
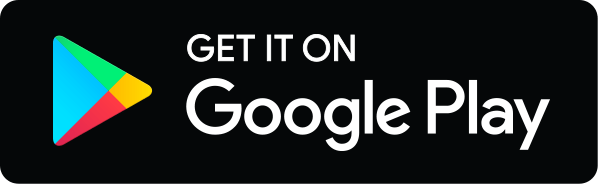