Electrophysiologic Testing
Andrew Krumerman
John D. Fisher
Overview
An electrophysiologic study (EPS) provides a safe and effective method for evaluation, risk stratification, and treatment of known and suspected arrhythmias. This chapter emphasizes EPS indications, techniques and normal findings.
An EPS permits assessment of individual portions of the cardiac electrical system. These assessments include evaluation of spontaneous function, responses to stresses, and vulnerability to induced tachyarrhythmias. Indications and guidelines for EPS have been established for a broad range of situations and clinical problems (1) (Table 61.1).
Glossary
AH interval
The interval from the first rapid deflection of the atrial electrogram on the His bundle lead to the first inscription of the His bundle depolarization.
Cycle length (CL)
Interval in milliseconds. During EPS, cycle lengths or intervals often change from beat to beat, so that these measures are more relevant than an overall rate, which is expressed in beats per minute. The use of rates in beats per minute is retained mostly to facilitate communications with physicians who are more comfortable with this terminology.
Effective and functional refractory periods
Measurements of impulse propagation at various levels in the heart (see text for detailed definitions).
Effective and functional conduction periods
Measurements of impulse propagation at various levels in the heart (see text for detailed definitions).
HV interval
The interval from the first inscription of the His bundle potential to the first evidence of ventricular depolarization on the surface ECG or the His bundle lead.
PA interval
The interval from the first evidence of sinus node depolarization, whether on the intracardiac or surface ECG, to the atrial deflection as recorded in the His bundle lead.
S1, S2,…,SN
S1 refers to the baseline or drive stimulus in a series, with each of the series of S1s being of equal intervals. S2 is the first extrastimulus, with the S1S2 interval almost always shorter than the S1S1 interval. The S3, S4, and SN stimuli are the second, third, and Nth extrastimuli, respectively. See text for details.
Historical Perspective
The leap from the experimental animal laboratory to the clinical arena and clinical cardiac electrophysiology was made possible by the development of cardiac catheterization and transvenous pacing techniques. Intracardiac recordings from
the right atrium, His bundle region, and right ventricle allowed direct rather than inferential analysis of normal and abnormal rhythms (2,3). Electrophysiologic testing was quickly established as the definitive method for diagnosis and subsequently for treatment of cardiac rhythm disorders.
the right atrium, His bundle region, and right ventricle allowed direct rather than inferential analysis of normal and abnormal rhythms (2,3). Electrophysiologic testing was quickly established as the definitive method for diagnosis and subsequently for treatment of cardiac rhythm disorders.
TABLE 61.1 Major Indications for Electrophysiologic Testing | |||||||||||||||||||||||||||||||||||||||||||||||||||||||||||||||
---|---|---|---|---|---|---|---|---|---|---|---|---|---|---|---|---|---|---|---|---|---|---|---|---|---|---|---|---|---|---|---|---|---|---|---|---|---|---|---|---|---|---|---|---|---|---|---|---|---|---|---|---|---|---|---|---|---|---|---|---|---|---|---|
|
Introduction
A complete EPS consists of a series of tests that are designed to evaluate the heart from top to bottom, that is, from the sinus node to the ventricle with steps in between. Depending on the purpose of the EPS for a given patient, all or only some of these regions may be tested, in an à la carte fashion.
The testing techniques used to evaluate each region are remarkably similar. Pacing at increasing rates and programmed electrical stimulation (PES) form the core of testing at each level, supplemented by various pharmacologic probes and physical maneuvers.
This chapter emphasizes the indications for EPS, matters related to patient preparation, vascular access, stimulation and recording techniques, and an outline of EPS related to the major parts of the heart’s electrical system. Other chapters cover the details of specific arrhythmias.
Indications for Electrophysiologic Study
The earliest practical application of EPS was for assessment of the conduction system. This was followed in rapid succession by EPS for characterization of various tachycardias; as a guide for antiarrhythmic drug efficacy; identification of the cause of syncope of undetermined origin (SUO); arrhythmia mapping before and during open heart surgery; and as an integral part of the procedure for catheter ablation of tachycardias. Guidelines on the indications for EPS have been published by the American College of Cardiology (ACC) together with the American Heart Association (AHA) and by the Heart Rhythm
Society (HRS), but they have not been recently updated (1) (Table 61.1).
Society (HRS), but they have not been recently updated (1) (Table 61.1).
Patient Preparation
Education and Consent
Patients are often quite anxious about the prospect of an invasive study. Most diagnostic EP studies involve only venous access, and they are therefore safer than coronary angiography. The latter is a procedure that many patients are familiar with and tend to take for granted. Electrophysiologists often have patients referred to them who have been told by the referring physician that EPS is a minor, low-risk procedure. These patients may be alarmed to learn that no procedure is risk free and that EPS may require several hours. It is therefore important for realistic patient education to begin with the referring physician. The electrophysiologist should put the various risks in context. Patients can understand analogies, such as the risks versus the benefits of walking across a busy street. Patients should also have a realistic idea of the benefit they may derive from undergoing EPS, including the possibility that the study may be negative or equivocal.
Preprocedure Preparations
Traditionally, diagnostic EPS was performed in the “postabsorptive, nonsedated state.” There are of course some problems with this idea. There is great variation among patients in the anxiety that they experience during invasive procedures. As EP procedures became longer and more complex, it became clear that sedation would be required for many of these individuals.
Twelve-Lead Electrocardiogram
It is helpful to have a 12-lead ECG available, particularly when tachyarrhythmias are expected during the procedure or for patients for whom ischemia is an issue. It is desirable for the 12 leads to be recorded simultaneously or in just a few seconds in case the arrhythmia is fast, slow, or transient.
Defibrillator Pads
Using preapplied adhesive defibrillator pads avoids the need to disrupt the sterile field in the event that electrical defibrillation/cardioversion is needed during the procedure.
Arterial Lines
Arterial lines are used routinely in a minority of EP labs, except in unstable patients or for certain ablation procedures. Automated/cuff blood pressure devices are usually adequate.
Sedation
Many patients benefit from minimal sedation. Longer procedures and ablations are now routinely performed using intravenous “conscious” sedation. Guidelines are published by the HRS (4).
Urinary Problems
Urinary retention may occur in individuals of either gender during lengthy EP studies, particularly if the study is combined with sedation and a tachycardia-related diuresis. When this situation is anticipated, it is useful to insert a Foley catheter prior to the procedure. Lubrication and topical anesthesia of the urethra using a commercially available lidocaine jelly injector system makes catheterization both acceptable and well tolerated by the patient.
Oxygen and Carbon Dioxide Monitoring
Monitoring of O2 saturation has become virtually routine in many laboratories and is of particular value in sedated patients. If patients are receiving supplemental oxygen, the O2 saturation may be misleadingly high. Expired CO2 monitors are useful in preventing hypercapnia in such situations and are recommended in the guidelines for conscious sedation.
Universality of Stimulation Techniques
There is a relatively small menu of stimulation techniques useful in the study of both bradycardias and tachycardias.
Stimulus Amplitude and Pulse Duration (Pulse Width)
Most EP labs use outputs at two to four times the diastolic threshold. Twice the threshold is more common. In general, refractory periods (see later discussion) are somewhat longer when determined using twice the threshold, and this may reduce the incidence of induction of “nonclinical” tachyarrhythmias. At four times the threshold, one is at the beginning of the more vertical portion of a strength–interval curve, which may have several advantages (5,6).
A pulse duration of 1 or 2 msec is most commonly used. This dates from the early days of clinical EP when implanted pacemakers had similar pulse durations, as do current temporary pacemakers. This pulse duration is now out of synchrony with those used in implantable devices, but it has been retained for the sake of consistency in EP protocols done over long periods of time.
Most commercial EP stimulators emit constant-current pulses. Initially this too was in keeping with the norm for implantable pacemakers. The latter are now nearly universally constant-voltage devices. Testing of patients with and without clinical tachycardias with both constant-voltage and constant-current stimulation, all at four times the diastolic threshold, produced no significant difference in any of the results.
Incremental versus Decremental
Both terms are in regular use and have opposite meaning depending on whether one is considering rate in beats per minute or cycle length (CL) in milliseconds. The paragraphs that follow employ the most conventional usage.
Straight Pacing
The pacing rate or cycle length is maintained throughout the duration of the stimulation. There are several common applications for straight pacing.
(Rate) Incremental Pacing
After pacing at a given rate for a predetermined number of stimuli or seconds, the rate is then increased (with or without intervening pauses) in a series of steps until predetermined endpoints are reached. The terminology of rate incremental pacing is derived from the use of stimulators controlled by an analogue dial. Digitally controlled devices often increase the rate by choosing a sequence of cycle length decrements, but the term “incremental pacing” may still be used.
S1S1 Drive Stimuli
The heart is paced, or driven, at a specified rate and duration (typically 8 beats), after which a premature extrastimulus is delivered (see later discussion). The eight drive beats are each termed S1 stimuli. These S1s may be followed by first, second, third, and Nth premature extrastimuli, which are designated S2, S3, S4, and SN, respectively. When extrastimuli follow a series of sinus beats, the latter may also be designated
as S1s. S1S1 drive stimuli are sometimes called “trains,” but this can be confused with ultrarapid train stimulation (see later discussion), which is also referred to as “trains.”
as S1s. S1S1 drive stimuli are sometimes called “trains,” but this can be confused with ultrarapid train stimulation (see later discussion), which is also referred to as “trains.”
Burst Pacing for Tachycardia Induction
Stimuli are delivered at a constant rate for a relatively short duration but at successively faster rates with each burst until a predetermined maximum rate (or minimum interval, i.e., CL) has been reached.
Bursts for Termination of Tachycardia
This procedure is similar to the technique used for induction. The initial pacing rate is faster than that of the tachycardia.
Underdrive Pacing for Tachycardia Termination
This refers to pacing at a rate slower than the tachycardia at a constant rate. Stimuli will therefore fall at differing points in the tachycardia cycle.
Ramps
Ramp pacing implies a smooth change in the interval between successive stimuli. Ramps too can be incremental or decremental as defined by either rate or cycle length. Ramps have several applications:
As a test for conduction, long ramps with small cycle-length decrements between successive stimuli provide results comparable to stepwise rate incremental pacing (7).
For tachycardia induction, the ramp is decreased in duration but the steepness of the slope is increased (i.e., interstimulus intervals are decreased more rapidly). Successive ramps go to higher and higher rates (shorter intervals) up to a predetermined limit.
For tachycardia termination, the procedure is somewhat similar to the previous one. It is commonly used in antitachycardia pacing algorithms in implantable cardioverter-defibrillators (ICDs). Programmed rate incremental ramps are also known as autodecremental pacing.
Extrastimulus Technique
One or more extrastimuli, (designated S2, S3, SN) are introduced at specific coupling intervals based on previous drive S1s, or spontaneous beats. Thereafter the S1S2 interval is altered, usually in 10- to 20-msec steps, until an endpoint is reached, such as tissue refractoriness or termination or induction of a tachycardia. It is usual to begin late in diastole and successively decrement the S1S2 interval. When the physician is satisfied with the results of S1S2 testing, a second extrastimulus (S3) may then be introduced with the S2S3 interval altered in a fashion similar to that used for S1S2.
Two methods are in common clinical use for decrementing the S1S2S3S4 intervals (8,9). In the tandem method the S1S2 is decremented until S2 does not capture, and then the S1S2 interval is increased by 40 to 50 msec and held there. S3 is then introduced, and the S2S3 interval is decremented until S3 fails to capture. At that point, the S1S2 interval is decremented, and S3 is retested to see whether it captures. From that point on, the S1S2 and S2S3 are decremented in tandem until refractory, so that both they and the S3S4 interval are altered in a fashion similar to that used for S2S3. In the simple sequential method, the S1S2 interval is decremented until it no longer captures, and then it is incremented until it does (usually 10 msec). The S1S2 interval is then held constant while the S2S3 interval is decremented in a similar fashion to that used for S1, and then the same for S3S4. The tandem method can be looked on as more conservative because it favors relatively longer intervals and gives a larger number of stimulation runs before moving on to the next extrastimulus. Prospective studies comparing the two methods have shown no differences between the two methods in any of the outcomes assessed (8). The choice of the simple sequential or tandem method therefore remains a matter of the investigator’s choice.
Ultrarapid Train Stimulation
Stimuli are delivered at very short intervals (10 to 50 msec) for a duration sufficient to result in a desired number of captures. The technique has long been used experimentally (with sequentially increasing stimulus amplitudes) for induction of ventricular fibrillation (VF). It is now used clinically for that purpose with ICDs. Shorter trains have also proved useful in termination of tachycardias (10) and in induction of monomorphic tachycardias (11). Of all the techniques described, ultrarapid trains are the least universally employed except for VF induction with ICDs.
Stimulation Protocols
Adequacy is more important than universality. Bodies such as the Heart Rhythm Society have published guidelines on the minimum standards for an acceptable protocol (12). In addition to suggesting reasonable ranges of stimulation sites, drive cycle lengths, numbers of extrastimuli, and amplitudes and pulse durations of the extrastimuli, the guidelines also suggest outcome criteria. For example, the protocol used should be able to induce sustained monomorphic ventricular tachycardia (VT) in at least 90% of patients with coronary artery disease who present with such a rhythm.
Signal Filtering and Interelectrode Distance
The surface ECG is usually filtered at 0.1 to 100 Hz. The bulk of the energy is in the 0.1–20-Hz range. Because of interference from alternating current (AC), muscle twitches, and similar relatively high frequency interference, it is sometimes necessary to record the surface ECG over a lower frequency range or to use notch filters. Timing of events with respect to QRS onset is often important during EPS, but it is cumbersome to display all 12 leads of the regular surface ECG. Orthogonal lead systems (XYZ) are logical but not commonly used. It is more typical to use mutually perpendicular leads (I, AVF, and V1), often supplemented by lead II, which gives an indication as to the presence of abnormal left-axis deviation. Many electrophysiologists have their own favorite lead selections.
Intracardiac leads can be placed strategically at various positions within the heart. It is therefore desirable to record local events in the region of the lead rather than far-field events. This is accomplished by filtering intracardiac electrograms, typically at 30 or 40 to 500 Hz. Note that this eliminates the spectrum with the greatest energy that reaches the surface ECG. The higher-frequency waves carry much less energy and may be difficult to detect more than 1 cm away from the recording lead. Bipolar electrograms are generally used, and smaller interelectrode distances record increasingly local events.
Timing of Local Events
With an “unfiltered” bipolar lead, an approaching wavefront creates a positive deflection that quickly reverses itself as the lead passes directly under the electrode. This rapid reversal constitutes the “intrinsic deflection” of the electrogram and represents the timing of the most local event, that is, at the site of the bipole (13). Electrograms are filtered at 30 or 40 to 500 Hz, have a much more jagged appearance. When recorded simultaneously with an unfiltered electrogram, the midpoint of the intrinsic deflection of the unfiltered electrogram occurs at the same time as a similar deflection on the filtered electrogram,
or at the peak positive or negative deflection of the filtered electrogram. The slew rate or dV/dt of the filtered electrogram is so rapid in normal heart tissue that the difference between the peak and the nadir of the deflection is 5 msec or less. Identification of the local event is therefore easy with either filtered or unfiltered electrograms in normal tissue. However, diseased myocardium may conduct very slowly with fractionated electrograms that make local events harder to identify. The electrophysiologist must then decide whether to use onset rather than local criteria for timing of events. Even in normal tissue, onset deflections are usually less crisp than the local deflection. Tradition plays a large role in the choices of deflections used for timing of events (see the section to follow on baseline intervals). At times there may be a deflection that is of particular interest, such as a His or bundle branch deflection, that is very small relative to the size of surrounding electrograms. This is particularly true when the gain must be markedly increased to produce a measurable deflection. In such instances, a “limiter” or “clipper” option on the amplifier/recorder system can eliminate the very highly amplified surrounding signals to allow concentration on the deflection of interest. However, limiters also eliminate the ability to determine the timing of the intrinsic deflection (local timing) of the signals being limited.
or at the peak positive or negative deflection of the filtered electrogram. The slew rate or dV/dt of the filtered electrogram is so rapid in normal heart tissue that the difference between the peak and the nadir of the deflection is 5 msec or less. Identification of the local event is therefore easy with either filtered or unfiltered electrograms in normal tissue. However, diseased myocardium may conduct very slowly with fractionated electrograms that make local events harder to identify. The electrophysiologist must then decide whether to use onset rather than local criteria for timing of events. Even in normal tissue, onset deflections are usually less crisp than the local deflection. Tradition plays a large role in the choices of deflections used for timing of events (see the section to follow on baseline intervals). At times there may be a deflection that is of particular interest, such as a His or bundle branch deflection, that is very small relative to the size of surrounding electrograms. This is particularly true when the gain must be markedly increased to produce a measurable deflection. In such instances, a “limiter” or “clipper” option on the amplifier/recorder system can eliminate the very highly amplified surrounding signals to allow concentration on the deflection of interest. However, limiters also eliminate the ability to determine the timing of the intrinsic deflection (local timing) of the signals being limited.
Catheterization Techniques
The percutaneous technique is used almost exclusively. The His bundle electrogram (HBE) is most commonly recorded using a lead inserted via a femoral vein and advanced through the right atrium across the tricuspid into the right ventricular inflow area. Some other areas are more easily reached through the superior vena cava. Insertion sites can also include the antecubital, jugular, and subclavian veins.
Choices of Surface and Intracardiac Signals
A classic display would include three or four surface ECGs; a recording from the high right atrium (HRA); the HBE, which includes the low medial right atrium, the His bundle deflection, and the right ventricular inflow deflection; and a recording from the right ventricular apex (RVA). Depending on the type of study and the information sought, stimulation and recording from other sites may be appropriate (Fig. 61.1).
The Electrophysiologic Study
Baseline Intervals
The PR interval of the surface ECG can be further subdivided based on intracardiac electrogram (Fig. 61.1). During sinus rhythm, the earliest atrial activity is identified using the high right atrial electrogram (HRA) from the sinus node region at
the junction of the superior vena cava and the right atrium. The P wave is then timed from the earliest evidence of such atrial activity, whether first seen in the HRA lead or in the surface ECG. Next, conduction through the right atrium to the region of the atrioventricular (AV) node and His bundle is then measured as the PA interval. This is defined as the interval from the P wave as just defined to the first rapid depolarization of the atrium recorded on the HBE lead. AV node conduction is measured by the interval from the first rapid deflection of the A wave in the HBE lead to the first evidence of His bundle depolarization on the HBE lead; this is designated the AH interval. Conduction through the His bundle and bundle branches is then measured as the timing from the first evidence of the His bundle deflection to the first evidence of ventricular deflection on the HBE lead or on the surface ECG, whichever comes first. Normal values for these intervals based on a compilation of sources (14,15,16,17,18,19,20,21,22) are found in Table 61.2. Note that the traditional use of first rapid deflections can be quite subjective compared with first evidence or the local or intrinsic deflection.
the junction of the superior vena cava and the right atrium. The P wave is then timed from the earliest evidence of such atrial activity, whether first seen in the HRA lead or in the surface ECG. Next, conduction through the right atrium to the region of the atrioventricular (AV) node and His bundle is then measured as the PA interval. This is defined as the interval from the P wave as just defined to the first rapid depolarization of the atrium recorded on the HBE lead. AV node conduction is measured by the interval from the first rapid deflection of the A wave in the HBE lead to the first evidence of His bundle depolarization on the HBE lead; this is designated the AH interval. Conduction through the His bundle and bundle branches is then measured as the timing from the first evidence of the His bundle deflection to the first evidence of ventricular deflection on the HBE lead or on the surface ECG, whichever comes first. Normal values for these intervals based on a compilation of sources (14,15,16,17,18,19,20,21,22) are found in Table 61.2. Note that the traditional use of first rapid deflections can be quite subjective compared with first evidence or the local or intrinsic deflection.
TABLE 61.2 Normal Conduction Intervals (±2 Standard Deviations) | ||||||||||||||||||||||||
---|---|---|---|---|---|---|---|---|---|---|---|---|---|---|---|---|---|---|---|---|---|---|---|---|
|
A minority of authors use the HQ interval rather than the HV interval. The HQ interval is the interval from the onset of the His bundle potential to the onset of the QRS and the surface ECG. Logically, one might also use information from the right ventricular apical (RVA) lead or other intracardiac leads (23,24), but these are not part of the conventional measurements. The His bundle potential itself should be crisp and distinct and less than 25 msec in duration. The inter atrial conduction is measured from the HRA to the left atrium (LA). Normal values are given in Table 61.2.
Influences on the Timing of Cardiac Intervals
Sympathetic and parasympathetic tone can influence the heart rate and timing of cardiac intervals (25,26). Changes in neurohumoral tone can result from anxiety, sedation, exercise, sleep, ischemia, metabolic state, pharmacologic agents, and maneuvers such as carotid sinus massage (CSM). Some of these are discussed further in the relevant sections.
Carotid Sinus Massage
In most patients, cardioinhibitory effects on the sinus node are most apparent with right CSM, whereas the AV node is more likely to be affected by left CSM, but this distinction is by no means universal (15,28,29,30,31,32,33). Sinus node inhibition results in sinus bradycardia or sinus arrest. Inhibition of the AV node can result in prolongation of the AH interval or in block at the AH level. If CSM results in sinus arrest, concurrent atrial pacing will determine whether there is block at the AV node level as well.
CSM may be particularly revealing in patients who have the Wenckebach phenomenon at the time of their EPS. In one of those “paradoxical responses” of medical lore, the Wenckebach phenomenon at the HV level will sometimes seem to improve with CSM. This is because the sinus node may be slowed, giving the His bundle and bundle branches (which are much less affected by vagal tone) time to recover and resume conduction. In a patient with the Wenckebach phenomenon, therefore, CSM that produces worsening of the block is “good” because such block is usually at the AV node level. This is more benign than block at the HV level, which leaves the patient with an idioventricular escape rhythm. Improvement of block with CSM may therefore be “bad.”
Carotid Sinus Massage: Normal Values
Sinus pauses of 3 seconds or more in response to CSM are considered abnormal. In a control group of 98 patients without a history of AV block, bradycardia, or syncope (33), the mean sinus interval with firm right or left CSM was 1,252 msec, and +2 standard deviations (SD) was 2,364 msec. Only 1% of patients had pauses of 3 seconds or more, and 2% had second- or third-degree AV block.
Sinoatrial Node Function Tests
Sinus Node Recovery Times
The sinus node is the archetype of an automatic focus. Automatic rhythms are characterized by spontaneous depolarization, overdrive suppression, and postoverdrive “warm-up” or return to baseline cycle length. Pacing at rates in excess of the spontaneous rate of an automatic focus results in temporary inhibition of spontaneous depolarization, with gradual return to the original cycle length over several beats following cessation of pacing (Fig. 61.2) (15,32
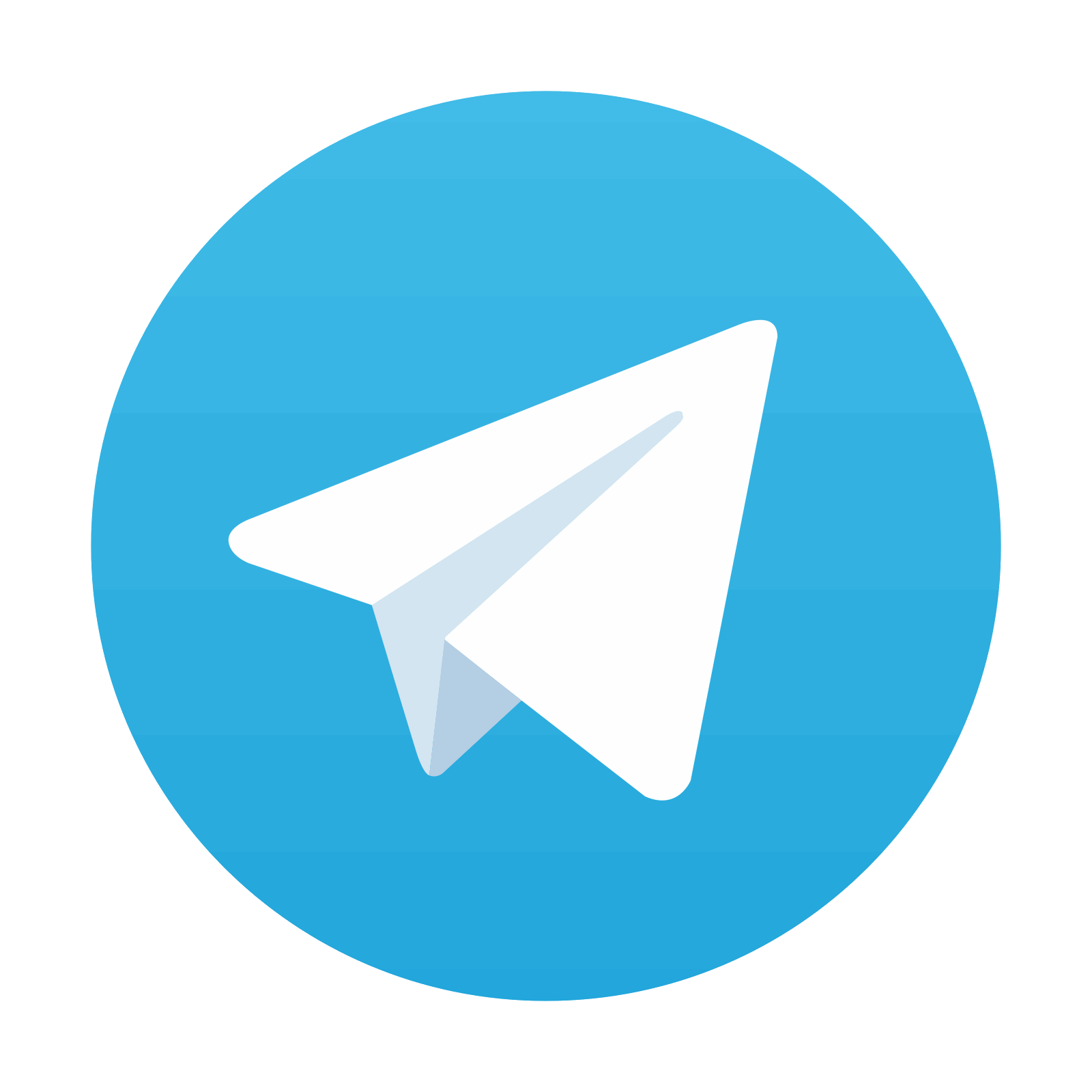
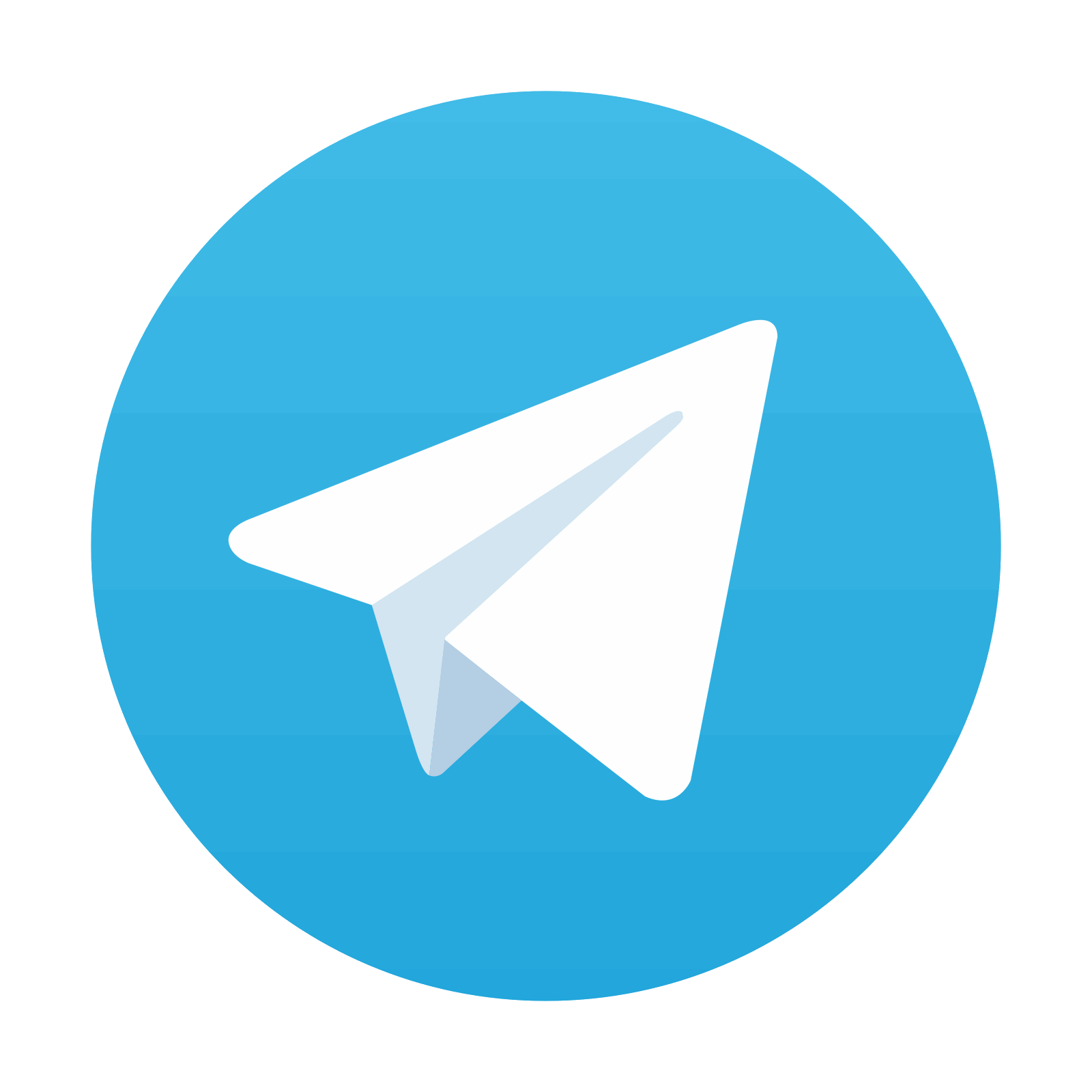
Stay updated, free articles. Join our Telegram channel

Full access? Get Clinical Tree
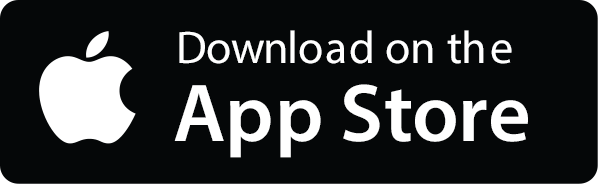
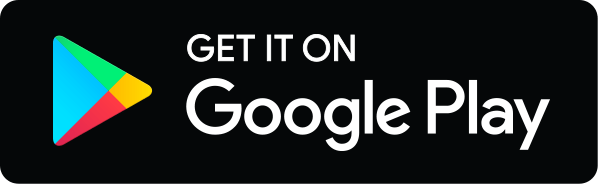
