Patients with early chronic kidney disease (CKD) have an increased risk for cardiovascular disease. Aldosterone levels are elevated and might impair ventricular function through adverse myocardial and vascular proinflammatory and fibrotic effects. In the Chronic Renal Impairment in Birmingham II (CRIB II) study, it was hypothesized that mineralocorticoid receptor blockade with spironolactone in addition to angiotensin-converting enzyme inhibitors or angiotensin receptor blockers would improve left ventricular (LV) function and markers of inflammation, ventricular stretch, and collagen turnover in early CKD. A total of 112 patients with early CKD were randomized to spironolactone 25 mg/day or placebo for 40 weeks. Ventricular function was assessed by echocardiography and cardiac magnetic resonance imaging tagging. High-sensitivity C-reactive protein, N-terminal–pro-B-type natriuretic peptide, and aminoterminal propeptide of type III procollagen were measured. Spironolactone improved LV long-axis systolic function (Sm 8.2 ± 1.4 vs 7.7 ± 1.3 cm/s, p <0.05), torsion (7.77 ± 1.61° vs 6.77 ± 1.48°, p <0.05), and myocardial deformation (strain rate −1.14 ± 0.24 vs −1.09 ± 0.20 s −1 , p <0.05) compared to placebo, without a change in the ejection fraction. Markers of LV relaxation (E/e′ ratio 7.2 ± 2.3 vs 8.5 ± 2.3, p <0.05) and suction (M-mode propagation velocity 56 ± 12 vs 50 ± 12 cm/s, p <0.05) were also improved. Spironolactone reduced N-terminal–pro-B-type natriuretic peptide (24.8 pmol/L [range 0.4 to 122.4] vs 39.4 pmol/L [range 10.8 to 102.4], p <0.01) and attenuated an increase in aminoterminal propeptide of type III procollagen observed with placebo. In conclusion, spironolactone improves markers of regional LV systolic and diastolic function in early CKD.
We have recently demonstrated that the addition of spironolactone to established treatment with the use of angiotensin-converting enzyme inhibitors or angiotensin receptor blockers in patients with early-stage chronic kidney disease (CKD) resulted in a reduction in left ventricular (LV) mass and improved arterial stiffness and aortic distensibility. In a further analysis of prospectively defined secondary end points from the Chronic Renal Impairment in Birmingham II (CRIB II) study, we have now examined the effect of spironolactone on LV function and on serologic markers of inflammation, ventricular stress, and collagen turnover.
Methods
The design and clinical characteristics of patients in CRIB II have been published previously. In brief, 115 patients were recruited from specialist nephrology clinics in Birmingham, England, as part of single-center, prospective, double-blind, randomized, placebo-controlled trial. All patients had CKD using the Kidney Disease Outcomes Quality Initiative classification (4-variable Modification of Diet in Renal Disease formula ); 26 patients had stage 2 (median albumin/creatinine ratio 6.0 mg/mmol [range 2 to 46]), and 89 patients had stage 3 CKD. The largest disease categories were glomerulonephritis (53%), quiescent vasculitis (19%), and adult polycystic kidney disease (8%). All patients were on maximally tolerated treatment with the use of angiotensin-converting enzyme inhibitors and/or angiotensin receptor blockers for ≥6 months, with good blood pressure control (mean daytime 24-hour ambulatory blood pressure <130/85 mm Hg). Exclusion criteria included a history of diabetes; heart failure; symptomatic coronary artery disease; peripheral vascular, cerebrovascular, and renovascular disease; anemia (<120 g/dL); or previous documented hyperkalemia (>5.5 mmol/L).
All patients received 25 mg of spironolactone once daily for a 4-week, open-label run-in phase, after which patients were randomized to a further 36 weeks of treatment with spironolactone or placebo. Biochemical and safety monitoring was performed as previously described. Patients with serious hyperkalemia (potassium ≥6.5 or ≥6.0 mmol/L on repeat testing) were withdrawn. Patients with potassium levels of 5.5 to 5.9 mmol/L received spironolactone 25 mg on alternate days. Clinical assessment and echocardiography were performed before the run-in phase and after 40 weeks of treatment. All subjects gave written informed consent, and the study was approved by the South Birmingham Research Ethics Committee.
Echocardiography (Vivid 7; GE Vingmed Ultrasound AS, Horten, Norway) was performed by a single experienced operator (N.C.E.). All parameters were measured in triplicate according to the recommendations of the American Society of Echocardiography and stored for blinded off-line analysis using a customized software package (EchoPAC; GE Healthcare, Milwaukee, Wisconsin). LV mass was determined using the 2-dimensional area-length formula and indexed to body surface area. Relative wall thickness was calculated as (2 × LV posterior wall)/LV end-diastolic dimension. LV diastolic function at rest was assessed using standard techniques. Peak systolic (s′), early diastolic (e′), and late diastolic (a′) mitral annular velocities were measured at the septal, lateral, inferior, and anterior LV walls with pulsed-wave tissue Doppler. The ratio of early mitral diastolic inflow velocity to average early diastolic mitral annular velocities at the 4 basal annular sites (E/e′) was calculated. Grayscale images were acquired from the apical 4- and 2-chamber views at end-expiration at a frame rate >80 frames/s for 2-dimensional LV longitudinal strain imaging. The endocardial border was manually tracked at end-systole using commercially available software (2D-strain EchoPAC PC version 7.0.1; GE Vingmed Ultrasound AS). Peak systolic strain and peak systolic strain rate were measured for each myocardial segment in triplicate and averaged for global LV data. Ventricular-vascular interaction was assessed by effective arterial elastance, estimated as (end-systolic pressure measured by sphygmomanometry × 0.9)/stroke volume. LV end-systolic elastance was determined using the single-beat technique. The ventricular-vascular coupling ratio (effective arterial elastance/LV end-systolic elastance) was calculated. End-diastolic elastance was estimated as the E/e′ ratio divided by the volume of filling during diastole.
Forty patients were selected randomly to undergo tagging studies on cardiac magnetic resonance (CMR) (1.5-T Symphony; Siemens Medical Solutions, Erlangen, Germany). Three short-axis tagged images at the LV base (mitral valve), middle (papillary muscle), and apex and a long-axis image were acquired using prospective electrocardiographic gating. Spatial modulation of magnetization was used to create a tag grid on the images with a tag separation of 8 mm using a fast field echo multishot sequence (repetition time 3.9 ms, echo time 1.7 ms, voxel size 1.99/2.04/8.00 mm 3 , flip angle 5°, tag grid angle 45° with 12 ms, minimum 15 phases per cardiac cycle), as previously described. Blinded analysis was performed for LV longitudinal shortening, apical and basal rotation, and LV torsion (difference in apical and basal rotation multiplied by the average radius and divided by the distance between the apical and basal slices).
Blood samples for the following biomarkers were measured, with the lower limit of detection, intra-assay coefficient of variation, and interassay coefficient of variation stated, respectively: N-terminal–pro-B-type natriuretic peptide (NT-proBNP), noncompetitive immunoluminometric assay (University of Leicester, Leicester, United Kingdom), 0.3 pmol/L, 2.3%, and 4.8%; high-sensitivity C-reactive protein, Tina-quant CRP (Latex) ultrasensitive assay (Roche Diagnostics, Burgess Hill, United Kingdom), 0.03 mg/L, 2.51% to 5.70%, and 0.28% to 1.34%; intact aminoterminal propeptide of type III procollagen (PIIINP), radioimmunoassay (Orion Diagnostica, Helsinki, Finland), 0.3 μg/L, 7%, and 7%; and carboxy-terminal telopeptide of type I collagen, Elecsys b-CrossLaps assay (Roche Diagnostics), 0.07 ng/ml, 6%, and 8%.
All data are expressed as mean ± SD, absolute change ± SEM, or frequency (percentage), unless otherwise stated. Non-normally distributed values were logarithmically transformed and are presented as medians and interquartile ranges (25th to 75th percentile). Treatment groups were compared using Student’s t tests or chi-square tests (at baseline). Repeated-measures analysis of variance was used to assess changes in individual variables over follow-up with adjustment for baseline differences. Multivariate regression was used to identify the independent predictors of changes in LV function. The model included treatment group, change in systolic blood pressure, age, medication use, and estimated glomerular filtration rate. Intraobserver variability is reported using interclass correlation coefficients: strain 0.96 (95% confidence interval [CI] 0.86 to 0.99), strain rate 0.93 (95% CI 0.75 to 0.98), long-axis s′ velocities 0.99 (95% CI 0.97 to 0.99), left atrial volume index 0.94 (95% CI 0.79 to 0.99), and M-mode propagation velocity 0.96 (95% CI 0.87 to 0.99). All analyses were 2 sided, and significance was judged at p <0.05.
Results
Of the 115 patients recruited for this study, 112 were suitable for randomization after the 4-week open-label run-in phase. Groups were well matched at baseline for demographic and clinical variables and medication use ( Table 1 ).
Variable | Placebo | Spironolactone | p Value |
---|---|---|---|
(n = 56) | (n = 56) | ||
Age (years) | 53 ± 12 | 54 ± 12 | 0.50 |
Men | 33 (59%) | 32 (57%) | 0.70 |
24-hour blood pressure (mm Hg) | 125 ± 10/77 ± 8 | 124 ± 11/76 ± 8 | 0.60/0.50 |
Heart rate (beats/min) | 65 ± 11 | 66 ± 12 | 0.63 |
Serum creatinine (mg/dl) | 1.4 ± 0.4 | 1.5 ± 0.4 | 0.12 |
Estimated glomerular filtration rate (ml/min/1.73m 2 ) | 53 ± 11 | 49 ± 6 | 0.08 |
Plasma renin concentration (μmol/ml) | 83 (34–138) | 68 (42–148) | 0.62 |
Angiotensin II (pg/ml) | 9 (5–18) | 8 (5–21) | 0.10 |
Plasma aldosterone concentration (pg/ml) | 67 (41–91) | 60 (41–79) | 0.57 |
Aminoterminal propeptide type III procollagen (μg/L) | 3.73 ± 0.98 | 4.01 ± 0.77 | 0.07 |
Carboxy-terminal telopeptide type I collagen (ng/ml) | 0.20 (0.13–0.34) | 0.22 (0.15–0.36) | 0.93 |
High-sensitivity C-reactive protein (mg/L) | 1.09 (0.43–4.24) | 2.22 (0.61–4.68) | 0.15 |
N-terminal pro-B-type natriuretic peptide (pmol/L) | 24.7 (2.7–72.9) | 62.1 (10.8–130.8) | 0.14 |
Medications | |||
Angiotensin-converting enzyme inhibitors | 39 | 39 | 0.50 |
Angiotensin receptor blockers | 19 | 19 | 0.55 |
β blockers | 8 | 15 | 0.07 |
Calcium channel blockers | 17 | 13 | 0.28 |
Statins | 17 | 27 | 0.06 |
Diuretics | 13 | 18 | 0.18 |
Twenty-one patients (9 in the spironolactone group) were excluded because of inadequate image quality. Treatment groups were matched for LV geometry (relative wall thickness 0.35 ± 0.06 vs 0.32 ± 0.06, p = 0.07), volumes (LV end-diastolic volume index 46 ± 12 vs 46 ± 9 ml/m 2 , p = 0.23), and LV mass index (88 ± 20 vs 91 ± 22 g/m 2 , p = 0.15). After treatment with spironolactone, there was a decrease in LV mass index (−8.9 ± 1.6 vs 2.2 ± 1.7 g/m 2 , p <0.01) but no change in LV end-diastolic volume or relative wall thickness (data not shown), consistent with previous published results in this group using CMR.
At baseline, LV filling pressures were within normal limits in the 2 groups ( Table 2 ). After treatment with spironolactone, markers of LV relaxation improved, including a reduction in left atrial volume index, attenuation of isovolumic relaxation time, an increase in M-mode propagation velocity (an approximation of the intraventricular pressure gradient and hence LV suction), and an increase in average myocardial early relaxation velocities (e′) ( Figure 1 , Table 2 ). The increase in e′ with spironolactone was responsible for the reduction in the E/e′ ratio, indicating a reduction in LV filling pressure ( Table 2 ). Treatment with spironolactone increased s′ tissue velocities but did not change the ejection fraction. Longitudinal systolic strain in each wall was significantly increased, resulting in a global improvement in LV deformation. Global longitudinal systolic strain rate also improved with spironolactone ( Table 2 ). Effective arterial elastance, LV end-systolic elastance, and LV end-diastolic elastance were all reduced with spironolactone. The arterial-ventricular coupling ratio (LV end-systolic elastance/effective arterial elastance) was maintained in the 2 groups ( Table 2 ).
Variable | Placebo (n = 55) | Spironolactone (n = 55) | ||
---|---|---|---|---|
Week 0 | Week 40 | Week 0 | Week 40 | |
Systolic function | ||||
LV ejection fraction (%) | 63 ± 7 | 66 ± 7 | 63 ± 6 | 66 ± 7 |
Tissue Doppler systolic velocity (cm/s) | 7.6 ± 1.4 | 7.7 ± 1.3 | 7.5 ± 1.5 | 8.2 ± 1.4 ⁎ |
Global peak strain (%) | −17.8 ± 3.0 | −17.9 ± 2.4 | −17.3 ± 3.2 | −19.7 ± 2.7 † |
Global peak strain rate (s −1 ) | −1.01 ± 0.18 | −1.09 ± 0.20 | −1.02 ± 0.23 | −1.14 ± 0.24 ⁎ |
Diastolic function; LV filling pressures | ||||
Transmitral early velocity/M-mode flow propagation velocity | 1.54 ± 0.6 | 1.49 ± 0.5 | 1.40 ± 0.4 | 1.27 ± 0.4 |
Pulmonary atrial velocity duration − transmitral atrial duration (ms) | −21 ± 23 | −25 ± 25 | −17 ± 23 | −34 ± 18 ⁎ |
Left atrial volume index (ml/m 2 ) | 27 ± 6 | 26 ± 6 | 28 ± 5 | 24 ± 6 † |
Diastolic function; LV relaxation | ||||
Ratio of early to late mitral inflow velocities (E/A) | 1.17 ± 0.29 | 1.07 ± 0.32 | 1.08 ± 0.3 | 1.09 ± 0.29 |
Deceleration time (ms) | 223 ± 50 | 235 ± 42 | 216 ± 50 | 227 ± 38 |
Isovolumic relaxation time (ms) | 93 ± 14 | 102 ± 15 | 97 ± 14 | 95 ± 15 ⁎ |
Average septal and lateral annular myocardial TDI velocities (cm/s) | 8.4 ± 2.4 | 8.8 ± 2.5 | 8.1 ± 2.0 | 9.7 ± 2.3 † |
Transmitral E/average annular myocardial TDI velocities | 8.9 ± 2.6 | 8.5 ± 2.3 | 8.7 ± 2.0 | 7.2 ± 2.3 † |
M-mode flow propagation velocity (cm/s) | 50 ± 13 | 50 ± 12 | 50 ± 15 | 56 ± 12 ⁎ |
Ventricular-vascular interaction | ||||
Arterial elastance (mm Hg/ml) | 1.76 ± 0.45 | 1.69 ± 0.38 | 1.79 ± 0.56 | 1.43 ± 0.37 † |
End-systolic elastance (mm Hg/ml) | 2.42 ± 0.79 | 2.45 ± 0.71 | 2.37 ± 0.76 | 1.98 ± 0.59 † |
End-diastolic elastance | 0.12 ± 0.04 | 0.12 ± 0.46 | 0.11 ± 0.04 | 0.09 ± 0.39 ⁎ |
Ratio of arterial elastance to end-systolic elastance | 0.76 ± 0.20 | 0.73 ± 0.21 | 0.79 ± 0.22 | 0.74 ± 0.17 |
† p <0.01 (treatment group–by–time interaction in the repeated-measures analysis of variance after adjustment for baseline differences in age, gender, estimated glomerular filtration rate, blood pressure, heart rate, medications, and NT-proBNP.
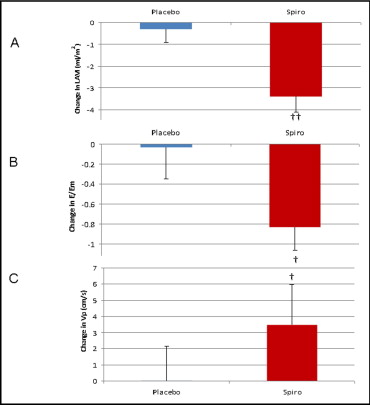
Patients randomized to CMR tagging were younger (48 ± 10 vs 58 ± 11 years, p <0.01) but did not differ in any other characteristic from the other patients recruited to the study. Treatment groups in the tagging study were well matched for age (48 ± 12 vs 46 ± 8 years, p = 0.34), percentage of men (30% vs 33%), estimated glomerular filtration rate (54 ± 13 vs 53 ± 14 ml/min/1.73 m 2 , p = 0.86), and systolic blood pressure (123 ± 13 vs 125 ± 9 mm Hg, p = 0.68). Two data sets were excluded because of poor image quality. Systolic strain and strain rate were significantly improved with spironolactone, consistent with the changes documented on 2-dimensional echocardiography ( Figure 2 , Table 3 ). In addition, diastolic strain rate was improved, consistent with a reduction in diastolic ventricular stiffness. LV peak apical rotation and LV peak torsion were increased after treatment with spironolactone, while basal rotation remained unchanged ( Table 3 ).
