Left ventricular (LV) diastolic filling is characterized by the formation of intraventricular rotational bodies of fluid (termed “vortex rings”) that optimize the efficiency of LV ejection. The aim of the present study was to evaluate the morphology and dynamics of LV diastolic vortex ring formation early after acute myocardial infarction (AMI), in relation to LV diastolic function and infarct size. A total of 94 patients with a first ST-segment elevation AMI (59 ± 11 years; 78% men) were included. All patients underwent primary percutaneous coronary intervention. After 48 hours, the following examinations were performed: 2-dimensional echocardiography with speckle-tracking analysis to assess the LV systolic and diastolic function, the vortex formation time (VFT, a dimensionless index for characterizing vortex formation), and the LV untwisting rate; contrast echocardiography to assess LV vortex morphology; and myocardial contrast echocardiography to identify the infarct size. Patients with a large infarct size (≥3 LV segments) had a significantly lower VFT (p <0.001) and vortex sphericity index (p <0.001). On univariate analysis, several variables were significantly related to the VFT, including anterior AMI, LV end-systolic volume, LV ejection fraction, grade of diastolic dysfunction, LV untwisting rate, and infarct size. On multivariate analysis, the LV untwisting rate (β = −0.43, p <0.001) and infarct size (β = −0.33, p = 0.005) were independently associated with VFT. In conclusion, early in AMI, both the LV infarct size and the mechanical sequence of diastolic restoration play key roles in modulating the morphology and dynamics of early diastolic vortex ring formation.
The assessment of left ventricular (LV) diastolic function usually relies on noninvasive measures of LV relaxation and stiffness. More recently, the evaluation of LV muscle and fluid mechanics, using novel echocardiographic indexes and techniques, has been proposed to refine the assessment of LV diastolic function. In particular, 2-dimensional speckle tracking imaging enables the assessment of the complex torsional mechanics of the left ventricle. During systole, the contraction of the helically arranged subendocardial and subepicardial layers leads to the opposite rotation of the LV apex and LV base, the so-called LV twist. During isovolumic relaxation, the reverse rotation of the LV apex and LV base (LV untwisting) releases the energy stored during LV systole. The restoring forces generate intraventricular pressure gradients contributing to early LV filling. These complex LV mechanics are associated with characteristic intraventricular fluid dynamics. During early LV filling, the blood flow forms intraventricular rotational bodies of fluid (so-called vortex rings) that are critical in optimizing the blood flow during LV ejection. These diastolic fluid dynamics can be noninvasively evaluated using color Doppler echocardiography or contrast echocardiography. In addition, a novel echocardiographic dimensionless index (vortex formation time [VFT]) has recently been introduced to quantitatively characterize the optimal conditions leading to vortex formation.
It is well known that myocardial injuries, such as those induced by acute myocardial infarction (AMI), negatively affect the LV diastolic function ; conversely, not much data regarding the effect of AMI on the VFT or vortex morphology are available. The knowledge of abnormalities involving the LV vortex formation in clinical setting would be useful, because it would provide direct information regarding the ultimate goal of LV performance (i.e., optimal blood flow).
Accordingly, the aim of the present study was to quantify vortex ring formation using the dimensionless index of the VFT and to estimate the morphology of the vortex during routine contrast echocardiography early after AMI. In addition, we sought to correlate the dynamics of vortex ring formation with LV diastolic function, torsional mechanics, and the extent of myocardial damage (i.e., infarct size).
Methods
The population consisted of 110 consecutive patients admitted to the coronary care unit for a first ST-segment elevation AMI. The diagnosis of AMI was determined from typical electrocardiographic changes and/or ischemic chest pain associated with the elevation of cardiac biomarkers. All patients underwent immediate coronary angiography and primary percutaneous coronary intervention. The infarct-related artery was identified by the site of coronary occlusion during coronary angiography and electrocardiographic criteria.
The clinical evaluation included 2-dimensional echocardiography with speckle-tracking analysis to assess the LV systolic and diastolic function, dimensionless index of VFT (see paragraph with equation below), and LV untwisting rate; contrast echocardiography to assess LV vortex morphology; and myocardial contrast echocardiography (MCE) to assess the extent of perfusion abnormalities and infarct size. These echocardiographic examinations were performed 48 hours after primary percutaneous coronary intervention. Subsequently, the relations between VFT and vortex morphology with LV diastolic function, LV untwisting rate, and infarct size (as assessed from MCE) were evaluated.
Patients with significant (moderate or severe) valvular heart disease or rhythm other than sinus were not included.
The local ethical committee approved the study protocol, and all patients provided informed consent.
All patients with AMI underwent imaging in the left lateral decubitus position using a commercially available system (Vivid 7 Dimension, GE Healthcare, Horten, Norway) equipped with a 3.5-MHz transducer. Standard 2-dimensional images and Doppler and color Doppler data were acquired from the parasternal and apical views (2-, 3- and 4-chamber) and digitally stored in cine-loop format. The analyses were subsequently performed off-line using EchoPAC, version 7.0.0 (GE Healthcare, Horten, Norway). The LV end-diastolic and end-systolic volumes were measured according to the Simpson’s biplane method, and the LV ejection fraction was calculated as [(end-diastolic volume − end-systolic volume)/end-diastolic volume] × 100.
As previously reported, transmitral and pulmonary vein pulsed-wave Doppler tracings were used to classify the diastolic function as normal, diastolic dysfunction grade 1 (mild), diastolic dysfunction grade 2 (moderate), diastolic dysfunction grade 3 (severe), or diastolic dysfunction grade 4 (severe).
The VFT, a dimensionless index that characterizes the optimal conditions for vortex formation during diastole was calculated as follows :
where SV is the stroke volume, β the fraction of stroke volume contributed from the atrial component of LV filling and calculated from Doppler spectra of the E and A waves, and D the mitral valve diameter in centimeters. The mitral valve diameter was obtained by averaging the largest mitral orifice diameters measured during early diastolic filling in the 2-, 3-, and 4-chamber apical views.
Speckle tracking analysis was applied to evaluate the LV untwisting rate. Parasternal short-axis images of the left ventricle were acquired at 2 different levels: the basal level, identified by the mitral valve and the apical level, identified as the smallest cavity achievable distally to the papillary muscles (moving the probe down and slightly laterally, if needed). The frame rate was 60 to 100 frames/s, and 3 cardiac cycles for each short-axis level were stored in cine-loop format for off-line analysis (EchoPAC, version 7.0.0, GE Healthcare). The endocardial border was traced at an end-systolic frame, and the region of interest was chosen to fit the whole myocardium. The software allows the operator to check and validate the tracking quality and to adjust the endocardial border or modify the width of the region of interest, if needed. Each short-axis image was automatically divided into 6 standard segments: septal, anteroseptal, anterior, lateral, posterior, and inferior. The software calculated the LV rotation from the apical and basal short-axis images as the average angular displacement of the 6 standard segments, referring to the ventricular centroid, frame by frame. Counterclockwise rotation was marked as a positive value and clockwise rotation as a negative value when viewed from the LV apex. LV twist was defined as the net difference (in degrees) of the apical and basal rotation at the isochronal points. The opposite rotation after LV twist was defined as the LV untwist and the time derivative of the LV untwist was defined as the LV untwisting rate (°/s).
Immediately after 2-dimensional echocardiography, contrast echocardiography was performed using the same ultrasound system. Luminity (Bristol-Myers Squibb Pharma, Brussels, Belgium) was used as the contrast agent. A slow intravenous bolus of echocardiographic contrast (0.1 to 0.2 ml) was administered, followed by 1 to 3 ml of normal saline flush. Apical 3- and 4-chamber views were acquired using a low-power technique (0.1 to 0.4 mechanical index), and the focus was set in the middle of the left ventricle. The machine settings were optimized to obtain the best possible visualization of LV vortex formation. The frame rate was 60 to 100 frames/s, and ≥3 cardiac cycles were stored in cine-loop format for off-line analysis (EchoPAC, version 7.0.0, GE Healthcare). For the evaluation of vortex morphology, the vortex length and width relative to the LV volume were measured during early diastolic filling in the apical 3-chamber view. A vortex sphericity index was calculated as the vortex length/vortex width ratio.
Immediately after contrast echocardiography, MCE was performed to evaluate myocardial perfusion to assess the infarct size after AMI. The same ultrasound system was used, and the 3 standard apical views were acquired using a low-power technique (0.1 to 0.26 mechanical index). The background gains were set such that minimal tissue signal was seen, and the focus was set at the level of the mitral valve. Luminity (Bristol-Myers Squibb Pharma) was used as the contrast agent. Each patient received an infusion of 1.3 ml of echocardiographic contrast diluted in 50 ml of 0.9% NaCl solution through a 20-gauge intravenous catheter in a proximal forearm vein. The infusion rate was initially set at 4.0 ml/min and then titrated to achieve optimal myocardial enhancement without attenuation artifacts. The machine settings were optimized to obtain the best possible myocardial opacification with minimal attenuation. At least 15 cardiac cycles after high mechanical index (1.7) microbubble destruction were stored in cine-loop format for off-line analysis (EchoPAC, version 7.0.0, GE Healthcare). The left ventricle was divided according to a standard 16-segment model, and a semiquantitative scoring system was used to assess contrast intensity after microbubble destruction : 1, normal/homogenous opacification; 2, reduced/patchy opacification; and 3, minimal or absent contrast opacification. A myocardial perfusion index (MPI) was derived by adding the contrast scores of all segments and dividing by the total number of segments. In accordance with the number of segments showing minimal or absent contrast opacification, patients were categorized as having a small infarct size (<3 segments with minimal or absent contrast opacification) or a large infarct size (≥3 segments with minimal or absent contrast opacification).
Continues variables are expressed as the mean ± SD, when normally distributed, and as the median and interquartile range, when non-normally distributed. Categorical data are presented as absolute numbers and percentages. Differences in continuous variables were assessed using the Student t test or the Mann-Whitney U test, as appropriate. The chi-square test or Fisher’s exact test, if appropriate, were computed to assess differences in categorical variables.
Linear regression analyses were performed to evaluate the relation between the VFT and the vortex sphericity index; the vortex parameters and the grade of diastolic dysfunction; the vortex parameters and the LV untwisting rate; and the vortex parameters and infarct size (as assessed from MCE). Univariate and multivariate linear regression analyses (enter model) were performed to evaluate the relation between VFT and the following clinical and echocardiographic variables: age, gender, infarct location (anterior vs nonanterior), multivessel disease, LV end-diastolic volume, LV end-systolic volume, LV ejection fraction, diastolic dysfunction grade, LV untwisting rate, and MPI. Only significant variables on univariate analysis were entered as covariates in the multivariate model. A p value of <0.05 was considered statistically significant. Statistical analysis was performed using the Statistical Package for Social Sciences software package, version 15.0 (SPSS, Chicago, Illinois).
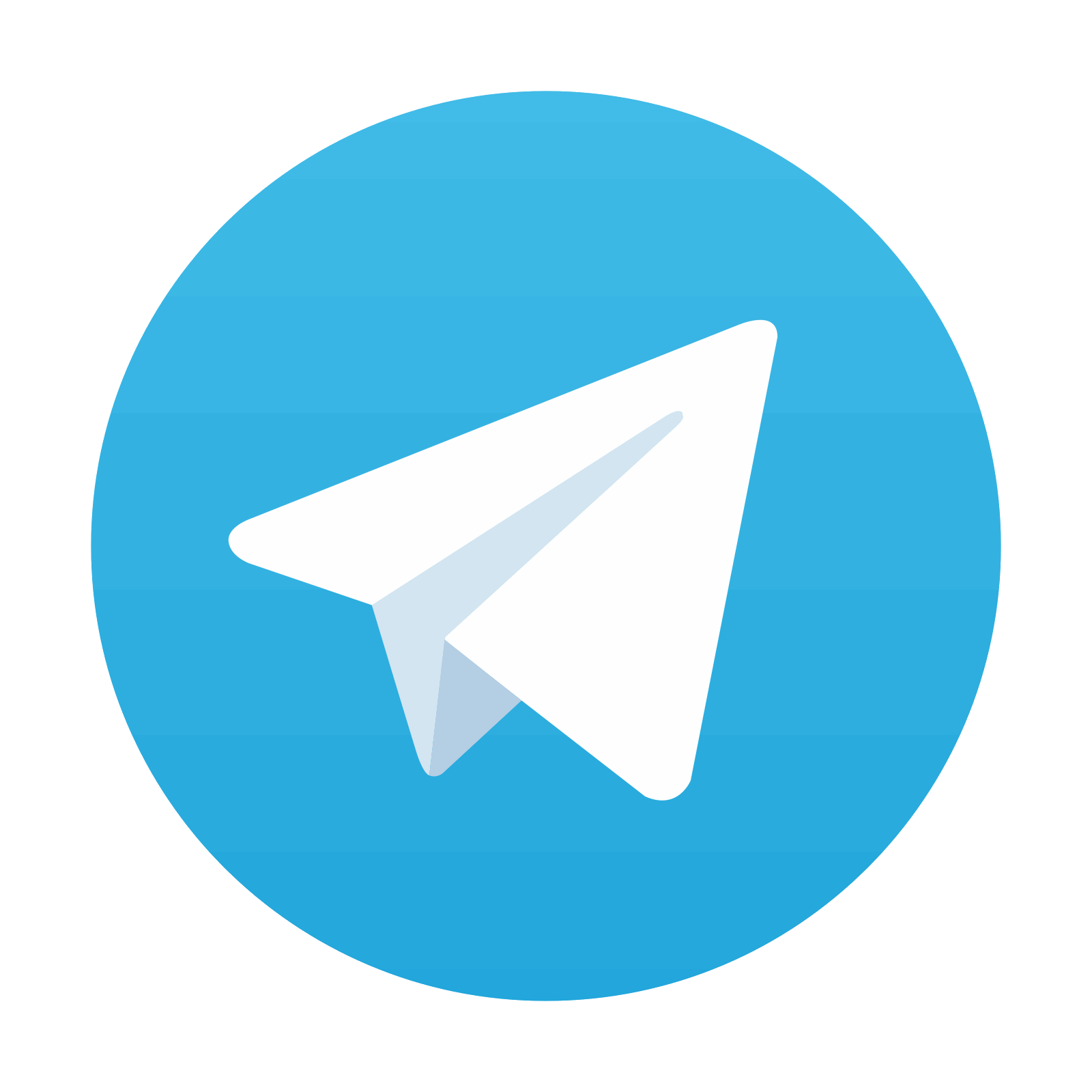
Stay updated, free articles. Join our Telegram channel

Full access? Get Clinical Tree
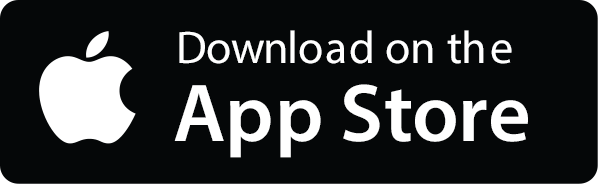
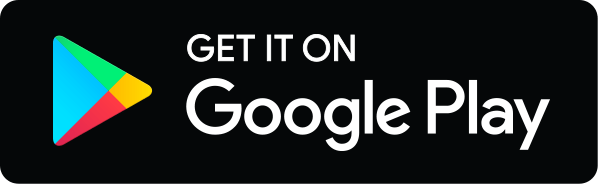