Pulmonary valve regurgitation is the most common complication after complete repair of tetralogy of Fallot. The benefits of pulmonary valve replacement (PVR) on right ventricular (RV) volumes is well established. However, the effect on left ventricular (LV) function is still debated. We aimed to determine the evolution of LV function after PVR and assess the contribution of the interventricular septum (IVS) motion. A total of 21 patients (mean age 30.1 ± 14.1 years) presenting with a history of complete repair of tetralogy of Fallot and requiring PVR prospectively underwent cardiac magnetic resonance imaging before and after PVR to measure the end-diastolic volume (EDV), end systolic volume, and ejection fraction for the LV and RV chambers. Maximal excursion of the IVS was also calculated to quantify abnormal septal motion. The LV-EDV and LV-end systolic volume was 80 ± 27 and 40 ± 19.5 ml/m 2 before PVR and 81.5 ± 23 and 35 ± 14 ml/m 2 after PVR, respectively, leading to a significant increase in LV ejection fraction of 6.1 ± 4.9% (51 ± 8.2% before and 57 ± 6.8% after PVR, p = 0.0003). Also, a significant reduction in RV-EDV (p = 0.0001) and RV end-systolic volume (p = 0.0001) was seen but without improvement in the RV ejection fraction. The maximum IVS excursion decreased after PVR (9.2 ± 3.4 mm before and 6.8 ± 3.6 mm after; p = 0.002). LV ejection fraction improvement correlated with RV-EDV before PVR (ρ = 0.43; p = 0.049). The maximum IVS excursion correlated with RV-EDV before and after PVR but was independent of LV ejection fraction improvement. In conclusion, the results of the present study have demonstrated a significant improvement in LV ejection fraction after PVR that correlated with the pre-PVR RV-EDV but was independent of IVS motion improvement.
Tetralogy of Fallot is 1 of the first congenital heart diseases to have successfully benefited from medical and surgical advances. The long-term survival and quality of life of these patients are excellent. Pulmonary regurgitation is the most common complication. It has a long-term detrimental effect on right ventricular (RV) function and increases the incidence of sudden cardiac death and ventricular tachycardia. Because of ventricular–ventricular interactions, pulmonary regurgitation also has a deleterious effect on left ventricular (LV) function, an additional risk factor for sudden cardiac death. The benefit of pulmonary valve replacement (PVR) on RV volumes, arrhythmia, and exercise intolerance is well established. The effect on LV function is still debated, and only 2 studies have reported on a benefit from PVR on the left ventricle. Ventricular interaction might play a key role, because PVR leads to important modifications in RV volumes and thus to changes in LV filling and interventricular septal (IVS) motion. We sought to evaluate the evolution of LV function after PVR in patients presenting with repaired tetralogy of Fallot and to assess the contribution of RV volumes and IVS motion in this evolution.
Methods
From March 2009 to June 2011, 21 patients (mean age 30.1 ± 14.1 years) with corrected tetralogy of Fallot and scheduled for PVR were prospectively enrolled in the present study. PVR was indicated for symptomatic patients with reduced exercise capacity or when the RV end-diastolic volume (EDV) exceeded 160 ml/m 2 or if an additional malformation required surgery. All patients underwent magnetic resonance imaging before and after surgical PVR. The functional New York Heart Association classification was evaluated before and after PVR. The QRS duration was recorded on the electrocardiogram before and after surgery.
Cardiopulmonary bypass was established using ascending aortic and bicaval cannulation in normothermia. When the procedure was limited to the PVR and reconstruction of the RV outflow tract, it was performed on a beating heart. If another procedure was necessary, the aorta was cross-clamped, and cardioplegia was performed to obtain cardiac arrest. A longitudinal incision was performed into the infundibular patch. The branch pulmonary arteries were sized and treated if necessary. Reduction plasty of the dilated RV infundibulum was performed, if necessary, and consisted of complete resection of the old infundibular patch. Bioprosthetic valve insertion was the preferred surgical option. The xenograft was inserted through the infundibulopulmonary incision and sutured using continuous suture.
Cardiac magnetic resonance studies were performed with a 1.5T System (Intera, Philips Medical Systems, Best, The Netherlands). The cardiac magnetic resonance protocol included cine steady-state free precession sequences in the vertical long-axis, 4-chamber view, RV outflow tract, and short-axis view covering the entirety of both ventricles. The acquisition parameters were excitation time 1.8 ms, repetition time 3.6 ms, flip angle 60°, slice thickness 7 mm, spacing between slices 5 mm, matrix 224 × 224, spatial resolution 1.6 × 1.6 × 7 mm, variable field of view, and 30 images per cardiac cycle. Measurements of the LV and RV volumes were performed by manual segmentation of the short-axis cine images at end-diastole and end-systole using dedicated software. The ejection fraction was calculated as follows: (ejection fraction = (EDV − end-systolic volume)/EDV). All volumes were indexed to the body surface area. The volumes and ejection fraction were compared with the standards published by Alfakih et al. Interventricular septum excursion (IVS) was calculated according to the method published by Muzzarelli et al ( Figure 1 ). First, a short-axis cine slice, located at the level of the papillary muscles of the left ventricle (the first midventricle slice from the base without any ventricular septal defect patch) was selected. For each phase of the cardiac cycle, a line (line a) was manually drawn, connecting the epicardial surface of the anterior and posterior RV–LV hinge points. At the midpoint of this line, a second line (line b) was set perpendicularly. The distance, along line b, between line a and the RV endocardial border of the septum was measured. RV trabeculations were excluded. The maximum displacement of the IVS was calculated by subtracting the maximum and minimum distance obtained during the cardiac cycle. IVS excursion was considered abnormal if the maximal excursion was >2 mm. Abnormal displacement of IVS was classified as systolic (excursion >2 mm during systole) and/or diastolic (excursion >2 mm during diastole).
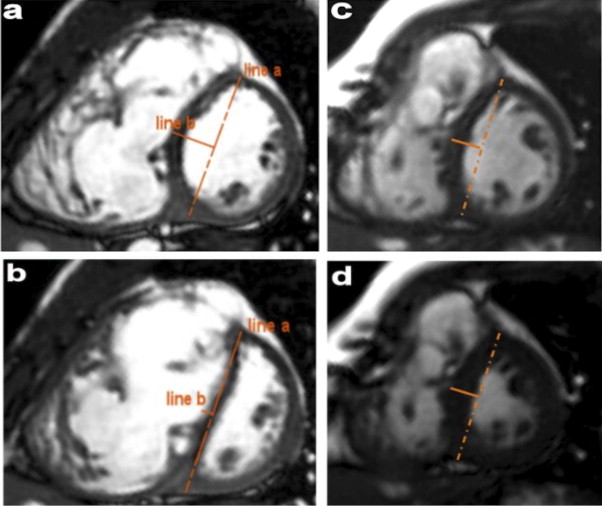
Descriptive data are presented as the mean ± SD or median, as appropriate. A Wilcoxon test was performed to compare the parameters before and after PVR. To assess interobserver variability, 10 randomly selected patients were selected. Two independent observers (A.C. and I.S.) performed the ventricular and septal measurement on these patients. The intraclass correlation coefficients with the 95% confidence intervals (CIs) were calculated for the left and right ventricles and septal measurements. Spearman’s test was performed to assess the correlation between the ventricular parameters before and after PVR (EDV, end-systolic volume, ejection fraction, and IVS excursion). Statistical analysis was performed using Stata, version 10.0 (StataCorp, College Station, Texas).
Our institutional ethics review board granted permission to proceed with the present study, and all patients provided informed consent.
Results
The patient characteristics are listed in Table 1 . After PVR, the New York Heart Association class had improved significantly from a median of 2 to a median of 1 ( Figure 2 ). Of the 21 patients, 19 had improvement in their functional class and 2 remained unchanged. All patients had prolongation of the QRS duration and a right bundle branch block pattern. After PVR, no modification in QRS duration (161 ± 24 vs 160.5 ± 27 ms; p = 0.8) was seen.
Pt. No. | Gender | Previous Palliation | Primary Repair | Age at Primary Repair (y) | PVR (mm) | Additional Procedures | Age at PVR (y) | NYHA | RV-EDV (ml/m 2 ) | RV-ESV (ml/m 2 ) | RVEF (%) | LV-EDV (ml/m 2 ) | LV-ESV (ml/m 2 ) | LVEF (%) | IVS Excursion (mm) | ||||||||
---|---|---|---|---|---|---|---|---|---|---|---|---|---|---|---|---|---|---|---|---|---|---|---|
Before | After | Before | After | Before | After | Before | After | Before | After | Before | After | Before | After | Before | After | ||||||||
1 | F | 0 | IP | 1.3 | PX 23 | RVOT-An | 13.9 | 2 | 1 | 320 | 148 | 203 | 89 | 36.4 | 39.4 | 75 | 98 | 30 | 34 | 60.1 | 64.8 | 15.6 | 11.7 |
2 | M | 0 | TAP | 2.7 | Hancock25 | PPA | 26.2 | 1 | 1 | 332 | 150 | 243 | 95 | 26.6 | 36.5 | 100 | 105 | 60 | 46 | 39.6 | 56.1 | 15.6 | 13.5 |
3 | F | 0 | TAP | 1.3 | PX 25 | RVOT-An | 16.7 | 2 | 1 | 151 | 98 | 108 | 72 | 28.6 | 26.0 | 47 | 50 | 19 | 23 | 59.3 | 53.6 | 10.0 | 6.3 |
4 | M | Left BT | TAP | 7.3 | PX 27 | PPA | 40.6 | 2 | 1 | 125 | 110 | 107 | 84 | 14.0 | 23.3 | 54 | 51 | 21 | 16 | 59.9 | 67.3 | 6.2 | 2.2 |
5 | F | 0 | IP | 4.9 | PX 25 | 0 | 33.9 | 1 | 1 | 176 | 132 | 131 | 91 | 25.6 | 30.9 | 49 | 64 | 19 | 17 | 60.1 | 72.2 | 7.6 | 5.1 |
6 | M | Potts | TAP | 29.4 | PX 25 | RVOT-An | 66.9 | 2 | 1 | 159 | 126 | 107 | 98 | 32.2 | 22.2 | 50 | 67 | 23 | 28 | 53.7 | 58.1 | 5.1 | 3.1 |
7 | F | 0 | IP | 2.4 | PX 25 | RVOT-An | 33.0 | 2 | 1 | 215 | 98 | 143 | 75 | 33.4 | 23.8 | 88 | 79 | 44 | 40 | 49.9 | 49.2 | 9.1 | 3.4 |
8 | F | Waterston | TAP | 5.3 | PX 23 | 0 | 27.4 | 3 | 2 | 368 | 241 | 327 | 205 | 11.1 | 15.0 | 99 | 95 | 60 | 47 | 39.1 | 50.7 | 13.8 | 13.8 |
9 | F | 0 | TAP | 2.4 | PX 25 | 0 | 15.6 | 2 | 1 | 215 | 127 | 120 | 84 | 43.8 | 33.8 | 77 | 84 | 37 | 32 | 51.7 | 62.1 | 10.0 | 8.5 |
10 | F | 0 | IP | 1.5 | PX 23 | 0 | 22.9 | 1 | 1 | 214 | 124 | 136 | 84 | 36.4 | 31.8 | 75 | 80 | 31 | 29 | 58.4 | 63.5 | 4.2 | 4.2 |
11 | M | 0 | TAP | 0.8 | PX 25 | 0 | 14.9 | 2 | 1 | 300 | 163 | 222 | 108 | 25.7 | 33.6 | 108 | 118 | 60 | 55 | 44.0 | 53.1 | 11.1 | 10.3 |
12 | M | Left BT | IP | 9.1 | PX 27 | TVR | 48.0 | 3 | 2 | 254 | 100 | 165 | 72 | 35.0 | 28.3 | 154 | 127 | 100 | 74 | 34.7 | 42.0 | 8.5 | 3.6 |
13 | M | Left BT | TAP | 5.3 | PX 25 | PPA | 29.0 | 1 | 1 | 156 | 102 | 106 | 70 | 31.9 | 31.4 | 82 | 81 | 45 | 36 | 45.7 | 54.7 | 10.3 | 7.3 |
14 | M | Right BT | TAP | 2.2 | PX 25 | PPA; RVSD | 14.3 | 2 | 1 | 184 | 118 | 123 | 85 | 32.9 | 28.1 | 58 | 88 | 24 | 36 | 57.8 | 59.1 | 8.2 | 4.8 |
15 | F | 0 | TAP | 3.9 | PX 23 | 0 | 20.9 | 1 | 1 | 151 | 81 | 92 | 64 | 38.5 | 21.1 | 75 | 54 | 30 | 21 | 59.8 | 61.0 | 9.6 | 7.9 |
16 | F | Left BT | TAP | 7.1 | PX 25 | EP | 38.6 | 2 | 2 | 213 | 117 | 132 | 73 | 38.0 | 37.0 | 74 | 79 | 34 | 35 | 54.3 | 55.3 | 5.7 | 4.9 |
17 | M | 0 | TAP | 4.6 | PX 27 | 0 | 26.8 | 1 | 1 | 154 | 141 | 93 | 107 | 39.2 | 24.1 | 51 | 73 | 28 | 34 | 45.8 | 52.7 | 9.3 | 9.1 |
18 | M | Left BT | TAP | 4.8 | PX 27 | 0 | 35.6 | 2 | 1 | 278 | 128 | 197 | 102 | 29.0 | 20.0 | 69 | 39 | 43 | 19 | 37.4 | 49.3 | 11.5 | 9.1 |
19 | F | Potts | TAP | 13.0 | PX 23 | 0 | 48.7 | 3 | 1 | 308 | 92 | 203 | 63 | 34.0 | 31.3 | 105 | 80 | 52 | 33 | 50.0 | 57.8 | 7.5 | 3.8 |
20 | F | Right BT | TAP | 2.7 | PX 21 | EP | 14.5 | 2 | 2 | 170 | 141 | 133 | 96 | 21.3 | 31.8 | 67 | 86 | 31 | 36 | 53.7 | 58.1 | 11.0 | 8.1 |
21 | M | Left BT | TAP | 9.0 | PX 25 | RVSD | 43.5 | 1 | 1 | 183 | 132 | 107 | 75 | 41.1 | 43.1 | 116 | 124 | 57 | 54 | 50.8 | 56.0 | 2.7 | 1.3 |
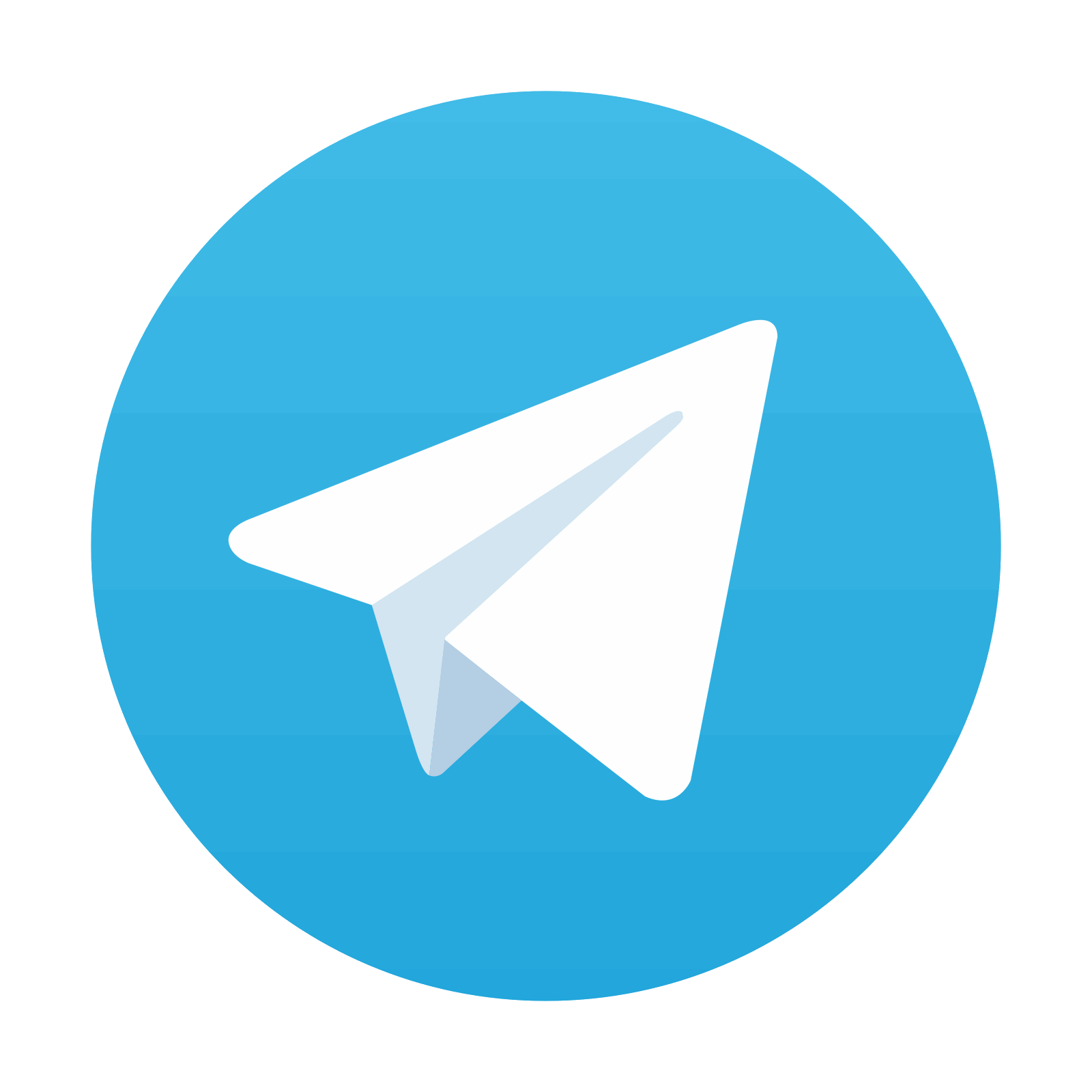
Stay updated, free articles. Join our Telegram channel

Full access? Get Clinical Tree
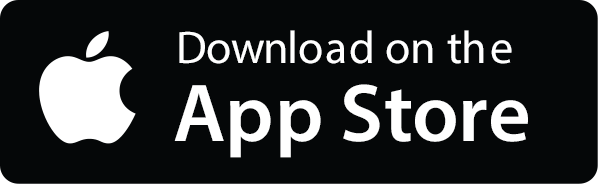
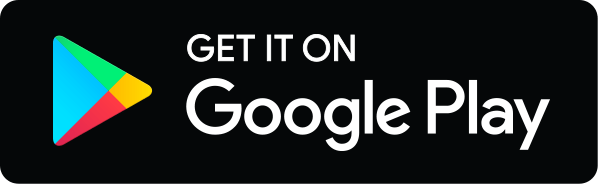
