Although numerous strategies for radiation dose decrease in coronary computed tomographic angiography are effective, their combined impact on diagnostic performance is not known. We therefore assessed the effect of a standardized coronary computed tomographic angiographic protocol on diagnostic accuracy. We evaluated 80 consecutive patients from 3 sites with coronary computed tomographic angiography and quantitative coronary angiography. All sites initially used nonstandardized protocols; 2 sites then initiated a standardized protocol, and 1 site continued its nonstandardized protocol as a time-overlapping control. Two blinded readers interpreted coronary computed tomographic angiographic studies; a third obtained consensus. A blinded core laboratory performed quantitative coronary angiography. Each segment was graded as <50% or ≥50% diameter stenosis. Compared to those using nonstandardized protocols (n = 35), studies using standardized protocols (n = 45) had a trend to increased use of prospective gating (p = 0.09), lower voltage (p <0.01), decreased current (p <0.01), and shorter scan length (p <0.01). Median (interquartile range) radiation dose decreased from 5.7 mSv (4.0 to 10.8) to 2.0 mSv (1.3 to 3.4, p <0.001). There were no significant differences in sensitivity (100%, 20 of 20, vs 100%, 18 of 18, p = 1.0), specificity (93%, 14 of 15, vs 85%, 23 of 27, p = 0.61), or accuracy (97%, 34 of 35, vs 91%, 41 of 45, p = 0.27) by patient; sensitivity (83%, 33 of 40, vs 83%, 25 of 30, p = 0.93), specificity (92%, 86 of 93, vs 92%, 134 of 146, p = 0.85), or accuracy (89%, 119 of 133, vs 90%, 159 of 176, p = 0.80) by artery; or sensitivity (80%, 44 of 55, vs 72%, 26 of 36, p = 0.74), specificity (94%, 332 of 353, vs 94%, 499 of 531, p = 0.96), or accuracy (92%, 376 of 408, vs 93%, 525 of 567, p = 0.80) by segment. In conclusion, a standardized dose-decrease protocol for coronary computed tomographic angiography decreases radiation dose without affecting diagnostic performance.
Coronary computed tomographic angiography imparts non-negligible ionizing radiation doses, with large variations reported between sites. Multiple techniques have been developed for decrease of radiation dose to minimize potential risk of future cancer. These techniques include tube current modulation for studies with retrospective electrocardiographic (ECG) gating, prospective ECG gating, decreased 100-kV voltage (vs standard 120-kV voltage), and lower tube current. The effects of these methods on diagnostic performance have generally been examined separately, whereas their combined impact on diagnostic performance remains largely unexplored. We hypothesized that coronary computed tomographic angiographic (CCTA) performance with a standardized protocol incorporating multiple dose-decrease techniques would result in lower radiation dose with preserved diagnostic accuracy compared to nonstandardized clinical protocols. We therefore compared radiation dose and diagnostic accuracy between patients scanned with nonstandardized and standardized protocols in an intent-to-diagnose fashion, including all patients, vessels, and segments for final efficacy analysis.
Methods
This study prospectively evaluated 80 consecutive patients undergoing 64-detector coronary computed tomographic angiography and invasive coronary angiography (ICA) at 3 sites. All patients were adults ≥18 years in age and were referred for clinically indicated ICA for chest pain or angina-equivalent symptoms (n = 67) or for heart failure symptoms (n = 13). All CCTA and invasive coronary angiographic studies were performed within 60 days; median intertest delay was 3 days (interquartile range 1 to 7). Exclusion criteria included history of coronary artery bypass grafting, renal insufficiency (creatinine ≥1.5 mg/dl), known pregnancy, or contraindication to β blockers or iodinated contrast. All patients were included in intent-to-diagnose fashion, irrespective of body mass index (BMI) and baseline or postmedication heart rate or rhythm. All sites had approval by their respective institutional review boards with informed consent or an approved waiver of consent per site protocol. Sites 1 and 2 enrolled 10 and 15 patients using nonstandardized clinical protocols followed by 17 and 28 patients using the standardized protocols, respectively. Site 3 served as a time-overlapping control and used its own nonstandardized clinical protocol throughout the study period; this site enrolled 5 patients before and 5 patients after initiation of the standardized protocol at the other 2 sites.
We performed an a priori power analysis to test the noninferiority of the standardized protocol on per-segment diagnostic accuracy. Because there is a potential for decreased accuracy using combined dose-decrease strategies, which may be similar to differences in accuracy between scanner generations, we used results from a recent meta-analysis to estimate values for power analysis. This study reported segment-based accuracies for 16-slice and 64-slice coronary computed tomographic angiography as 89% and 95%, respectively. We therefore used the mean accuracy of these 2 cohorts (92%) as a conservative estimate of baseline diagnostic accuracy and assessed for the ability to detect a similar 6% decrease in per-segment accuracy. Our power analysis determined that, to detect a decrease in per-segment diagnostic accuracy of 6% at 85% power, 390 segments would be required in each group.
Before initiation of the standardized protocol, each site used distinct nonstandardized clinical CCTA protocols. Two sites (sites 1 and 2) simultaneously initiated a standardized protocol that mandated scan settings based on BMI and heart rate. A third site (site 3) continued to perform coronary computed tomographic angiography by its nonstandardized protocol and was blinded to the standardized protocol at the other sites.
The standardized protocol ( Table 1 ) prescribed 100-kV tube voltage to all nonobese patients (BMI <30 kg/m 2 ) and 120-kV voltage to all obese patients (BMI ≥30 kg/m 2 ). Tube current was assigned based on BMI. Further, the protocol minimized scan length from the carina to the apex of the heart. The standardized protocol prescribed prospective ECG gating to patients with a regular heart rate <65 beats/min. Exceptions to prospective ECG gating were permitted if there was a clinical indication for retrospective ECG gating (e.g., to assess left ventricular function) or for patients with faster (≥65 beats/min) or irregular (variability ≥5 beats/min) heart rates. Further, the standardized protocol mandated the minimum required current pulse duration (time the current is turned on during diastole) for studies with prospective ECG gating in patients with regular and controlled heart rates. ECG dose modulation was performed for all studies using retrospective ECG gating throughout the study period.
BMI (m/kg 2 ) | Voltage (kV) | Current (mA) |
---|---|---|
<25 | 100 | 450 |
25–29.9 | 100 | 550 |
30–33.9 | 120 | 625 |
≥34 | 120 | 625–800 |
Patients received oral and/or intravenous β blockers, if needed, to attempt to achieve heart rates <65 beats/min and were given sublingual nitroglycerin 0.4 mg immediately before the study. All CCTA examinations were performed with the Discovery HD 750 scanner (GE Healthcare, Milwaukee, Wisconsin). All sites used a triple phase-contrast protocol: iodixanol (GE Healthcare, Princeton, New Jersey) 60 ml, followed by 75 ml of a 50:50 mixture of iodixanol and saline, followed by a saline flush 50 ml. Scan parameters included a rotation time of 350 ms, collimation of 64 × 0.625 mm, tube voltages of 100 to 120 kV and 275 to 800 mA.
Two blinded, independent CCTA readers interpreted all studies; a third blinded, independent reader achieved consensus for any segments with discordance. All readers had several years of experience interpreting several thousand studies. Studies were read using AW 4.4 Advantage Workstations (GE Healthcare, Milwaukee). Use of axial datasets, maximum intensity projections, curved multiplanar reformats, and volume-rendered reconstructions were at the discretion of each reader. Using a standard 17 segment model, segments were graded as <50% or ≥50% maximal luminal diameter stenosis compared to the most normal proximal lumen. All segments that were present and evaluable on invasive coronary angiogram were evaluated on coronary computed tomographic angiogram regardless of size. All stents were assessed and included in all analyses. Any segments nonevaluable by coronary computed tomographic angiography were assumed to exhibit ≥50% stenosis in an intent-to-diagnose fashion. Signal and noise were measured on axial images of the aortic root (1.0-cm region at level of the left main artery) and in the proximal portion of each of the 4 coronary arteries; mean values were used to determine study signal, noise, and signal-to-noise ratio. A blinded, experienced core laboratory (GBJM) (Vancouver, British Columbia, Canada) performed quantitative coronary angiography (QCA) for invasive coronary angiographic studies. The maximal diameter stenosis of each segment was measured as a continuous variable on quantitative coronary angiogram.
Radiation dose for coronary computed tomographic angiography was determined using the dose–length product, which was converted to millisieverts by multiplying the product by the conversion factor of 0.014 mSv/(mGy · cm), as has been previously recommended. Radiation doses for diagnostic ICA (excluding studies with interventional procedures) were available in 32 cases. Estimated radiation dose for ICA was determined from the dose–area product, and this was converted to millisieverts by multiplying this product by a conversion factor of 0.22 mSv/(Gy · cm 2 ).
The primary analysis compared all studies performed using nonstandardized protocols to studies performed using standardized protocols. Because there was a potential for differences between sites, secondary analyses were also performed that limited patients to those enrolled at the 2 sites that initiated the standardized protocol. Comparisons between groups were performed regarding patient and scan parameters, radiation dose, and diagnostic performance of coronary computed tomographic angiography compared to QCA. Comparisons of continuous variables were performed with t tests or Mann-Whitney U tests for variables with normal and non-normal distributions, respectively. Chi-square tests were used to compare categorical variables. Power analysis was performed with G*Power 3.1.0 (Kiel, Germany). All other analyses were performed with SPSS 18.0 for Macintosh (SPSS, Inc., Chicago, Illinois). A 2-tailed p value <0.05 was deemed statistically significant.
Results
Patient characteristics stratified by cohort are listed in Table 2 . In the 80 patients, there were 309 arteries and 975 segments that were present and evaluable by QCA. By QCA, stenosis ≥50% was observed in 38 of 80 patients (48%). Stents were present in 53 of 975 segments (5%). Studies using the standardized protocol had a trend to increased use of prospective ECG gating, with lower tube voltage, decreased current, and shorter scan length. The combination of prospective ECG gating and decreased 100-kV tube voltage was observed in 5 of 35 (14%) and 24 of 45 (53%) studies using nonstandardized and standardized protocols, respectively (p = 0.002). There was a 65% decrease in median radiation dose between patients undergoing coronary computed tomographic angiography by the standardized protocol and by nonstandardized protocols ( Table 3 ). Comparative radiation doses between sites are provided in Figure 1 .
Variable | Nonstandardized Protocols (n = 35) | Standardized Protocol (n = 45) | p Value |
---|---|---|---|
Age (years) | 55 ± 15 | 63 ± 12 | 0.01 |
Men | 77% | 71% | 0.54 |
Body mass index (kg/m 2 ) | 29 ± 4 | 27 ± 4 | 0.09 |
Heart rate (beats/min) | 56 ± 13 | 56 ± 10 | 0.99 |
Heart rate variability (beats/min) | 4 ± 7 | 6 ± 12 | 0.50 |
Hypertension | 54% | 38% | 0.14 |
Hyperlipidemia | 63% | 58% | 0.65 |
Diabetes mellitus | 20% | 22% | 0.81 |
Known coronary artery disease | 23% | 33% | 0.30 |
Current tobacco use | 17% | 13% | 0.64 |
Variable | Nonstandardized Protocols (n = 35) | Standardized Protocol (n = 45) | p Value |
---|---|---|---|
Scan parameters | |||
Prospective gating | 66% | 82% | 0.09 |
100 (vs 120) kV | 17% | 60% | <0.001 |
Current (mA) | 582 ± 72 | 529 ± 79 | 0.003 |
Scan length (cm) | 14.6 ± 1.4 | 13.8 ± 1.4 | 0.009 |
Radiation dose | |||
Dose–length product (mGy × cm) ⁎ | 404 (284–771) | 144 (93–241) | <0.001 |
Effective dose (mSv) | 5.7 (4.0–10.8) | 2.0 (1.3–3.4) | <0.001 |
Signal and noise | |||
Signal (HU) | 392 ± 129 | 474 ± 124 | 0.01 |
Noise (HU) | 38 ± 15 | 45 ± 16 | 0.03 |
Signal-to-noise ratio | 11 ± 4 | 11 ± 3 | 0.72 |
Study interpretability | |||
Per patient | 94.3% (33/35) | 93.3% (42/45) | 0.86 |
Per artery | 95.5% (127/133) | 97.7% (172/176) | 0.27 |
Per segment | 96.5% (394/408) | 98.8% (560/567) | 0.02 |
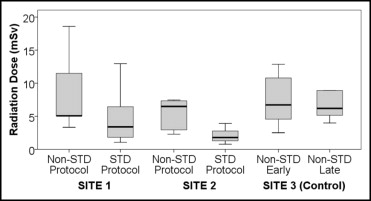
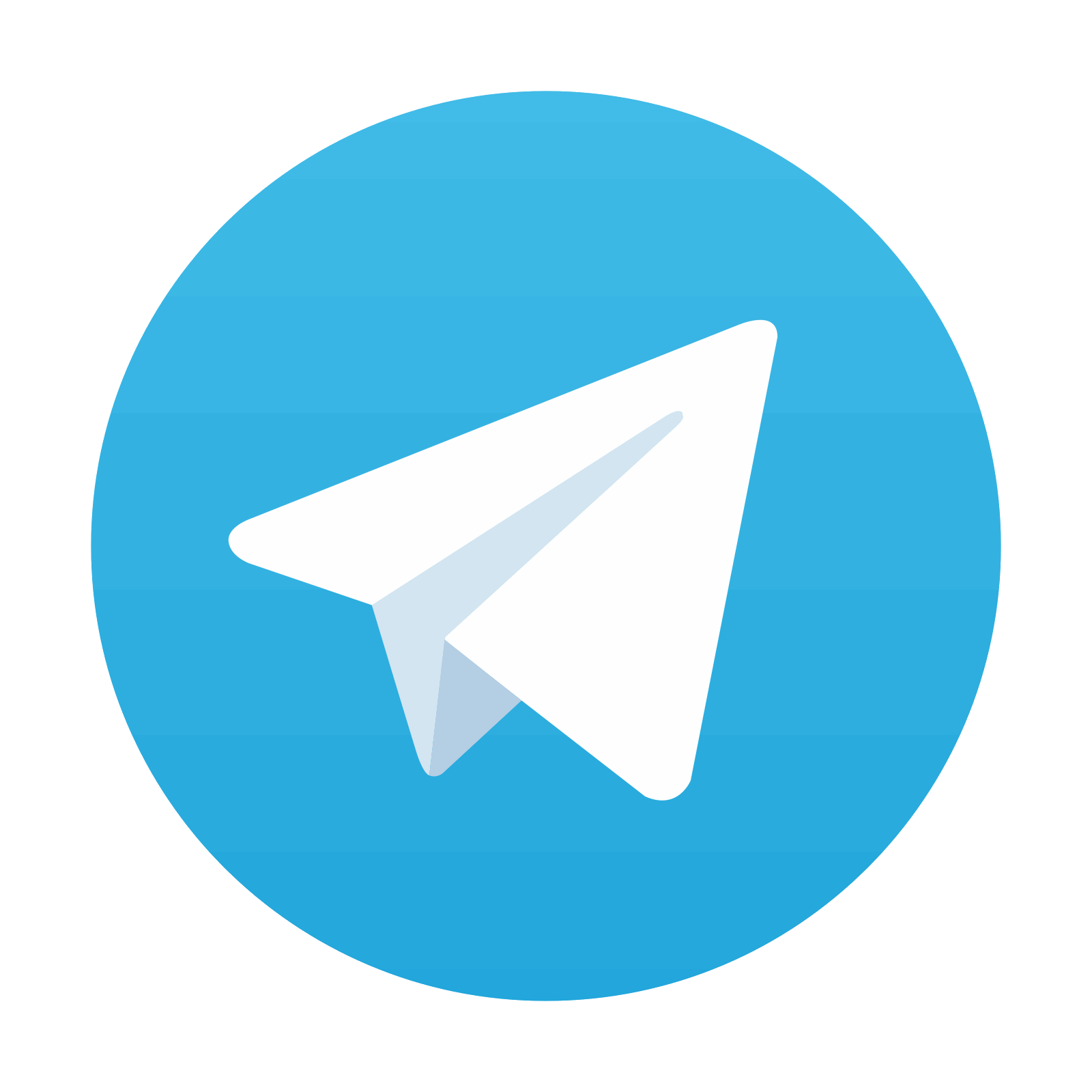
Stay updated, free articles. Join our Telegram channel

Full access? Get Clinical Tree
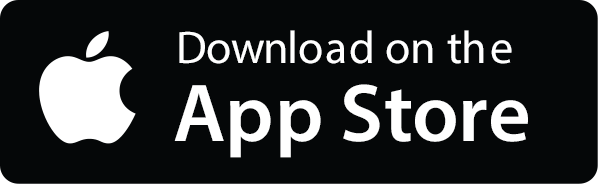
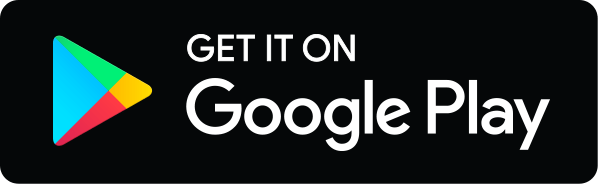
