Early Detection of Lung Cancer Using Bronchoscopy
Annette McWilliams
Stephen Lam
Although the overall lung cancer survival is poor with only 15% of patients surviving 5 years after diagnosis,1 patients with tumors <2 cm have a 5-year survival of 77%.1 The survival of those with preinvasive or microinvasive bronchial cancers is even better at >90%.2 Over the last 2 decades, the largest gain in life expectancy in lung cancer patients is among those with localized disease versus those with regional or distant metastasis.3 Clearly, strategies to improve outcome by detecting and treating the disease in the preinvasive or localized stages is needed.
There are unique challenges to localize preneoplastic lesions or early cancers in the lung. In contrast to other epithelial organs such as the oral cavity, cervix, or colon, the lung consists of a complex branching system of conducting airways leading to alveoli with a surface area the size of a tennis court. In addition, instead of a single cell type, lung cancer consists of several cell types and they are preferentially located in different parts of the tracheobronchial tree. Squamous cell carcinoma and small cell carcinoma, accounting for approximately 45% to 50% of the lung cancers are preferentially located in the central airways (trachea, main, lobar, and segmental bronchi). Adenocarcinoma, accounting for approximately 40% to 50% of all lung cancers, is preferentially located in the peripheral airways inaccessible to standard fiberoptic/video bronchoscopes because of the size of these instruments with an outer diameter of ≥4.9 mm. Ultrathin bronchoscopes with an outer diameter of 2.9 mm are available, but they cannot reach peripheral airways ≤2 mm in diameter. Thus, there is no single method that can scan the entire bronchial epithelium and allow tissue sampling for pathological diagnosis and molecular profiling.
DETECTION OF EARLY CANCER IN CENTRAL AIRWAYS
Rapid evaluation of the central airways became possible with the development of flexible fiberoptic white-light bronchoscopy by Dr. Shigeto Ikeda in the late 1960s. There has been continuing technological advancement over the last 40 years. The development of videobronchoscopy, with better image resolution and quality, is now replacing the former fiberoptic systems. However, early central lung cancers remain difficult to detect with white-light bronchoscopy even with the improved image capability of videobronchoscopy.4 Recent incorporation of optical zoom or magnifying lenses may enhance the examination of the bronchial mucosa and improve the detection of early vascular changes that can be associated with early malignant change.5 Other developments that can be used in adjunct with white-light imaging for localization of preneoplastic lesions and early lung cancer include autofluorescence bronchoscopy (AFB), narrow-band imaging (NBI), and optical coherence tomography (OCT). The recent development of endobronchial ultrasound is an additional tool for the bronchoscopic assessment of central early lung cancers.
Autofluorescence Reflectance Bronchoscopy Auto fluorescence reflectance bronchoscopy has been shown in multiple studies to significantly increase the detection of preneoplastic lesions and carcinoma in situ when used as an adjunct to white-light bronchoscopy6,7,8,9,10,11,12,13,14,15,16,17,18,19,20,21,22,23,24,25,26 (Table 19.1). In addition, it is important in the staging of early central lung cancers prior to curative endobronchial therapy.27,28 It enables the bronchoscopist to obtain a more accurate visualization of the margins and assessment of lesion size.27,28
This technique utilizes the spectral differences in fluorescence and absorption properties of normal and dysplastic bronchial epithelium and has served as the basis for the design of several autofluorescence imaging devices for localization of early lung cancer.6,12,13 More recent versions of these devices use a combination of reflectance and fluorescence for imaging.14,22,23,25,29,30
AFB was first developed in the early 1990s at the British Columbia Cancer Research Centre in Vancouver, British Columbia, and became commercially available in 1998.10 The LIFE-Lung system (Xillix Technologies, Vancouver, BC) used a helium-cadmium laser for illumination (442 nm) and detected
the emitted red and green autofluorescent light with two image-intensified charge-coupled device (CCD) cameras. Normal areas appear green, and abnormal areas appear reddish brown because of reduced green autofluorescence in preneoplastic and neoplastic lesions.
the emitted red and green autofluorescent light with two image-intensified charge-coupled device (CCD) cameras. Normal areas appear green, and abnormal areas appear reddish brown because of reduced green autofluorescence in preneoplastic and neoplastic lesions.
TABLE 19.1 Results of Multicenter Clinical Trials and Randomized Studies of Autofluorescence Bronchoscopy | |||||||||||||||||||||||||||||||||||||||||||||||||||||||||||||||
---|---|---|---|---|---|---|---|---|---|---|---|---|---|---|---|---|---|---|---|---|---|---|---|---|---|---|---|---|---|---|---|---|---|---|---|---|---|---|---|---|---|---|---|---|---|---|---|---|---|---|---|---|---|---|---|---|---|---|---|---|---|---|---|
|
Subsequent improvements in technology made it possible to use nonimage intensified CCDs for detection. Two secondgeneration devices approved by Food and Drug Administration (FDA) made use of a combination of fluorescence and reflectance to enhance contrast between normal and abnormal tissues (Table 19.2). The Onco-LIFE system (Novadq Technologies, Richmond, Canada) utilizes a combination of reflectance and fluorescence imaging. Blue light (395 to 445 nm) and small amount of red light (675 to 720 nm) from a filtered mercury arc lamp is used for illumination (Fig. 19.1). A red reflectance image is captured in combination with the green autofluorescence image to enhance the contrast between premalignant, malignant, and normal tissues as well as to correct for differences in light intensities from changes in angle and distance of the bronchoscope from the bronchial surface.14 Using reflected near infrared red light as a reference has the theoretical advantage over reflected blue light in that it is less absorbed by hemoglobin and hence less influenced by changes in vascularity associated with inflammation. The D-Light system (Karl Storz Endoscopy of America, Culver City, California) consists of a red/green/blue (RGB) CCD camera and a filtered Xe lamp (380 to 460 nm). It combines an autofluorescence image from wavelengths >480 nm with a blue reflectance image.25 Frame averaging is used to amplify the weak autofluorescence signal.
TABLE 19.2 Autofluorescence Imaging Systems | |||||||||||||||||||||||||||||||||
---|---|---|---|---|---|---|---|---|---|---|---|---|---|---|---|---|---|---|---|---|---|---|---|---|---|---|---|---|---|---|---|---|---|
|
These earlier autofluorescence systems were developed to be used with fiberoptic bronchoscopes. With the advanced CCD sensor technology and more widespread use of videobronchoscopy, autofluorescence systems that can be used with videobronchoscopes have been developed (Table 19.2). The Pentax SAFE-3000 system (Pentax Corporation, Tokyo, Japan) uses a semiconductor laser diode that emits 408 nm wavelength light for illumination and detects autofluorescence using a single high-sensitivity color CCD sensor in the fluorescence spectrum 430 to 700 nm (Fig. 19.2). Reflected blue light is used to generate a fluorescence-reflectance image. The white-light and fluorescence images can also be made displayed simultaneously.29 The Olympus autofluorescence imaging bronchovideoscope system (Olympus Corporation, Tokyo, Japan) uses blue light (395 to 445 nm) for illumination. An
autofluorescence image (490 to 700 nm) as well as two reflectance images, one green (550 nm) and one red (610 nm) are captured sequentially and integrated by a videoprocessor to produce a composite image.30
autofluorescence image (490 to 700 nm) as well as two reflectance images, one green (550 nm) and one red (610 nm) are captured sequentially and integrated by a videoprocessor to produce a composite image.30
![]() FIGURE 19.1 White-light (left) and autofluorescence bronchoscopy (right) images of a carcinoma in situ (CIS) lesion in the left main bronchus. No abnormality was seen under white-light examination. Under fluorescence imaging, the CIS lesion as an area of decreased fluorescence. (See color plate.) |
A reduction in specificity has been associated with the increased sensitivity for detection of early lesions. However, there is some data that shows that areas with abnormal autofluorescence contain increased chromosomal aberrations despite a benign histopathology, and that the presence of multiple areas of abnormal autofluorescence is an indicator of overall increased lung cancer risk.31,32 Recently, the use of a quantitative score during autofluorescence examination has been shown to improve specificity.33
Optical Coherence Tomography Currently, there are two imaging modalities with sufficient spatial resolution and tissue depth penetration to address the relatively high falsepositive AFB results and to determine whether the tumor has already invaded through the basement membrane (Fig. 19.3). Confocal microendoscopy offers spatial resolution down to the submicron range, but epithelial cells do not autofluoresence strong enough to allow detection without application of a contrast agent.34,35 In addition, because contact with the bronchial surface is required, fragile epithelium can be scraped off during imaging. Motion artifacts caused by cardiac pulsation and respiratory movements can also lead to suboptimal confocal imaging of cellular details. OCT is a promising micron-scale-resolution method that may be more suitable for rapid endoscopic imaging. OCT is a noncontact method that delivers near infrared light to the tissue and allows imaging of cellular and extracellular structures from analysis of the backscattered light with a spatial resolution of 3 to 15 µm and a depth penetration of approximately 2 mm to provide near-histological images in the bronchial wall.36,37,38,39 Preliminary studies showed that invasive cancer can be distinguished from carcinoma in situ (CIS), and that dysplasia can be distinguished from metaplasia, hyperplasia, or normal.39,40,41 A progressive increase in the epithelial thickness was found to parallel the severity of the histopathology grade. The nuclei of the cells also became darker and less light scattering in lesions that were moderately dysplastic or worse. The basement membrane became disrupted or disappeared with invasive carcinoma.41 However, CIS could not be distinguished from high-grade dysplasia. Therefore, systems with higher resolution and Doppler capability and polarization sensitivity that can measure tissue microstructures in greater detail and quantify microvascular blood flow are needed.42 Doppler OCT (DOCT) systems already exist that can detect extremely slow blood flow (<20 µm/sec in blood vessels as small as ∽15 µm diameter).43,44 DOCT’s unprecedented micron-scale spatial resolution and
ability to monitor functional blood flow parameters at the microvascular level should prove valuable for (a) structural and functional lesion assessment,45 (b) differential diagnosis/staging (invasion through the basement membrane and hence may no longer be curable by endoscopic therapy such as electrocautery treatment),39,41 and (c) therapeutic feedback monitoring during endobronchial therapy such as photodynamic therapy.44,46
ability to monitor functional blood flow parameters at the microvascular level should prove valuable for (a) structural and functional lesion assessment,45 (b) differential diagnosis/staging (invasion through the basement membrane and hence may no longer be curable by endoscopic therapy such as electrocautery treatment),39,41 and (c) therapeutic feedback monitoring during endobronchial therapy such as photodynamic therapy.44,46
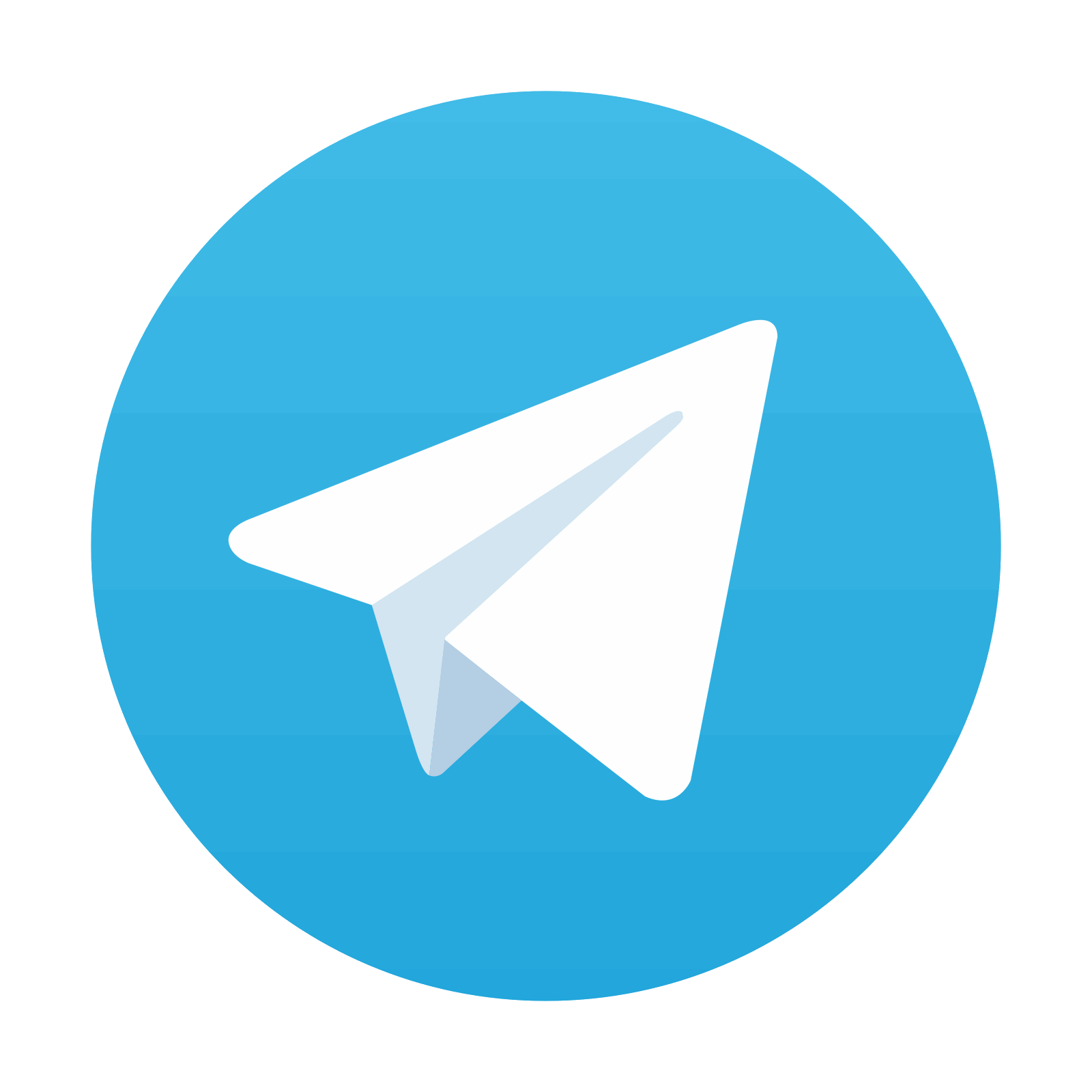
Stay updated, free articles. Join our Telegram channel

Full access? Get Clinical Tree
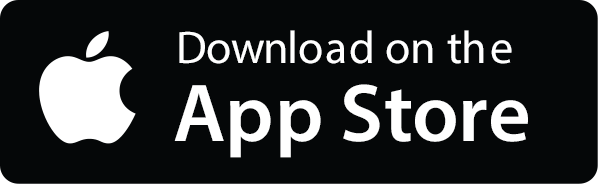
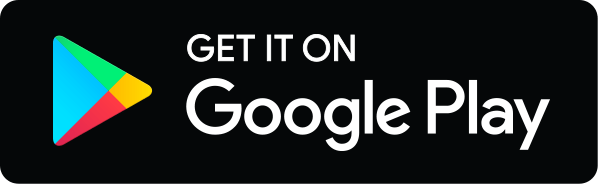