Classification
RAR
PSV
PST
Normal
<3.5
<120 cm/s
Absent
<60% stenosis
≤3.5
>180 cm/s
Absent
>60% stenosis
>3.5
>180 cm/s
Present
Occlusion
N/A
N/A
N/A
Low velocity, low amplitude parenchymal signals
Renal size, as reflected by the ultrasound-measured length of the kidney, is useful in ascertaining potential intervention for renal artery stenosis. It has been shown that renal length decreases significantly in the presence of severe renal artery stenosis [32, 33]. Caps and his colleagues reported that kidneys with a renal artery peak systolic velocity greater than 400 cm/s, and cortical end-diastolic velocities less than or equal to 5 cm/s, were at high risk for progression to renal atrophy (Fig. 47.1) [34]. An atrophic kidney, usually defined as less than 8 cm in length, is unlikely to benefit from revascularization procedures. Although some clinicians have been aggressive in such circumstances, the results with either surgical or endovascular revascularization have been disappointing as reperfusion of the ischemic kidney is not likely to reverse parenchymal changes [35–37]. On the other hand, studies suggest that improved renal function may be expected when intervention is performed prior to progression of the lesion to critical levels of renal impairment [36] or in cases where renal function has acutely deteriorated [38, 39]. However, measurement of renal length alone is not a sensitive enough indicator to detect significant renal artery stenosis and progression to arterial occlusion [34]. Therefore, measurement of kidney length is useful to assist in the proper selection of patients for revascularization but inadequate by itself in defining the presence of a hemodynamically significant renal artery stenosis.
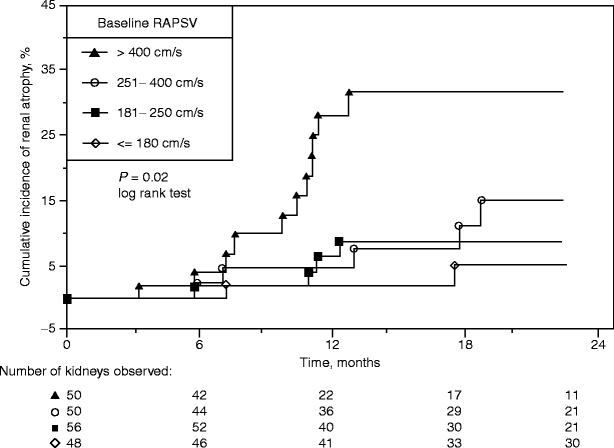
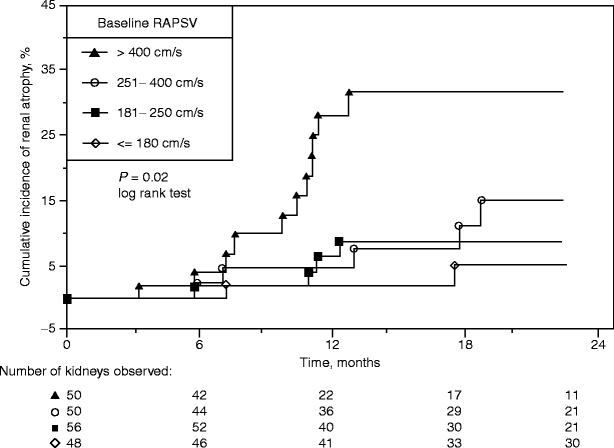
Fig. 47.1
Cumulative index of renal atrophy stratified according to baseline renal artery peak systolic velocity (RAPSV, in cm/s). Standard error is <10% through 24 months for all plots (Reprinted from Caps et al. [34]. With permission from Nature Publishing Group)
Duplex Ultrasound Evaluation of the Renal Arteries
Anatomy of the Renal Arterial System
The kidneys are located between the 12th thoracic and 3rd lumbar vertebrae lying retroperitoneally in the dorsal abdominal cavity. The right kidney usually lies slightly more inferior to the left. While the kidneys decrease in size with increasing age, the normal organ length is 8–13 cm with a width of 5–7 cm. In less than 1% of the population, the kidneys may be joined at their lower poles by an isthmus of tissue which lies anterior to the aorta at the level of the fourth or fifth lumbar vertebrae, forming a horseshoe-shaped organ [40].
For the purpose of sonographic interrogation, the kidneys are segmented into four main areas. The renal artery, vein, and ureter enter the kidney through the renal hilum that forms the renal sinus. The sinus, which in large part is comprised of fat and some fibrous tissue, contains the renal artery and vein, and the collecting and lymphatic systems. For this reason, it is normally brightly echogenic on sonographic imaging (Fig. 47.2). The parenchymal tissue of the kidney is divided into two parts: the medulla and cortex. The cortex constitutes the outermost area of the kidney lying just beneath the renal capsule. Cortical tissue (columns of Bertin) lies between the triangular-shaped medullary pyramids which carry urine from the cortex to the renal pelvis. The 12–18 pyramids generally have lower echogenicity than the cortex and are usually seen well in the normal adult patient.
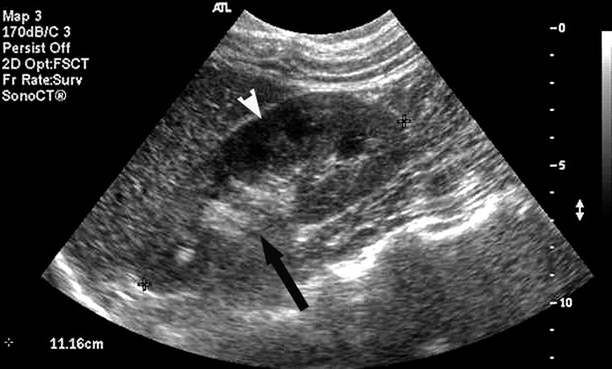
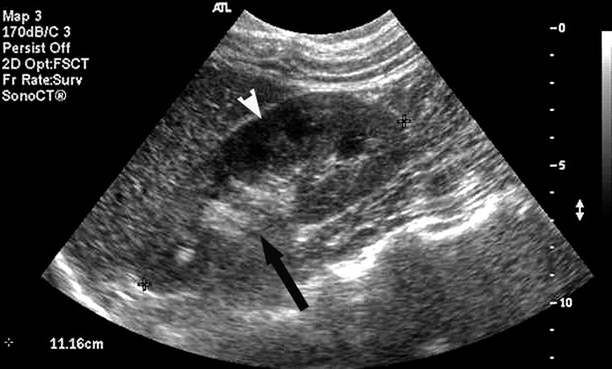
Fig. 47.2
Longitudinal B-Mode image of a normal adult kidney demonstrates echogenicity of the renal sinus (black arrow) and definition of the renal cortex and pyramids (white arrowhead)
Attention to both surface and internal anatomic landmarks facilitates sonographic localization of the renal arteries. Using a surface landmark, the renal arteries are located approximately 2 cm below a transverse plane (the transpyloric plane) located midway between the suprasternal notch and the symphysis pubis. This plane cuts through the lower border of the first lumbar vertebrae, the ninth costal cartilages and the pylorus. The renal arteries can be visualized arising from the lateral or postero-lateral wall of the abdominal aorta. Soon after its origin, the right renal artery will arch inferiorly and then slightly posteriorly to go behind the inferior vena cava (IVC) and the right renal vein. The left renal artery usually originates from the aortic wall slightly more cephalad than the right and courses superiorly and then posterior to the splenic artery, left renal vein and is crossed by the inferior mesenteric vein.
Between 12% and 22% of patients have variant anatomy which includes duplication of the main renal arteries or accessory polar arteries, a feature found more often on the left than on the right [41, 42]. In the majority of patients, the accessory renal arteries arise from the aortic wall below the main renal artery and course to the polar surfaces of the kidney while the main renal arteries enter the kidney through the renal hilum. Occasionally, accessory renal arteries originate from the common or internal iliac, superior or inferior mesenteric, adrenal, or right hepatic arteries.
The renal artery normally gives rise to two to five anterior and posterior segmental branches, which supply blood to upper-, mid-, and lower poles of the kidney (Fig. 47.3). The segmental arteries give rise to interlobar arteries which course along the sides of the renal pyramids. At the level of the corticomedullary junction, the interlobar arteries branch into the arcuate arteries, which travel across the superior border of the pyramids and give rise to the interlobular arteries. The interlobular arteries course toward the surface of the kidney forming the afferent glomerular arterioles.
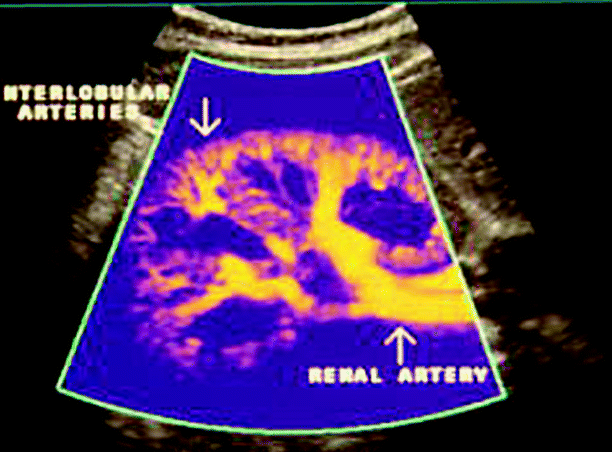
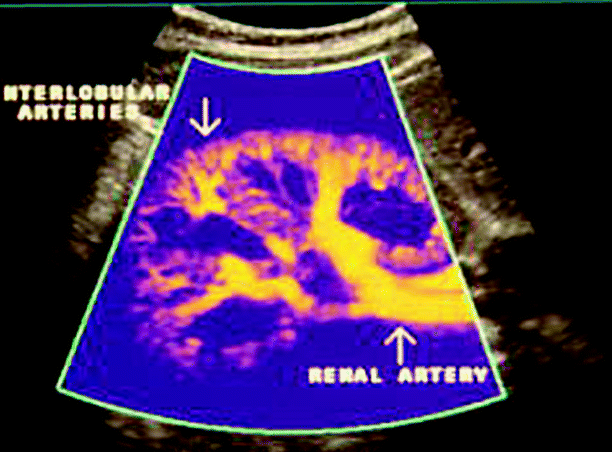
Fig. 47.3
Transverse color flow image of the vasculature of a normal kidney demonstrating anterior and posterior segmental branches of the main renal artery. Courtesy of Philips Ultrasound
On each side, the renal vein courses anteriorly from the renal hilum with the ureter arising posteriorly. The renal artery normally lies between the vein and the ureter. On the right, the renal vein has a short course from the hilum of the kidney to the IVC. While venous anatomy may be anomalous in many patients (primarily due to multiple veins), the left renal vein normally courses anterior to the aorta and posterior to the superior mesenteric artery (Fig. 47.4). In 3% of patients, the left renal vein may take a completely retroaortic path while up to 18% of patients will demonstrate a bifid (circumaortic) left renal vein with both a retroaortic branch and another branch coursing anterior to the aorta [43, 44]. It is important to be aware of these anatomic anomalies because the left renal vein is used as a major anatomic landmark for locating the renal arteries during the sonographic examination.
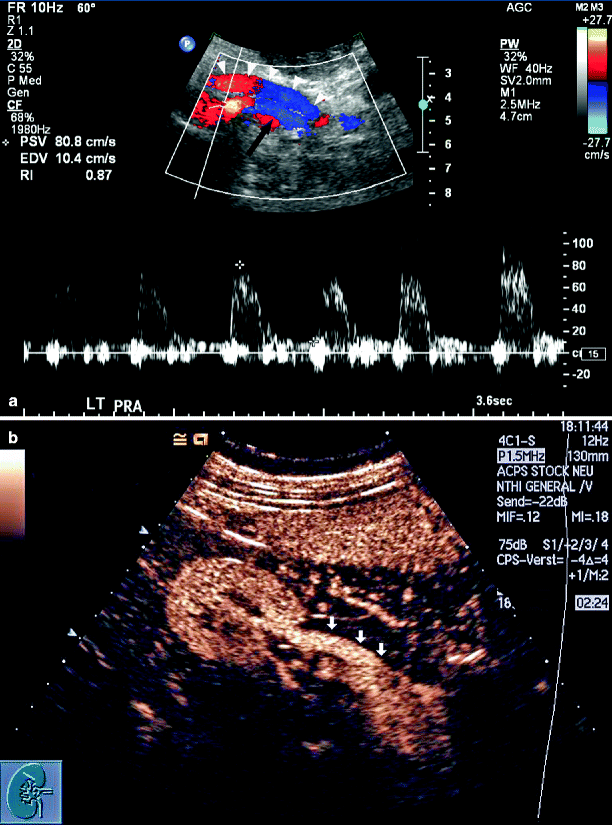
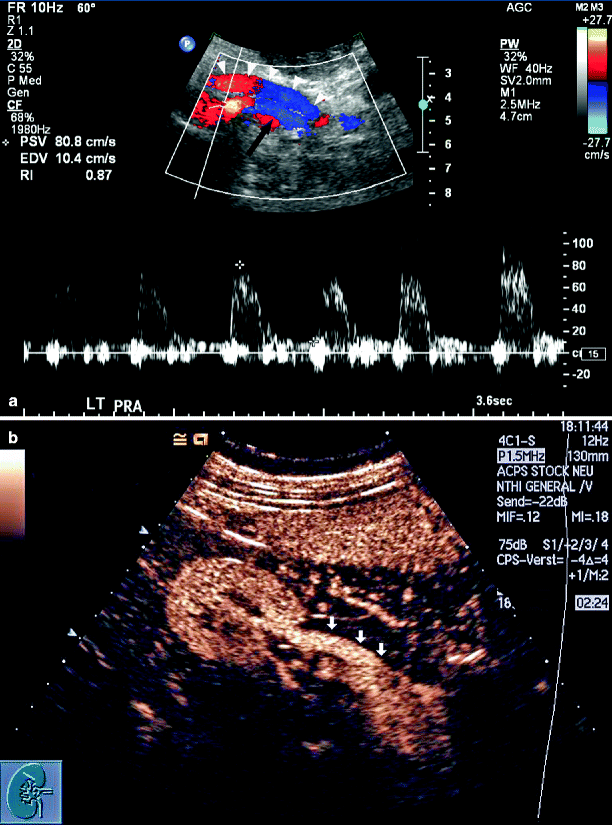
Fig. 47.4
(a) Transverse color flow image of the abdominal aorta, superior mesenteric artery, portal vein confluence, IVC, and left renal vein. Note the left renal vein (white arrowheads) crossing over the renal artery (black arrow) and anterior aortic wall. (b) A longitudinal view of the right renal vein (arrows) following intravenous contrast (Image courtesy of Konrad Stock, M.D.)
Technique
Patient Preparation and Positioning
Although patients are required to fast for 6–8 h prior to the sonographic examination to reduce excessive abdominal gas that would make visualization of the renal arteries and veins more difficult, we have not found it necessary to use cathartics. Patients are allowed to take their usual medications with small sips of water. Diabetic patients are permitted to have dry toast and clear liquids in the morning of the study in order to prevent development of hypoglycemia while awaiting their sonogram. Because of this concern, diabetic patients are also the first ones scheduled in the morning. It is recommended that all patients refrain from smoking or chewing gum because these activities increase the amount of swallowed air in the stomach.
Once in the vascular laboratory, the patient is placed on the examination table in the supine position with the head slightly elevated and the feet lower than the heart. This allows the viscera to descend into the lower abdomen and pelvis, increasing the likelihood of finding acceptable acoustic windows. With the patient in this position, the aorta, mesenteric, and proximal-to-mid segments of the renal arteries are visualized. For examination of the distal renal arteries, renal veins, and the kidneys themselves, the patient is moved to the right or left lateral decubitus position with the arm raised over their ear and their legs extended to elongate the body. A prone position, using intercostal scan planes, may occasionally be required for a thorough examination of the kidneys and distal renal arteries. In patients with a low rib cage, it may also be helpful to have them raise their arms over their head to elevate the ribs and allow improved access to the proximal renal arteries.
Equipment
A high-resolution ultrasound system with pulsed Doppler transducers ranging in frequency from 2.25 to 5.0 MHz is required to allow adequate penetration to the depths of the aorta and distal renal arteries. Although color flow and power Doppler imaging are not absolutely required for successful renal vascular examination, these technologies will greatly facilitate visualizing the vessels, identification of regions of flow disturbance, and confirmation of arterial and/or venous occlusion. The sonographer must optimize the B-Mode, color, and spectral Doppler information throughout the examination because velocities may vary over small regions of the renal arterial and venous systems due to tortuosity and short-segment lesions, dissections, or webs.
Examination of the Aorta, Mesenteric, and Renal Arteries
The examination is initiated with evaluation of the aorta beginning at the level of the diaphragm and continuing to its bifurcation. B-Mode imaging is used to determine the presence of atherosclerotic plaque, aneurysmal dilation, or dissection. The evaluation is complemented with color flow imaging to highlight any regions of disturbed flow. Selective Doppler spectral waveforms are recorded at the level of the celiac and superior mesenteric artery origins. The aortic peak systolic velocity is documented and retained for later utilization in calculation of the renal to aortic velocity ratios.
Branches of the celiac and superior mesenteric arteries may course in close proximity and may be mistaken for accessory or main renal arteries. To help prevent such confusion, Doppler spectral waveforms may be recorded from both the celiac and superior mesenteric arteries with the primary purpose being recognition of the mesenteric flow patterns. A secondary benefit would be detection of unsuspected mesenteric occlusive disease.
The aorta is thereafter imaged in the cross-sectional plane at the level of the superior mesenteric artery. Just inferior to this level, the left renal vein can be identified as it crosses anterior to the aorta (Fig. 47.4). Although this vein serves as a useful landmark for locating the renal arteries, care must be taken to identify anomalous venous anatomy. The vein diameter and flow patterns should be assessed to rule out thrombosis or extrinsic compression due to overlying small bowel or superior mesenteric artery compression syndrome.
Although the renal arteries usually arise from the mid-lateral or posterolateral wall of the aorta at the level of the second lumbar vertebrae, just posterior to the left renal vein, there can be much anatomic variability in their location (Fig. 47.5a). The location of accessory renal arteries is even more unpredictable and therefore easy to miss. Color flow imaging facilitates the identification of the arteries and makes it easier to follow their course from the origin to the mid-segment of the vessel. When neither color flow nor power Doppler allows satisfactory vessel identification, the use of intravenous ultrasound contrast can be helpful.
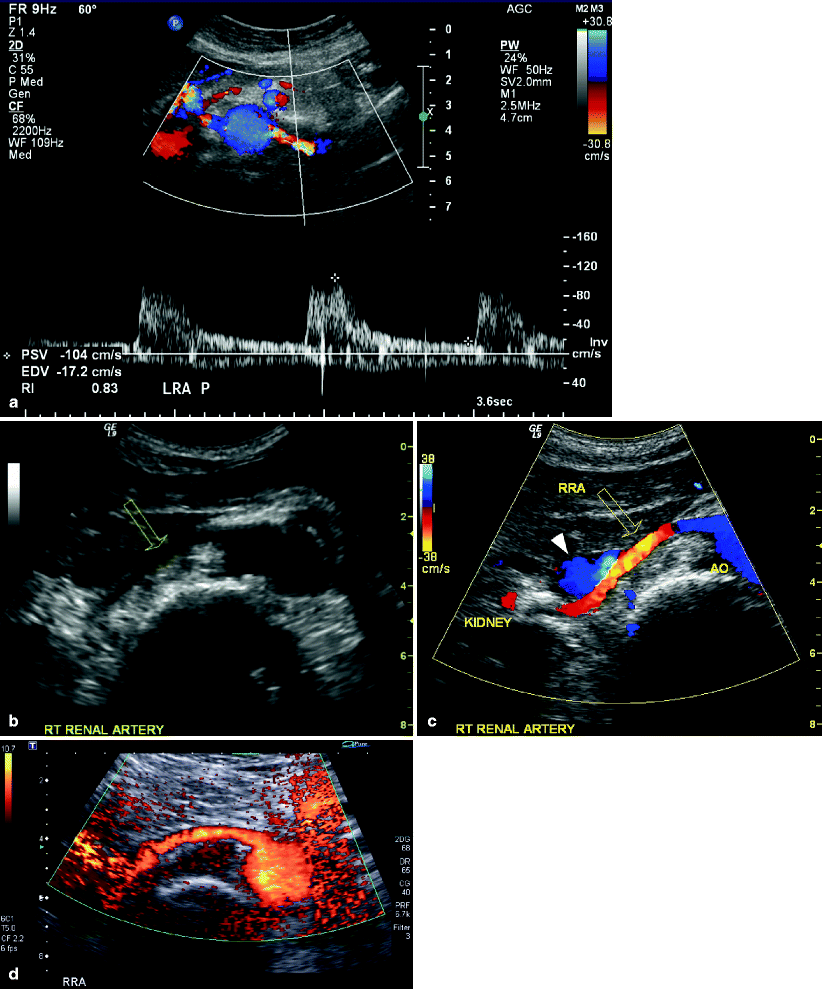
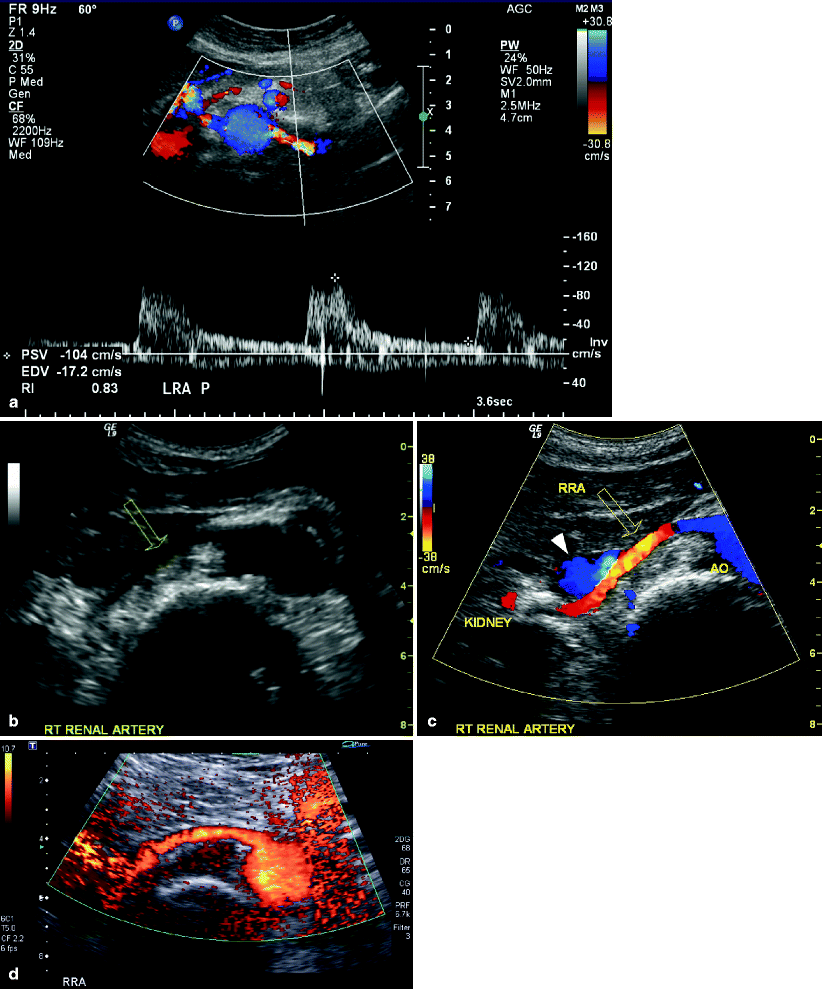
Fig. 47.5
(a) Color flow image of the abdominal aorta and left renal artery demonstrating appropriate angle correction for demonstration of Doppler spectral waveforms within the renal artery. A low resistance spectral pattern is evident which is characteristic of normal renal arterial blood flow. (b) Particularly on the right side, it may be more difficult to identify the full length of the renal artery (open arrow). (c) The use of color Doppler greatly improves visualization of the right renal artery (open arrow), particularly as it goes posterior to the vena cava (white arrowhead). (d) In difficult circumstances, power Doppler can even more effectively display the entire renal artery
To rule out orificial stenotic disease commonly associated with plaque on the aortic wall, the Doppler sample volume is swept slowly from within the lumen of the aorta and through the renal artery ostium. In making the transition from the aorta into the renal artery, the high-resistance aortic flow pattern changes to a low-resistance configuration in the renal artery. Dependent on the angulation of the renal ostium, it may be difficult to maintain appropriate angle correction during the transition maneuver. Regardless of this technical limitation, high velocity, turbulent signals reliably indicate stenotic ostial disease.
Using the smallest sample volume, Doppler spectral waveforms are recorded continuously throughout the visualized length of the renal arteries. The highest peak systolic velocity in the vessel is documented being careful to utilize an angle of insonation less than 60° (Fig. 47.5a). If needed, the patient is moved to the right or left lateral decubitus, lateral oblique, or prone position for interrogation of the mid-to-distal renal artery and renal parenchymal vessels. Color and/or power Doppler imaging may facilitate appropriate angle correction and identification in cases of vessel tortuosity (Fig. 47.5b–d).
Evaluation of Renal Parenchymal Blood Flow
Blood flow patterns are documented throughout the distal renal artery and the interlobar and arcuate arteries of the renal medulla and cortex (Fig. 47.6). Using a 0° angle of insonation, spectral waveforms are recorded throughout the kidney, noting regions of increased signal amplitude and disordered or absent flow. The signals with the highest peak and end-diastolic velocities are documented from the vessels within the medulla and cortex of the upper-, mid-, and lower poles of the kidney. The presence of cortical thinning, cysts, masses, renal calculi, hydronephrosis, and/or perinephric fluid collections is noted (Fig. 47.7).
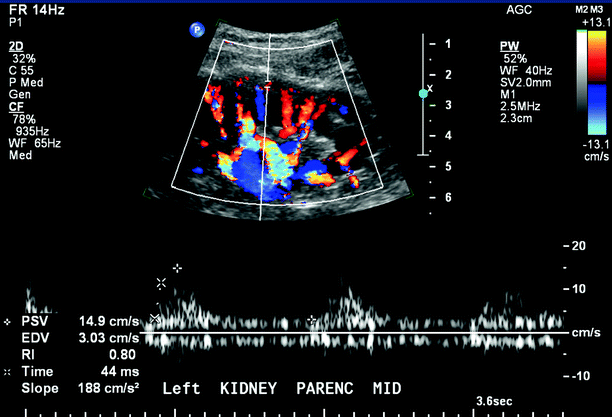
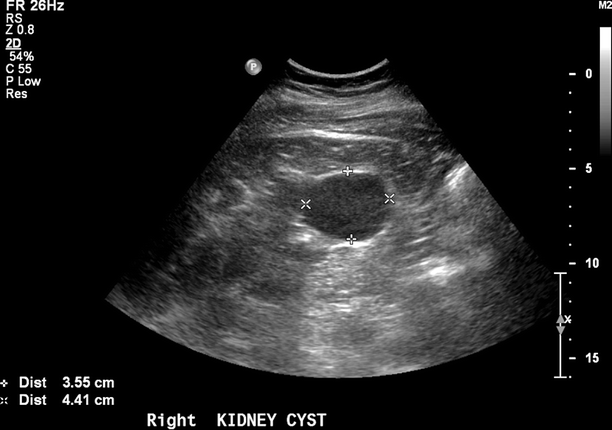
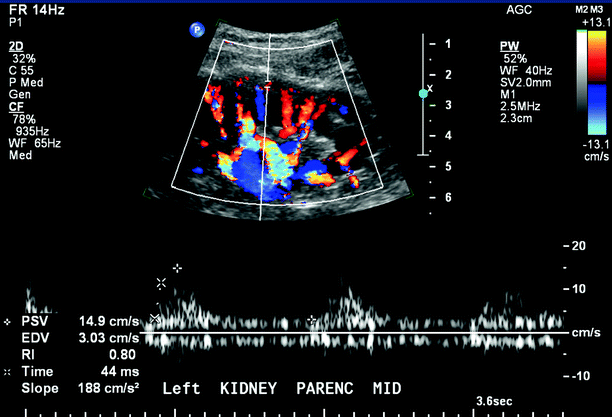
Fig. 47.6
Color flow image and Doppler spectral waveforms from the arcuate arteries within the renal cortex. Normal renal parenchymal flow is characterized by constant forward diastolic flow
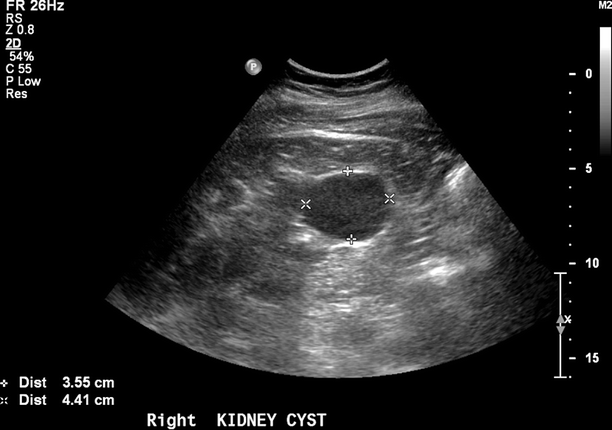
Fig. 47.7
Benign echolucent renal cysts are commonly found in the body of the kidney
Measurement of Renal Size
Because renal atrophy would be a contraindication to revascularization, the pole-to-pole length of each kidney is documented during each study (Fig. 47.8). Normal renal length is between 9 and 13 cm in the greatest longitudinal plane and there is usually less than 1 cm difference in renal lengths between the two kidneys. The length of the organ is measured during maximum inspiration using a flank approach to optimize visualization of the renal margins on both poles.
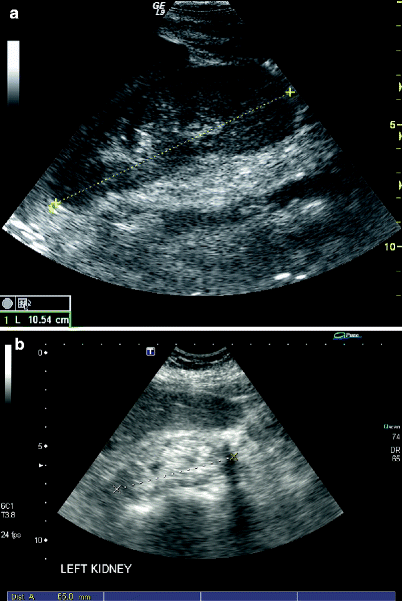
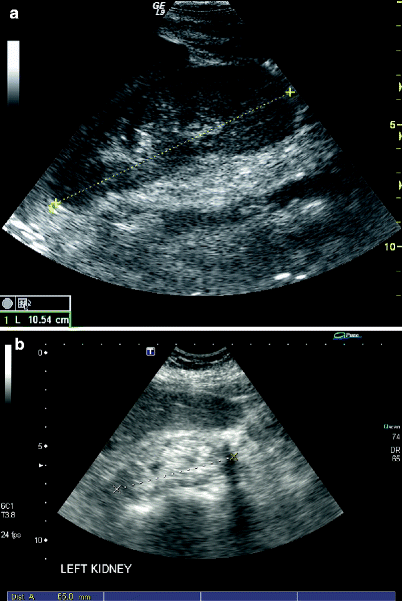
Fig. 47.8
The pole-to-pole length of the kidney is measured to evaluate suitability for revascularization of stenotic lesions. Normal adult kidney length is greater than 9 cm (a) while atrophic organs are significantly shorter and more echogenic in character (b)
Evaluation for Renal Vein Thrombosis
Although most renal studies are performed for examination of the arterial circulation, renal vein thrombosis can lead to acute renal failure and referral to the vascular laboratory. Confirmation of renal vein thrombosis can be technically challenging. The vein may be dilated with acute thrombus or contracted when it has been chronically thrombosed. Optimization of the grayscale image is needed to demonstrate intraluminal echoes that indicate thrombosis (Fig. 47.9). Doppler spectral examination will document the absence of blood flow within the vein and a retrograde, blunted diastolic flow component in the renal arterial signals (Fig. 47.10). In the case of renal cell carcinoma, extensive tumor involvement in the renal vein is common with extrusion and propagation into the IVC. In patients with a thrombosed IVC, possibly associated with prior placement of a vena caval filter, retrograde thrombosis may extend into the renal veins.
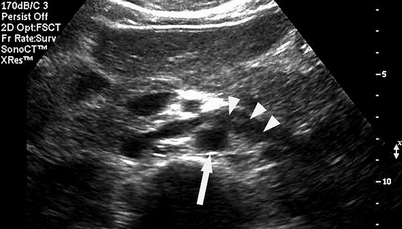
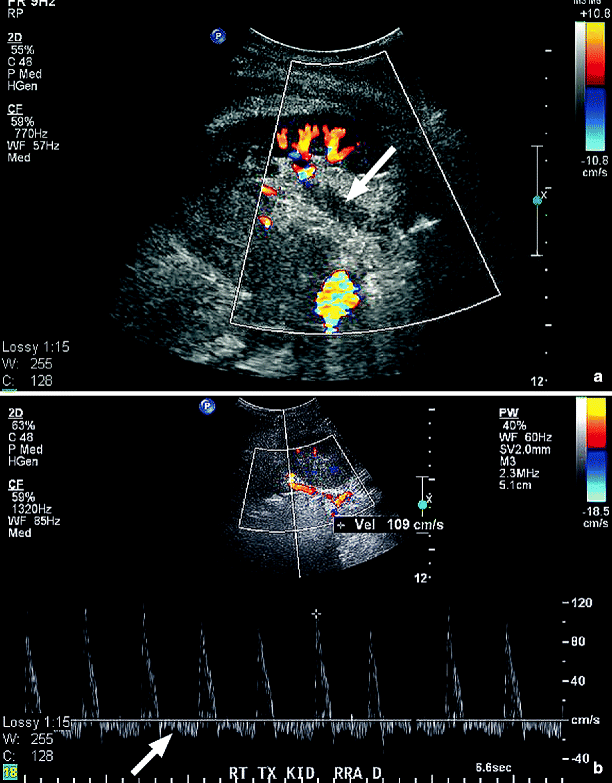
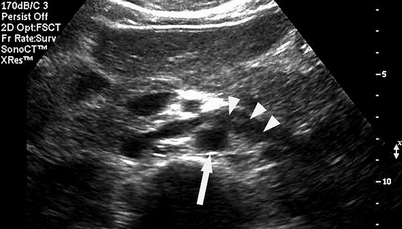
Fig. 47.9
Transverse abdominal B-Mode image of the aorta (arrow) and the crossing left renal vein demonstrating dilation of the vein and intraluminal echoes (arrowheads) consistent with thrombus
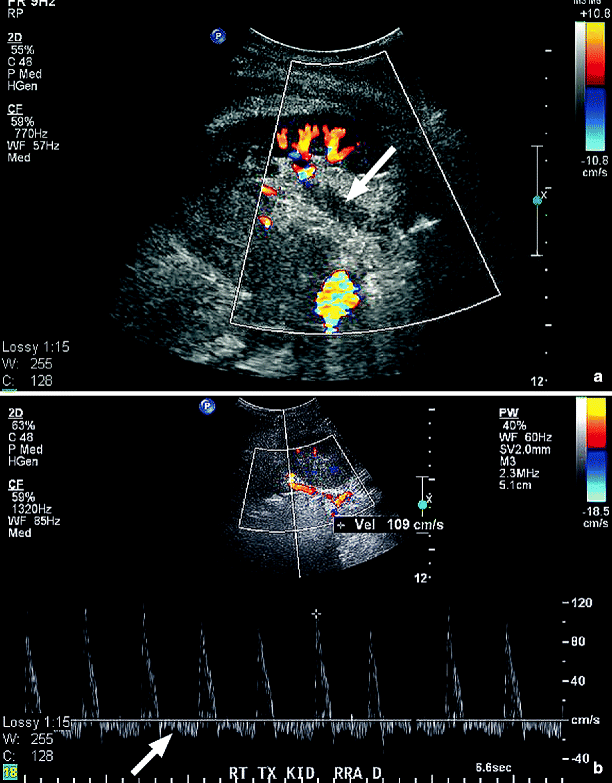
Fig. 47.10
(a) Longitudinal color duplex image of a transplanted kidney showing lack of color flow and echogenic thrombus in the dilated renal vein (arrow). (b) Doppler spectral waveforms recorded from the inflow renal artery. Note the retrograde diastolic flow (arrow) suggestive of obstruction to venous outflow from the kidney (Images courtesy of Nicos Labropoulos, Ph.D.)
Contrast Enhanced Imaging
Duplex imaging of renal artery stenosis can be technically challenging and is influenced by both operator expertise and patient factors, such as overlying bowel gas, obesity, and inability to optimally position the patient due to lack of patient cooperation [31]. Contrast enhancement to improve imaging and increase diagnostic accuracy is prevalent in radiologic techniques such as angiography, computed tomography (CT), and MRI. Ultrasound contrast agents are stabilized gas microbubbles that enhance ultrasound signals due to the difference between them and the surrounding blood in terms of compressibility and density. They reflect transmitted ultrasound waves strongly, enhancing echogenicity at both fundamental and harmonic frequencies, which provides improved vascular imaging and tissue differentiation [45, 46]. Intravenous ultrasound contrast agents have been found to improve ultrasound diagnostic accuracy and are approved for use in the heart with utility also reported in the liver, mesenteric, and peripheral vasculature [47–49] (Fig. 47.11).
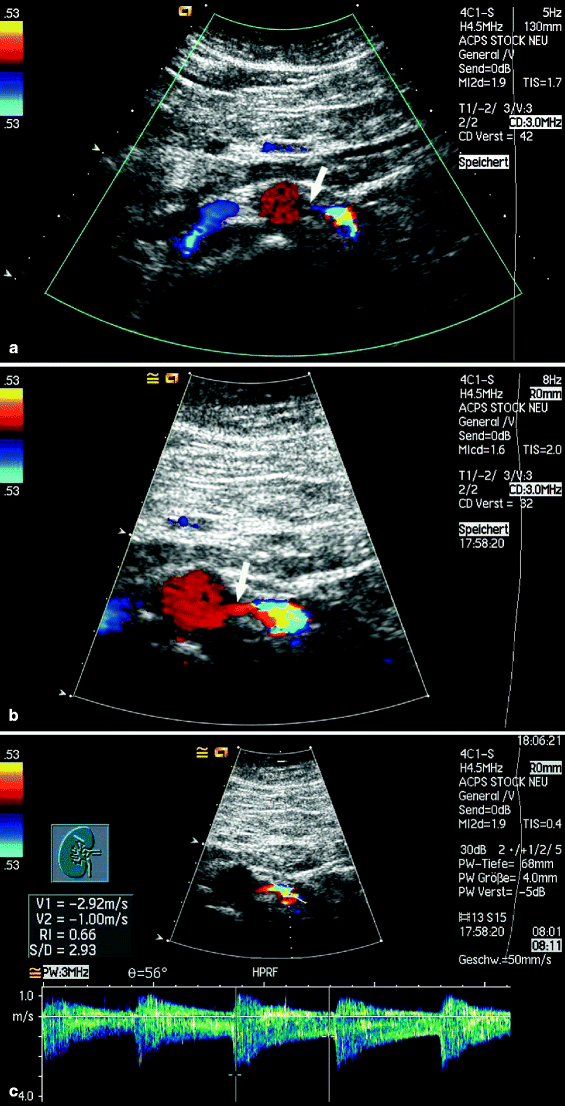
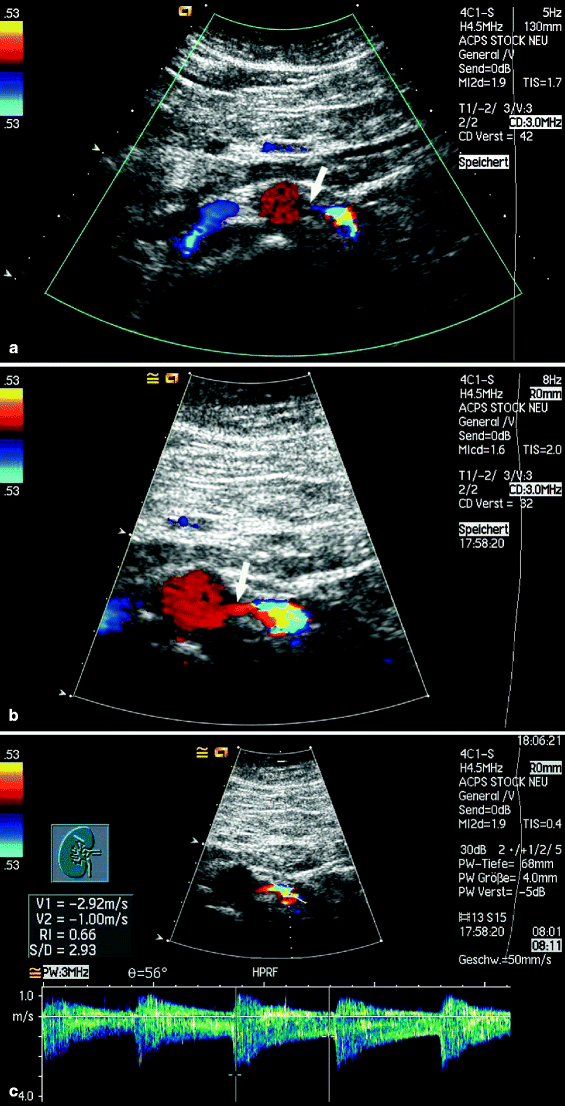
Fig. 47.11
(a) Transverse color flow image of an atherosclerotic abdominal aorta without contrast is unable to demonstrate the origin (arrow) of the left renal artery. (b) With the addition of contrast, the proximal portion of the renal artery (arrow) is well visualized. (c) This allows accurate placement of the sample volume to identify the presence of a high-grade stenosis (Images courtesy of Konrad Stock, M.D.)
A prospective study examined the use of perflutren contrast agent (Definity, DuPont) to improve imaging of the renal arteries [50]. This agent is composed of three phospholipids and a perfluoropropane gas that, when mixed together, form lipid-encapsulated-gas-filled microbubbles [48]. Because the microbubbles are less than 5 μm in diameter, and thus smaller than red blood cells, they pass easily through the pulmonary microcirculation and are rapidly cleared by respiration from the systemic circulation within 5 min of their infusion. This particular agent requires mixing for 45 s at 4,500 oscillations/min in a specific vial shaker. For this study, a volume of 1.3 ml of agitated contrast solution was injected into a 50 ml bag of 0.9% sterile saline solution, which was subsequently infused intravenously at a rate of 2 ml/min via an 18-guage needle throughout the duration of the duplex examination. The results of the ultrasound examination, with and without contrast enhancement, were compared with intra-arterial angiography. Both duplex alone and duplex with contrast demonstrated excellent identification of the renal arteries (Table 47.2). Overall visualization of the renal arteries was 85% for standard duplex and 94% following contrast. Contrast infusion was particularly helpful in identifying additional accessory arteries and visualization of hemodynamically significant stenotic vessels. A total of five of seven arteries not visualized by color flow duplex was detected following the infusion of contrast, resulting in an additional 10% (5/48) of vessels visualized. In addition, significantly longer lengths of the renal arteries were visualized in continuity when contrast was infused (3.9 cm as compared to 3.3 cm [P = 0.001]).
Table 47.2
Visualization of renal arteries with intravenous contrast
Renal arteries | Duplex alone | Duplex + contrast |
---|---|---|
All renal arteries (48) | 41 (85%) | 45 (94%) |
Main (43) | 40 (93%) | 42 (98%) |
Accessory (5) | 1 (20%) | 3 (60%) |
Normal/minimal disease (32) | 30 (94%) | 31 (97%) |
Stenosis > 60% (12) | 9 (75%) | 12 (100%) |
Occlusion (4) | 3 (75%) | 3 (75%) |
Visualized length of artery | 3.3 ± 0.3 cm | 3.9 ± 0.3 cm |
There were no complications associated with the use of the intravenous contrast. The patients experienced no changes in blood pressure or heart rates during the contrast infusion and no deterioration of renal function as measured by blood urea nitrogen or serum creatinine levels, consistent with the experience of other investigators [48, 51]. Insonated Doppler velocities, however, were increased following contrast administration by an average of 10% in normal or minimally diseased vessels and 12% in stenotic vessels (Table 47.3). Although these differences were statistically significant, they did not lead to a change in the category of stenosis. This artifactual increase in measured Doppler velocities has been noted in both human and in vitro studies [49, 52]. As contrast agents become more extensively used, separate velocity criteria may need to be established. Currently, however, the 10–12% increase in peak systolic velocities does not represent a large enough difference to require immediate attention but should be kept in mind for borderline results.
Table 47.3
Doppler velocities following contrast administration
Renal arteries | Duplex alone | Duplex + contrast |
---|---|---|
Normal/minimal disease (cm/s) | 112 ± 6 | 123 ± 7b |
Stenosis > 60% (cm/s) | 173 ± 20a | 194 ± 18a,b |
All (cm/s) | 127 ± 7a | 144 ± 8a,b |
House et al. used contrast enhancement (Levovist, Schering, Berlin, Germany) for technically unsuccessful studies or to improve diagnostic confidence in sonographic detection of recurrent stenosis in stented renal arteries [53]. Use of contrast enhancement improved the technical success rate from 89% to 95% and also increased diagnostic confidence in a significant number of examinations.
The results from these studies indicate that contrast-enhanced duplex imaging of the renal arteries is safe but not routinely required when an experienced sonographer performs the study. However, it can increase vessel visualization and thus allow more specific placement of the pulsed Doppler sample volume. This may increase overall accuracy of the study in patients with stenoses and accessory renal arteries or in approximately 10% of patients whose vessels are not initially successfully visualized. No comparative studies have been done among different contrast agents. Another perflutren-based contrast agent (Optison, Amersham Health) is FDA approved for echocardiography and has the potential benefit of not requiring a specific vial mixer before infusion. A recent controversy about a potential increased mortality risk of intravenous contrast agents in patients undergoing echocardiography has not been substantiated [54]. It is hoped that the FDA will approve noncardiac indications for ultrasound contrast agents in the near future.
Special Considerations
Accessory and Multiple Renal Arteries
Approximately 30% of the population has multiple renal arteries on one side and another 10% have bilateral multiple vessels [41, 43]. Duplicate renal arteries usually arise from the lateral aortic wall and are directed toward the central hilum of the kidney while accessory polar renal arteries course toward the upper or lower poles of the kidney. For an unknown reason, more accessory renal arteries are seen on the left as compared to the right side. When only a single accessory artery is present, it is usually supplying the lower pole of the kidney. In the presence of an accessory vessel, the main renal artery is usually of near-normal caliber while the accessory vessels have a smaller diameter. When multiple renal arteries occur, none of the vessels may be dominant and it is quite difficult to be certain that velocity measurements have been acquired from all vessels.
Positive identification of all accessory polar renal arteries by B-Mode imaging is not generally possible because of the small diameter of these vessels and lack of clinical suspicion for their presence. While color flow imaging may facilitate recognition of accessory renal arteries, it is important to remember that color encodement of Doppler-shifted frequencies from blood flow is angle dependent and accessory renal arteries may arise from the aortic wall at angles between 70° and 90° (Fig. 47.12). To overcome this problem, power Doppler imaging, which relies on the amplitude of the returned Doppler signal and is not as angle dependent as color Doppler imaging, may prove beneficial for detection of these small vessels.
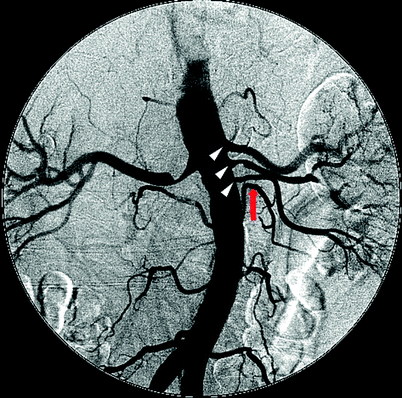
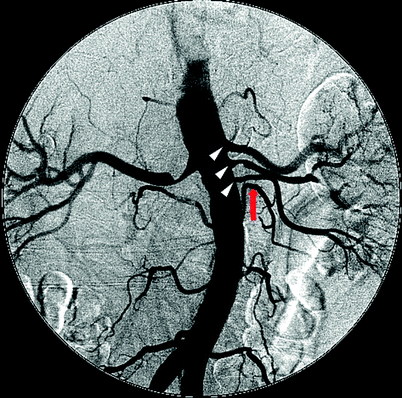
Fig. 47.12
Digital subtraction angiogram illustrating three renal arteries on the left (white arrowheads) with the mid-renal demonstrating a 50% stenosis. An adjacent lumbar artery (red arrow) can be misconstrued as another accessory artery if it is not followed distally to confirm that it does not lead to the kidney
There are several scanning maneuvers that can be used to detect the presence of supernumery renal arteries. It is helpful to increase the size of the Doppler sample volume and scan in the para-aortic region, moving from the level of the main renal artery origin to the aortic bifurcation to detect any additional low resistance signals that could imply renal arterial flow. Lumbar arteries can be confused with accessory renal arteries but these have a more posterior origin from the aorta. A sagittal image of the IVC may reveal multiple right renal arteries beneath this vessel. Using color and/or power Doppler, the origins of the renal arteries can quite often be visualized from a coronal view of the aorta (the “banana peel” approach). This is not only an excellent imaging approach in adult patients but this view also facilitates identification of fetal supernumery renal arteries during second or third trimester in utero evaluations. Accessory polar renal arteries can often be identified on a long axis or transverse color/power Doppler image of the kidney but their identity must be confirmed by tracing the vessel back to its origin. It should also be noted that the use of an intravenous contrast agent has improved the detection of accessory renal arteries [49].
Occlusion of the Main Renal Artery
Diagnosis of an occluded main renal artery can be difficult because the sonographer is appropriately concerned that it has not been possible to adequately visualize flow within the vessel rather than prematurely concluding that the vessel is thrombosed. Excessive overlying abdominal gas that may compromise visualization compounds this hesitation. After optimizing Doppler spectral and color flow parameters for detection of very slow flow, and possibly the use of intravenous contrast, it is possible to look for secondary indications of renal artery occlusion. With chronic occlusion and atrophy, kidney length is less than 8 cm and there can be a significant >3 cm difference in length as compared with the contralateral kidney. Intraparenchymal insonation demonstrates low cortical velocity of <10 cm/s with low amplitude and a delayed systolic upstroke. The presence of cortical blood flow is a result of collateral flow to the kidney through adrenal and ureteral vessels.
Horseshoe Kidney
Horseshoe kidneys are not common, occurring in less than 1% of autopsies. Most often, the organs are fused at the lower pole, with approximately 10% of kidneys joined at the upper pole. This large unified kidney, connected by an isthmus of renal parenchyma of variable size, will lie anterior to the aorta usually at the level of the fourth or fifth lumbar vertebrae. Horseshoe kidneys are usually found in patients who present for investigation of a pulsatile abdominal mass or they may be discovered incidentally during an abdominal ultrasound examination.
Anatomically, the kidney is supplied by multiple renal arteries and drained by multiple renal veins at unpredictable locations [40, 43]. Multiple arteries may arise from the iliac arteries or the distal aorta. Their location, posterior to the body of the kidney, makes it difficult to identify these arteries and even more challenging to diagnose the presence of stenoses. In patients with an underlying abdominal aortic aneurysm, diagnosing renal artery stenosis is best accomplished with contrast angiography.
Renal Aneurysms
Aneurysmal dilatation of the renal artery usually involves the main segment of the renal artery or its first-order branches [55]. Although aneurysms may also occur intraparenchymally, this location is seen in only approximately 10% of cases. Renal aneurysms are most easily identified with color flow imaging (Fig. 47.13). Their large size, compared to the native vessel, makes identification relatively easy. Intervention is generally recommended for aneurysms of 2 cm or greater in diameter. Therefore, it is important to measure the maximal diameter of the aneurysm, either in preparation for surgical repair or as a baseline for monitoring future growth. The specific location is less important although differentiation between an aneurysm involving the renal artery and an intraparenchymal position is helpful in selecting proper therapy. Rarely, there is an associated arteriovenous fistula that makes the initial diagnosis more challenging.
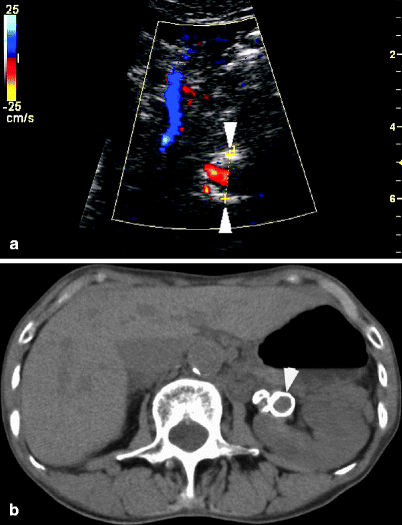
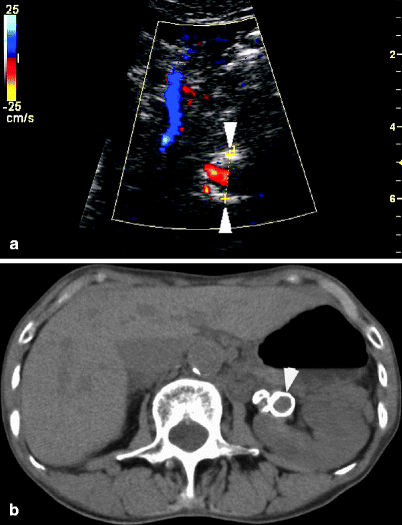
Fig. 47.13
(a) A left renal artery aneurysm is measured transversely on a sonographic image. Color flow evaluation is difficult due to circumferential calcification in the wall of the aneurysm. (b) The calcified aneurysm, located just beyond the bifurcation of the renal artery, is seen on CT scan without intravenous contrast
Fibromuscular Dysplasia
Fibromuscular dysplasia (FMD) is a term encompassing several histologic forms of arterial wall changes of which medial fibroplasia is the most common [2, 22]. As a group, FMD is the second most common cause of renal artery stenosis and accounts for up to one-third of cases of renovascular hypertension in adults. This nonatherosclerotic disease process is seen almost exclusively in females and presents as a “string of beads” angiographic appearance with web-like stenotic segments alternating with post-stenotic dilations. It involves the mid-to-distal segments of the renal artery and will frequently extend into branch vessels with characteristic superimposed high- and low-velocity signals (Fig. 47.14). Awareness that this disease affects primarily young women, in the third or fourth decade of life, an age group not usually affected by renal atherosclerosis, may aid in identification of this disorder. The less common variants of FMD, perimedial dysplasia and intimal fibroplasia, are not associated with post-stenotic dilations. Intimal fibroplasias, in particular, produce a smooth concentric stenosis. Differentiation between the types of FMD is neither practical nor necessary during sonographic examination. Because fibromuscular dysplasia is often found bilaterally, it is important to search for similar flow patterns in the contralateral renal artery.