Chapter 9
Diet and Lifestyle in CVD Prevention and Treatment
Introduction
Cardiovascular disease (CVD) is the leading cause of morbidity and mortality in developed countries and more recently in developing countries. Modifications to habitual dietary patterns and lifestyle behaviors (physical activity and tobacco use) can strongly influence the risk of developing CVD. This relationship is multifactorial, and can be influenced through effects on body weight, body composition, plasma lipid and lipoprotein concentrations, cardiorespiratory fitness, glucose homeostasis, blood pressure, and inflammatory status. The goals for any diet and lifestyle intervention with the intent of reducing CVD risk should include achieving and maintaining a healthy body weight, systolic and diastolic blood pressure values within the normal range, and plasma lipoprotein profiles and glucose concentrations associated with optimal health outcomes. This chapter will focus on diet, physical activity, and tobacco use.
Diet
There is a wide range of dietary variables that have been associated with CVD risk. Some were first identified early in the early twentieth century (e.g., dietary cholesterol), while others have been recognized more recently (e.g., trans fatty acids). Many have been the topic of controversy and as new data emerge our relative assessment of each evolves. For example, the importance of dietary cholesterol has diminished relative to the importance of saturated fat.
It is difficult, if not impossible, to assess directly the efficacy of individual dietary components on CVD risk because of the challenges, both practical and financial, in modifying the diets of a large group of people for long periods of time, as well as the difficulty that arises in studying individual dietary components within the context of habitual dietary patterns. Therefore, most dietary factors with the intent of reducing CVD risk are evaluated on the basis of short-term interventions (weeks or months) using biomarkers (e.g., plasma cholesterol concentrations, inflammatory factors) rather than hard endpoints. By combining data from different types of studies, dietary patterns have emerged that are associated with a lower risk of CVD [1, 2].
Total Dietary Fat
Dietary fat serves as a major energy source in the human diet. One gram of fat has the equivalent of 9 calories, whereas one gram of protein and carbohydrate has the equivalent of 4 calories, and alcohol has the equivalent of 7 calories per gram. The two major issues to consider with regard to total amount of dietary fat and CVD risk are body weight and plasma lipoprotein profiles. The range of total fat intake is relatively wide, from 15% of energy (%E) to 45%E. Current recommendations are to consume a diet containing 25%E to 35%E as total fat [3, 4]. For individuals with diabetes, the recommendation is to consume diets toward the higher end of this range [5, 6].
Body weight
Long-term data indicated that reducing total fat intake by 10%E resulted in only modest reductions in body weight over a 12-month period, approximately 1 kg in normal-weight individuals, and 3 kg in overweight or obese individuals [7–9]. More recent data indicate that total fat intake is not significantly related to annual body weight changes [8, 10] and only weakly related to annual changes in waist circumference [8]. One mitigating factor may be the fiber content of the diet [7–9]. Higher fiber intakes have been associated with lower body weights and waist circumferences [8]. These data suggest that consuming fruits, vegetables, and whole grains rather than fat-free cookies, cakes, and fat-free savory snacks may be preferential in preventing weight gain or promoting weight loss.
Lipoprotein Profiles
Low-fat diets are associated with elevated triglyceride concentrations and depressed high-density lipoprotein (HDL)-cholesterol concentrations resulting from what is commonly referred to as carbohydrate-induced hypertriglyceridemia [11–13]. Carbohydrate-induced hypertriglyceridemia, resulting in elevated triglyceride concentrations, is caused by an enhanced rate of hepatic fatty acid synthesis and is precipitated by an excess flow of glucose from the gut to the liver [14, 15] and subsequent production of hepatic triglyceride-rich particles, termed very low-density lipoprotein (VLDL) [16–18] (Figure 9.1). In some cases delayed triglyceride clearance associated with low-fat diets has also been observed, contributing to the elevated triglyceride concentrations [19].
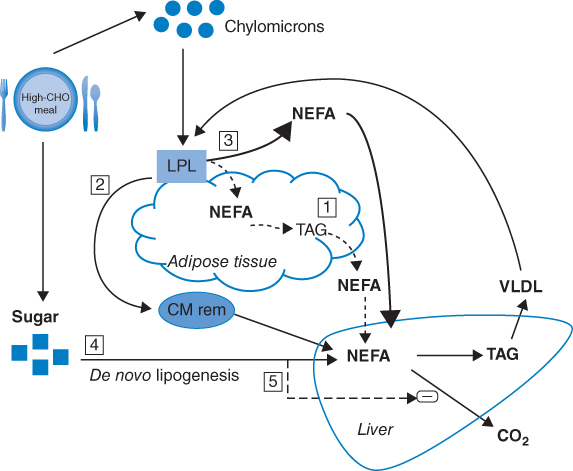
Figure 9.1 Mechanisms of carbohydrate (CHO)-induced hypertriacylglycerolemia. (1) Impaired ability of insulin to suppress lipolysis, leading to increase NEFA flux (); (2) accumulation of chylomicron remnants (CM rem;
); (3) down-regulation of adipose tissue lipoprotein lipase (LPL) activity (
; (4) de novo lipogenesis (
); (5) less hepatic fatty acid oxidation, possibly inhibited through malonyl-CoA produced in de novo lipogenesis (
). (Source: Chong et al. 2007 [17]. Reproduced with permission of Cambridge University Press.)
The metabolic response to a low-fat diet can vary depending on the experimental design or characteristics of the study subjects. Within the context of a stable body weight, replacement of dietary fat with carbohydrate results in higher triglyceride and VLDL-cholesterol concentrations, lower HDL-cholesterol concentrations, and a higher (less favorable) total cholesterol to HDL-cholesterol ratio [20–25]. This effect is blunted when an individual is in negative energy balance, engages in regular physical activity, or consumes a fiber-rich source of carbohydrate [11, 23, 24]. Sedentary individuals with visceral adiposity are at particularly high risk for carbohydrate-induced hypertrygliceridemia [13]. The associated elevated insulin concentrations (insulin resistance) may enhance VLDL synthesis or delay clearance.
The extent to which the fat to carbohydrate ratio of the diet is altered predicts the change in triglyceride and HDL-cholesterol concentrations [26, 27] or CVD outcomes [28]. Moderate carbohydrate restriction and weight loss have been reported to provide equivalent but nonadditive improvements in the atherogenic dyslipidemic pattern, characterized by an elevated triglyceride concentration and total cholesterol to HDL-cholesterol ratio [29].
Type of Dietary Fat
Studies performed in the mid-1960s demonstrated that changes in dietary fatty acid profiles altered plasma total cholesterol concentrations in most individuals [30, 31]. In using a variety of different experimental designs, more recent work has confirmed these early findings [25, 32–34]. When carbohydrate is displaced by saturated fatty acids, low-density lipoprotein (LDL)-cholesterol concentrations increase, whereas when carbohydrate is displaced by unsaturated fatty acids, LDL-cholesterol concentrations decrease. The effect of polyunsaturated fatty acids is greater than that of monounsaturated fatty acids [25, 35]. When carbohydrate is displaced by saturated, monounsaturated, or polyunsaturated fatty acids, HDL-cholesterol concentrations are increased, with saturated fatty acids having the greatest effect and polyunsaturated fatty acids having the least effect. With respect to dietary fat and total cholesterol to HDL-cholesterol ratios, there is little effect when carbohydrate is displaced by saturated fatty acids, whereas monounsaturated and polyunsaturated fatty acids decrease the ratio (more favorable) to a similar magnitude. Relative to carbohydrate, all types of dietary fat decrease triglyceride concentrations. Changes in LDL-cholesterol concentrations induced by changes in the fatty acid profile of the diet are primarily attributed to differences in the fractional catabolic rate of LDL rather than production rate [36, 37]. Differences in HDL-cholesterol concentrations induced by changes in the fatty acid profile of the diet are primarily attributed to differences in the production rate rather than the fractional catabolic rate [38, 39].
The major sources of saturated fatty acids in the diet are meat and dairy fats. The major sources of monounsaturated fatty acids in the diet are canola and olive oils. The major sources of polyunsaturated fatty acids (PUFA) in the diet are soybean, corn, safflower, and sunflower oils. The common dietary fatty acids are listed in Table 9.1.
Table 9.1 Common dietary fatty acids.
Code | Common Name | Formula |
SATURATED | ||
12:0 | lauric acid | CH3(CH2)10COOH |
14:0 | myristic acid | CH3(CH2)12COOH |
16:0 | palmitic acid | CH3(CH2)14COOH |
18:0 | stearic acid | CH3(CH2)16COOH |
MONOUNSATURATED | ||
16:1n-7 cis | palmitoleic acidCH3 | (CH2)5CH=(c)CH(CH2)7COOH |
18:1n-9 cis | oleic acid | CH3(CH2)7CH=(c)CH(CH2)7COOH |
18:1n-9 trans | elaidic acid | CH3(CH2)7CH=(t)CH(CH2)7COOH |
POLYUNSATURATED | ||
18:2n-6,9 all cis | linoleic acid | CH3(CH2)4CH=(c)CHCH2CH=(c)CH(CH2)7COOH |
18:3n-3,6,9 all cis | α-linolenic acid | CH3CH2CH=(c)CHCH2CH=(c)CHCH2CH=(c)CH(CH2)7COOH |
18:3n-6,9,12 all cis | γ-linolenic acid | CH3(CH2)4CH=(c)CHCH2CH=(c)CHCH2CH=(c)CH(CH2)4COOH |
20:4n-6,9,12,15 all cis | arachidonic acid | CH3(CH2)4CH=(c)CHCH2CH=(c)CHCH2CH=(c)CHCH2CH=(c)CH(CH2)3COOH |
20:5n-3,6,9,12,15 all cis | eicosapentaenoic acid | CH3(CH2CH=(c)CH)5(CH2)3COOH |
22:6n-3,6,9,12,15,18 all cis | docosahexaenoic acid | CH3(CH2CH=(c)CH)6(CH2)2COOH |
Unique Fatty Acid Classes
Omega-3 (n-3) PUFA
A difference in the prevalence of CVD between Greenland Inuits and genetically similar Scandinavians was first reported in the early 1980s [40, 41]. The lower rate of CVD in the Greenland Inuits was attributed to higher HDL-cholesterol concentrations, resulting from higher intakes of marine foods, particularly the n-3 PUFA.
Since that time, a number of studies have reported an inverse association between dietary n-3 PUFA and CVD and stroke risk [42]. Intervention studies have demonstrated that eicosapentaenoic acid (EPA, 20:5n-3) and docosahexaenoic acid (DHA,22:6n-3), but not alpha-linolenic acid (ALA, 18:3n-3), positively affect CVD outcomes in both primary and secondary prevention settings [43]. The effect of EPA and DHA on CVD has been attributed to antiarrhythmic properties and effects on lowering plasma triglyceride concentrations, platelet reactivity, and blood pressure [44–46]. A meta-analysis suggested that for beneficial effects on CVD outcomes to be achieved, the minimum intake should be 250 mg EPA+DHA per day [47]. This intake can be achieved by adhering to the recommendation to consume at least two fish meals per week, particularly oily fish for the general population [3, 4, 48].
The three major dietary n-3 PUFA are ALA, EPA, and DHA. ALA is found in plants and plant oils. Of oils commonly used in food preparation, soybean oil and canola oil have the highest levels of ALA. EPA and DHA, sometimes referred to as very long chain n-3 PUFA, are found in marine foods, predominantly fatty fish. Humans can convert ALA to EPA and DHA. However, this conversion capacity is low [49–51]. For this reason, the recommendation, with respect to CVD risk reduction, is made on the basis of fish meals per week [3, 4, 48]. For individuals with established disease, the current recommendation is to consume the equivalent of 1 g EPA+DHA per day. Due to their hypotriglyceridemic effect, 3–5 g EPA+DHA is recommended for individuals with chronically elevated triglyceride concentrations and use should be monitored by a physician [48, 52]. A new source of DHA, algal oil, has also been shown to decrease triglyceride concentrations significantly [53].
Trans Fatty Acids
The double bonds in fatty acids occur in the cis or trans configuration. The cis configuration is the predominant form, representing the majority of double bonds in plant oils and animal fats.
Since the 1990s attention has focused on the effect of trans fatty acids on CVD and other health outcomes [54, 55]. Similar to saturated fatty acids, trans fatty acids increase LDL-cholesterol concentrations. In contrast to saturated fatty acids, trans fatty acids do not increase HDL-cholesterol concentrations. Taken together, these changes result in a less favorable LDL-cholesterol/HDL cholesterol ratio with respect to CVD risk [25, 56]. A trend toward increased triglyceride concentrations is frequently reported. Some research has also suggested that trans fatty acids may increase lipoprotein (a) and high-sensitivity C-reactive protein concentrations, insulin resistance, metabolic syndrome, and diabetes [57, 58].
There are two main sources of dietary trans fatty acids: those that occur naturally in meat and dairy products as a result of anerobic bacteria fermentation in ruminant animals, and those formed during partial hydrogenation of vegetable or fish oils. Traditionally, oils have been partially hydrogenated to increase their viscosity (changing liquid oil into a semi-liquid or solid) and extend their shelf life (decreasing susceptibility to oxidation). In recent decades the major source of dietary trans fatty acids has been from partially hydrogenated fat, primarily from commercially prepared fried foods and baked goods [57].
The trans fat intake has declined in recent years in the USA. In 2003 the Food and Drug Administration mandated that by 2006 the trans fat content of packaged food should be listed on the Nutrient Facts panel [59]. As a result, between 2005 and 2010 the US Department of Agriculture documented a decline in the trans fat content of newly introduced foods and a rise in the use of “no trans fat” claims on food packages [60]. Around that same time some US cities restricted the use of partially hydrogenated fats by chain restaurants. In one such city, New York, between 2006 and 2008 the proportion of restaurants using partially hydrogenated fat declined from 51% to 2% [61]. Between the years 2000 and 2009, plasma trans fatty acid levels in non-Hispanic white adults living in the USA decreased by 50% [62]. Similar trends have been seen in other counties [63, 64].
Dietary Cholesterol
The observation that dietary cholesterol increased blood cholesterol concentrations and is associated with the development of CVD was first made early in the twentieth century in rabbits [65]. In humans, a positive association between dietary cholesterol and both plasma cholesterol concentrations and CVD risk has been repeatedly observed [66–68]. However, within the range currently consumed in the USA, 250–350 mg per day, the impact of further decreasing dietary cholesterol on plasma cholesterol concentrations, in most people, is modest [69].
The effect of dietary cholesterol on plasma lipoprotein concentrations is less than that of saturated and trans fatty acids, and as such receives less emphasis with respect to dietary recommendations [3, 70]. Of note is that there is a relatively high degree of variability in response to dietary cholesterol among individuals, and this hypo- and hyper-responsiveness has been attributed to genotype differences [71].
With few exceptions, dietary cholesterol is present in foods of animal origin. Eggs provide a particularly rich source of dietary cholesterol. Other sources include milk and meat fat. With regard to the latter source, restricting saturated fat intake is likely to result in a decrease in dietary cholesterol intake.
Dietary Carbohydrate
The area of dietary carbohydrate and CVD risk is less defined than dietary fat and CVD risk, notwithstanding the relationship, as it pertains to low-fat/high-carbohydrate diets and both body weight and lipoprotein profiles.
A positive association has been reported between diet patterns high in simple carbohydrate (primarily sucrose and high-fructose corn syrup) and CVD outcomes, and a negative association between diet patterns high in unrefined carbohydrate (whole grains) and CVD risk factors [72–84]. The intervention data have been heterogeneous and limited in scope [79–82, 85–88].
A negative association has been reported between diets high in unrefined carbohydrate and diabetes risk [76, 87, 89–91]. Studies directly comparing the effect of refined carbohydrate (products made with white flour) and simple carbohydrate on plasma glucose and insulin concentrations have yielded mixed results [92–94]. Likewise, study results directly comparing the effect of unrefined carbohydrate and refined carbohydrate on plasma glucose and insulin concentrations have been mixed [95–97].
This discordance between the observational and interventional data is likely attributable to experimental difficulties in distinguishing among the differences associated with substituting foods high in simple carbohydrate and whole grains in the diet. However, on the basis of the totality of the data, dietary recommendations consistent with emphasizing the intake of products made with whole grains is prudent and consistent with overall dietary pattern guidance to minimize CVD risk.
Fiber
An inverse association between fiber and CVD risk has been reported for whole-grain intake and CVD [98]. Studies that have estimated the independent effect of dietary fiber on CVD risk have ranged from concluding a modest [99–103] to a more substantial (20% to 40%) [98] effect for those who eat whole grain foods regularly relative to those who eat them rarely. To avoid increased energy intake, recommendations to increase dietary fiber should be focused on substituting foods made with refined carbohydrate with those made with whole grains rather than adding whole-grain foods to the diet.
Most evidence suggests that soluble fiber exerts its hypocholesterolemic effect by binding bile acids and cholesterol in the intestine, resulting in increased fecal loss and altered colonic metabolism of bile acids [104]. The fermentation of fiber polysaccharides in the colon yields short-chain fatty acids. Some evidence suggests that these compounds may have hypocholesterolemic effects via alterations in hepatic metabolism. Interestingly, observational data has consistently associated dietary insoluble fiber from cereals, but not vegetables and fruits, with lower CVD risk and slower progression of atherosclerotic lesions [105–107]. Current recommendations for adults are the consumption of 25–28 g dietary fiber daily.
Fructose
In adults, higher intakes of sugar-sweetened beverages, frequently made from high-fructose corn syrup, have been associated with weight gain over time and increased risk for developing type 2 diabetes. In children, higher intakes of sugar-sweetened beverages have been associated with higher body weights [108–110]. Due to these relationships, concern was raised about the potential relationship between fructose and high-fructose corn syrup and both body weight and CVD risk factors [111].
Intervention data have suggested that at least in the short term, an increase in fructose intake, either alone or as high-fructose corn syrup, relative to glucose or sucrose, resulted in similar effects on insulin sensitivity or secretion, glucose kinetics, lipolysis, and glucose, insulin, C-peptide, triglycerides, HDL-cholesterol, and LDL-cholesterol concentrations [112–114]. Recent attention has now shifted from the type of carbohydrate per se to the form carbohydrate – liquid (beverage) verses solid (food) [115, 116]. This is an active area of investigation at this time.
Protein
Type of Protein
Target dietary recommendations for protein as a percentage of energy have changed little over the years [3, 70, 117–120]. Dietary protein can be divided into two categories as defined by origin: animal, primarily meat, fish and dairy; and vegetable, primarily grains and legumes. For the most part, the former source of protein contributes the majority of dietary saturated fatty acids and much of the monounsaturated fatty acids to the diet, while the latter, with the exception of tropical oils (palm, palm kernel, and coconut), contributes the majority of the polyunsaturated and some of the monounsaturated fatty acids to the diet. Fish contributes the majority of the very long-chain n-3 fatty acids to the diet, whereas soybean and canola oils contribute the majority of the ALA to the diet. The implications of fatty acids accompanying the sources of protein has already been discussed.
Soy Protein
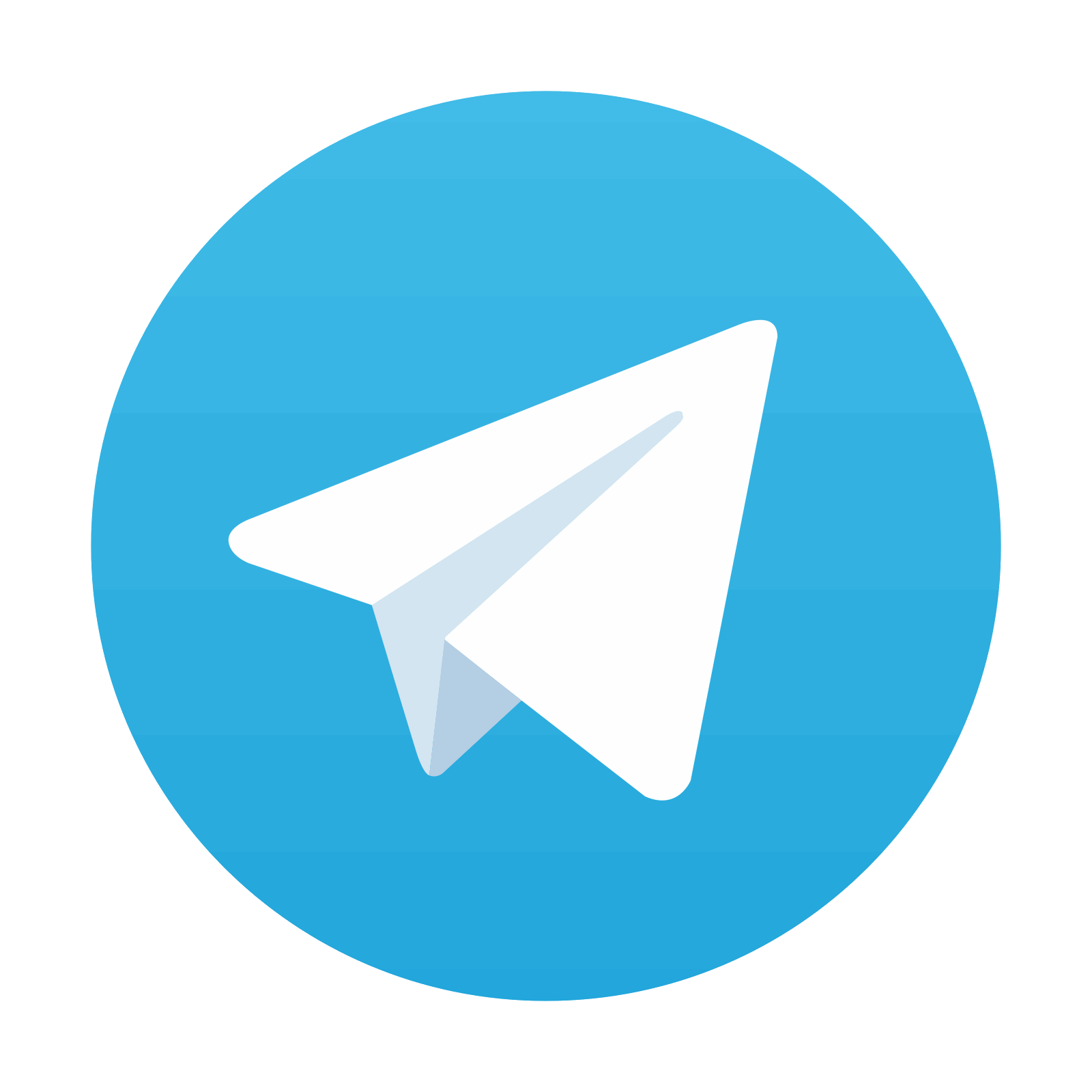
Stay updated, free articles. Join our Telegram channel

Full access? Get Clinical Tree
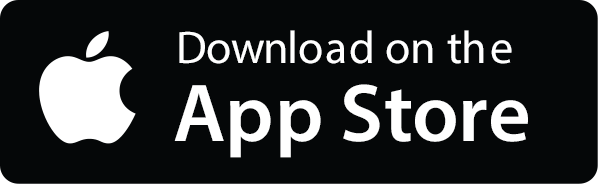
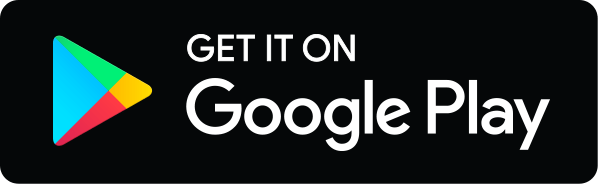