Diagnostic Studies in Lung Cancer
Lucian R. Chirieac
Massimo Loda
Introduction
Lung cancer is the leading cause of cancer deaths in the United States in both sexes, with an estimated mortality of more than 200,000 in 2007.61,116 Unfortunately the 5-year overall survival rate is only 15%, despite current therapy.61 The standard therapy includes surgical resection, platinum-based chemotherapy, and radiation therapy alone or in combination. This chapter provides an introduction to the role of the pathologist in thoracic surgery, as part of the management of patients with lung cancer, from (a) preoperative diagnosis and staging, to (b) intraoperative evaluation of the extent of distant disease and margin status, to (c) postoperative assessment of tumor genetic alterations. Last, we describe (d) the basic molecular biology principles and selected molecular diagnostic techniques and demonstrate their application in lung cancer.
Pathologists experienced in thoracic oncology are essential members of the thoracic team. The goals of pathologic analysis of surgical lung specimens are to classify the lung cancer, determine the extent of its invasion (pleural, lymphovascular, soft tissue, chest wall), establish the status of the surgical margins for cancer involvement, and determine the molecular abnormalities of lung cancer that may be able to predict sensitivity and resistance to various chemotherapeutic agents and epidermal growth factor receptor tyrosine-kinase inhibitors (EGFR-TKIs).22,30,37 Accurate disease diagnosis, staging, and identification of molecular abnormalities in lung cancer is extremely important to the decision-making process and influences the course of treatment, selection of the optimal surgical approach, and pursuit of appropriate adjuvant and neoadjuvant therapies such as chemotherapy, radiation, and other innovative approaches to treatment.
After the diagnosis of malignancy has been confirmed, the pathologist must determine whether the tumor is primary to lung or a metastasis from distant sites. Most tumors found in the lung represent metastatic foci from distant primaries, such as breast and colon cancer, as opposed to a primary lung malignancy.86 While the pathologic features of metastatic and primary lung adenocarcinoma may be similar, the treatment approaches are different. Immunohistochemistry has proved to be an important diagnostic tool helping to make the distinction in these cases. Primary malignant tumors of the lung are most often of epithelial origin. The epithelial tumors are broadly divided into small-cell lung cancer (SCLC) and non-small-cell lung cancer (NSCLC). NSCLC is further classified as squamous cell carcinoma, adenocarcinoma, and large-cell carcinoma.146
The pathologist plays a fundamental role in the preoperative, intraoperative, and postoperative evaluation. The preoperative evaluation includes examination of bronchial brushings, bronchial washings, fine-needle aspiration (FNA) biopsy, core needle biopsy, endobronchial biopsy, and transbronchial biopsy. Because lung cancer demonstrates a great deal of heterogeneity, accurate classification is dependent on specimen size and sampling technique: if the pathology sample is limited, sometimes the only categorization that can be made is the distinction between NSCLC and SCLC. Lymph node status is among the most important prognostic features in patients with NSCLC.34,108 Since mediastinoscopy with pathologic examination of lymph nodes remains the “gold standard” for the evaluation of lymph node status in patients with NSCLC, mediastinal lymph nodes are sampled during the preoperative evaluation and provide information important to staging and therapeutic options.83,96,121 The intraoperative evaluation of the surgical pathology specimen is performed by frozen section examination, which can be analyzed immediately and findings communicated to the operating room. Lobectomy or pneumonectomy specimens are routinely evaluated intraoperatively to determine the status of the surgical resection margin, diagnose incidental nodules discovered at the time of surgery, and evaluate regional lymph nodes.
The postoperative evaluation reveals pathologic characteristics necessary for the classification of tumor type, staging, and prognostic factors. In addition, molecular diagnosis in lung cancer may be able to identify genetic alterations capable of finding therapeutic modalities to target these alterations. The parameters considered in the surgical pathology report are histologic type, histopathologic grade, visceral pleural invasion (Fig. 16-1), venous/lymphatic vessel invasion (Fig. 16-2), bronchial invasion, tumor-nodes-metastasis (TNM) status, and stage grouping.41
In 2004, a revised World Health Organization (WHO) classification was created after a consensus meeting in Lyon, France (Table 16-1).146 This tumor classification system provides the foundation for tumor diagnosis, patient therapy, and a critical basis for epidemiologic and clinical studies.10 Extensive sampling is necessary to correctly identify each of these elements of the surgical pathology specimen.
Bronchioloalveolar Carcinoma
Bronchioloalveolar carcinoma (BAC) is an important subtype of pulmonary adenocarcinoma. This subtype of lung cancer has received increasing attention in recent years owing to its
increasing incidence and rate of sensitivity to epidermal growth factor–tyrosine kinase inhibitors (EGFR-TKIs).59 The definition of BAC was changed in 1999 by the WHO, which restricted the BAC to noninvasive tumors only, where the neoplastic cells spread out along preexisting alveolar structures (lepidic spread).146 Therefore BAC requires absence of invasion of stroma, pleura, or lymphatic spaces. Tumors that include some but not all of these features are classified as adenocarcinoma of mixed subtype with a predominant BAC pattern.144
increasing incidence and rate of sensitivity to epidermal growth factor–tyrosine kinase inhibitors (EGFR-TKIs).59 The definition of BAC was changed in 1999 by the WHO, which restricted the BAC to noninvasive tumors only, where the neoplastic cells spread out along preexisting alveolar structures (lepidic spread).146 Therefore BAC requires absence of invasion of stroma, pleura, or lymphatic spaces. Tumors that include some but not all of these features are classified as adenocarcinoma of mixed subtype with a predominant BAC pattern.144
![]() Figure 16-2. Lymphovascular invasion. A focus of squamous cell carcinoma is present in a lymphatic space (× 400). |
BAC is divided into three subtypes: mucinous (Fig. 16-3), nonmucinous (Fig. 16-4), and a mixed mucinous and nonmucinous or indeterminate form.59 As with conventional lung adenocarcinoma, nonmucinous BAC expresses the thyroid transcription factor-1 (TTF-1). Mucinous BACs, however, may have an aberrant immunophenotype: they express CK20 but reportedly lack TTF-1 and CK7 expression.43
Both subtypes of BAC should be distinguished morphologically from primary adenocarcinoma of the conventional type, metastatic adenocarcinoma, and other benign and/or reactive conditions. Like pulmonary adenocarcinoma of the conventional type, BACs are usually CK7+ and CK 20– and therefore distinguishable from CK7– and CK20+ metastatic adenocarcinoma of the colorectum. However, mucinous BAC is often CK7–/CK20+.123 CDX-2, which is a highly sensitive and specific marker of adenocarcinomas of intestinal origin, helps to distinguish mucinous BAC from metastatic primary gastrointestinal cancers.76
Tumors with Neuroendocrine Morphology
Neuroendocrine (NE) tumors of the lung are a distinctive subset of lung cancers characterized by varying degrees of
neuroendocrine morphologic, immunohistochemical, and ultrastructural features.10 This category includes a wide spectrum of tumor types from the low-grade typical carcinoid tumors (TCs) (Fig. 16-5), to the intermediate-grade atypical carcinoid tumors (ACs) (Fig. 16-6), to the two high-grade tumors, large-cell neuroendocrine carcinoma (LCNEC) (Fig. 16-7) and small-cell carcinoma (SCLC) (Fig. 16-8).5 Accurate classification of neuroendocrine tumors has prognostic importance. The grade of malignancy of NE tumors progresses in the following order: TC, AC, LCNEC, and SCLC.5 No prognostic difference was noted between LCNEC and SCLC. The carcinoid nomenclature is preferred by the WHO over terms such as well-differentiated neuroendocrine carcinoma,
as it provides continuity with established terminology familiar to clinicians.146 In the 2004 WHO classification, TC and AC are categorized together under the heading of carcinoid tumors; LCNEC is listed as a subtype of LCC, and SCLC is retained as an independent category. Histologically, the neuroendocrine features consist of an organoid or trabecular growth pattern, peripheral palisading of tumor cells around the periphery of tumor nests, and the formation of rosette structures.
neuroendocrine morphologic, immunohistochemical, and ultrastructural features.10 This category includes a wide spectrum of tumor types from the low-grade typical carcinoid tumors (TCs) (Fig. 16-5), to the intermediate-grade atypical carcinoid tumors (ACs) (Fig. 16-6), to the two high-grade tumors, large-cell neuroendocrine carcinoma (LCNEC) (Fig. 16-7) and small-cell carcinoma (SCLC) (Fig. 16-8).5 Accurate classification of neuroendocrine tumors has prognostic importance. The grade of malignancy of NE tumors progresses in the following order: TC, AC, LCNEC, and SCLC.5 No prognostic difference was noted between LCNEC and SCLC. The carcinoid nomenclature is preferred by the WHO over terms such as well-differentiated neuroendocrine carcinoma,
as it provides continuity with established terminology familiar to clinicians.146 In the 2004 WHO classification, TC and AC are categorized together under the heading of carcinoid tumors; LCNEC is listed as a subtype of LCC, and SCLC is retained as an independent category. Histologically, the neuroendocrine features consist of an organoid or trabecular growth pattern, peripheral palisading of tumor cells around the periphery of tumor nests, and the formation of rosette structures.
Table 16-1 World Health Organization Classification of Lung Neoplasms | |||||||||||||||||||||||||||||||||||||||||||||||||||||||||||||
---|---|---|---|---|---|---|---|---|---|---|---|---|---|---|---|---|---|---|---|---|---|---|---|---|---|---|---|---|---|---|---|---|---|---|---|---|---|---|---|---|---|---|---|---|---|---|---|---|---|---|---|---|---|---|---|---|---|---|---|---|---|
|
![]() Figure 16-5. Typical carcinoid tumor (× 400). Cells are arranged in cords and ribbons, forming a filigree pattern. No mitoses or necrosis is present. |
TC is defined as a neuroendocrine tumor with fewer than two mitoses per 2 mm2 and no necrosis (Fig. 16-5). AC is defined as a neuroendocrine tumor that meets one of the following two criteria: 2 to 10 mitoses per 2 mm2 or necrosis (Fig. 16-6). In contrast to the high-grade neuroendocrine tumors, TCs and ACs do not occur in combination with other types of carcinoma. The number of mitoses and necrosis may be present only focally within a given tumor. Therefore accurate classification of carcinoid tumors into TC or AC may not be possible in limited biopsy specimens with scant diagnostic material; a definite diagnosis may require larger fragments of tumor. In these situations, it is recommended that small biopsies be reported as “carcinoid tumor” and the appropriate classification be performed upon thorough examination of the resected specimens.
![]() Figure 16-6. Photomicrograph from a lobectomy specimen showing an atypical carcinoid tumor. Characteristic coagulative necrosis is present in the center. |
LCNEC is defined as a neuroendocrine tumor with >10 mitoses per 2 mm2 and cytologic features of large-cell carcinoma (LCC) (Fig. 16-7). These features include cells of polygonal shape with abundant cytoplasm and prominent nucleoli. Evidence of neuroendocrine differentiation must be demonstrated by performing immunohistochemistry for the specific neuroendocrine markers chromogranin and synaptophysin.145 Only tumors that show both neuroendocrine morphology and positive staining should be classified as LCNEC. It is important to note that up to 20% of conventional adenocarcinomas, SCC or LCC, will stain with neuroendocrine markers. Such tumors have
been designated as NSCLC with neuroendocrine differentiation (NSCLC-ND).
been designated as NSCLC with neuroendocrine differentiation (NSCLC-ND).
![]() Figure 16-8. This small-cell carcinoma is a neuroendocrine tumor with round to oval cells, hyperchromatic nuclei, and scout cytoplasm (× 400). |
SCLC is defined as a neuroendocrine tumor with >10 mitoses per 2 mm2 and small-cell cytologic features (Fig. 16-8). Cells are oval or vaguely spindle-shaped and have scant cytoplasm. Nuclei are hyperchromatic and have absent or very small nucleoli (Fig. 16-8). Crush artifact may be prominent on small biopsies, but this is not pathognomonic for the diagnosis of SCLC. In larger core biopsies or resected specimens, the cells may appear slightly larger than in a transbronchial biopsy and may have distinct cytoplasm. Numerous prominent nucleoli and large cells should not be seen.
LCNEC and SCLC may occur in combination with other NSCLCs as well as with each other. Such tumors are termed combined LCNEC, combined SCLC, and combined SCLC/LCNEC, respectively.92 While the two high-grade neuroendocrine tumors show numerous similarities, they are retained in separate classifications, as LCNEC currently has not been shown to respond to chemotherapy in the same fashion as SCLC. Surgery is the currently preferred treatment for LCNEC, although further studies of this relatively newly defined tumor are ongoing.58,141,159
Immunohistochemical Staining
Immunohistochemistry is routinely used in the pathology laboratory for the diagnosis of lung tumors. Immunostains are used (a) to differentiate primary pulmonary adenocarcinoma from metastatic adenocarcinoma to the lung, (b) to distinguish adenocarcinoma from malignant mesothelioma, and (c) to determine the neuroendocrine status of tumors.
Differentiation Between Primary Pulmonary Adenocarcinoma and Metastatic Adenocarcinoma
The morphologic features of primary adenocarcinoma of the lung may be similar to the features of an adenocarcinoma that is metastatic from distant primary sites.
Although the presence of multiple nodules often leads to the presumptive diagnosis of metastases, multifocal adenocarcinoma is not rare and must be distinguished from metastases. Furthermore, patients with a solitary pulmonary nodule may have metastatic adenocarcinoma to the lung as the first presentation of disease.
TTF-1 is a homeodomain-containing transcription factor that regulates tissue-specific expression of surfactant apoprotein A (SPA), surfactant apoprotein B (SPB), surfactant apoprotein C (SPC), Clara cell antigen, and T1α. TTF-1 is very important in distinguishing primary from metastatic adenocarcinoma, since the majority of the cases of primary carcinomas are positive, whereas metastatic adenocarcinoma to lung is virtually always TTF-1–negative (Fig. 16-9A). Lung cancer subtypes have different TTF-1 expression: 75% positive in adenocarcinoma, 85% positive in small-cell carcinoma, and rarely positive in squamous cell and large-cell carcinoma.
Pulmonary adenocarcinoma of the lung is usually CK7+ and CK20– and therefore distinguishable from CK7– and CK20+ metastatic adenocarcinoma of the colorectum. CDX-2 is a highly specific and sensitive marker for metastatic gastrointestinal malignancies that could be used to differentiate these entities from primary lung tumors (Fig. 16-9B).
Although squamous cell carcinoma (Fig. 16-10) is usually p63-positive, there is no marker to date that can distinguish between primary and metastatic squamous cell carcinoma. However, p63 is an important immunostain in cases of poorly differentiated NSCLC, where the distinction between adenocarcinoma and squamous cell carcinoma is virtually impossible to make on the basis of hematoxylin-eosin slides alone (Fig. 16-11).
Determining the Neuroendocrine Status of Tumors
Both chromogranin (reacts with cytoplasmic neuroendocrine granules) and synaptophysin (reacts with a cell membrane glycoprotein) are used to diagnose the neuroendocrine tumors of the lung. All typical and atypical carcinoid tumors stain
with chromogranin and synaptophysin, whereas small-cell carcinoma is negative in 25% of cases (Figs. 16-12 and 16-13). Neuroendocrine markers are valuable in diagnosing “NSCLC with neuroendocrine differentiation,” a specific group of poorly differentiated tumors without a neuroendocrine morphology.
with chromogranin and synaptophysin, whereas small-cell carcinoma is negative in 25% of cases (Figs. 16-12 and 16-13). Neuroendocrine markers are valuable in diagnosing “NSCLC with neuroendocrine differentiation,” a specific group of poorly differentiated tumors without a neuroendocrine morphology.
Distinguishing Between Malignant Mesothelioma and Lung Adenocarcinoma
Immunohistochemistry is most valuable in distinguishing between malignant mesothelioma and lung adenocarcinoma. A panel of four markers, two positive in mesothelioma and two negative in mesothelioma (but positive in adenocarcinoma), are used routinely in the pathology laboratory. The stains that are negative in mesothelioma but positive in adenocarcinoma include carcinoembryonic antigen (CEA), B72.3, Ber-EP4, and MOC31. The stains that are sensitive and specific for mesothelioma are WT-1, calretinin, D2-40, and cytokeratin 5/6 (Figs. 16-14, 16-15, and 16-16).
Principles of Molecular Pathology
Introduction
Discoveries in molecular pathogenetic mechanisms have had a major impact in the field of pulmonary disease, with important implications for the screening, diagnosis, and treatment of diverse pulmonary diseases, including genetic disorders, infections, and particularly cancer. Novel molecular screening techniques are being developed, and new therapeutic approaches are being actively investigated. Molecular biology has had profound effects on the development of new products—including vaccines, therapeutic agents, and novel gene therapies—and is the basis of pharmacogenomics, the study of genes or chromosomal loci involved in determining the responsiveness of an individual to a particular drug.
Small molecules, such as interfering RNA, or chemical compounds modeled on the protein structure of their targets,
are being actively developed and potentially are powerful therapeutic agents owing to their ability to interfere with critical intracellular signaling pathways.
are being actively developed and potentially are powerful therapeutic agents owing to their ability to interfere with critical intracellular signaling pathways.
![]() Figure 16-14. Epithelioid mesothelioma (WT1, × 200). 93% of the mesotheliomas show nuclear positivity. Lung adenocarcinomas are negative. |
As a result of all these developments, those involved in patient care and treatment should have an understanding of molecular biology and related techniques to facilitate selection of the most appropriate investigation or therapy for their patients, understand the novel agents and techniques that are under investigation in clinical trials, and communicate adequately with their increasingly well-informed patients. In addition, it is imperative that institutions and pathology departments bank frozen tissue on a routine basis with patient informed consent because DNA, RNA, and protein extracted from such material is of paramount importance for evaluating genetic information.
Important aspects of lung cancer pathogenesis are discussed below, including genomic instability in lung cancer, proto- oncogenes, tumor suppressor genes, abnormalities leading to evading apoptosis, cell immortalization, angiogenesis, and abnormalities in the immune response in lung cancer.
General Principles
DNA and RNA are designed to retain, store, and express genetic information in the form of proteins and have an intrinsic functional role. DNA comprises two antiparallel strands of nucleotide bases wound around each other in a right-handed double helix and aligned on a sugar-phosphate backbone. The negatively charged sugar-phosphate molecules are on the outside, and the planar bases of each strand, stacked one above the other, form the center of the helix. The strands are joined by noncovalent hydrogen bonds between pairs of bases on opposite strands. The sequence of one DNA strand uniquely specifies the sequence of the other strand; this complementarity underlies the ability to perform in vitro manipulation of nucleic acids. DNA is organized into highly compact, regular units called chromosomes. It is an extraordinarily stable molecule and loses its normal conformational structure only at extremes of heat or pH or in the presence of destabilizing agents. DNA can be extracted from formalin-fixed archival paraffin blocks of tissue. RNA, in contrast, is much less stable than DNA because of its single-stranded, more random structure; its susceptibility to alkaline hydrolysis; and its rapid degradation by ubiquitous enzymes called RNAses. With current technology, useful amounts of RNA can be extracted from fresh tissue or fresh-frozen samples. It is difficult to retrieve good-quality amplifiable RNA from archival paraffin-embedded tissue because of degradation of the RNA that is extracted. New extraction methodologies are being developed in an attempt to overcome some of the problems of RNA extraction from archival material.
A gene is a portion of DNA that contains the amino acid sequence code to create a protein. Protein synthesis begins with the activation of the appropriate gene. A copy of the gene is made by the process of transcription, which is the synthesis of complementary single-stranded messenger RNA (mRNA) and takes place in the nucleus. Before export to the cytoplasm,
mRNA undergoes posttranscriptional modification. Pre-mRNA contains both amino acid coding sequences called exons and intervening noncoding sequences called introns. The introns are excised from mRNA by a process called splicing. mRNA is subsequently transported from the nucleus to the cytoplasm, where its linear sequence of nucleotide triplets or codons is translated on the ribosomes to form a linear sequence of amino acids. The final product of a gene is the assembly of the constituent amino acids into functional proteins.
mRNA undergoes posttranscriptional modification. Pre-mRNA contains both amino acid coding sequences called exons and intervening noncoding sequences called introns. The introns are excised from mRNA by a process called splicing. mRNA is subsequently transported from the nucleus to the cytoplasm, where its linear sequence of nucleotide triplets or codons is translated on the ribosomes to form a linear sequence of amino acids. The final product of a gene is the assembly of the constituent amino acids into functional proteins.
Control of the process of gene expression ultimately influences the activity or amount of protein product. Gene regulation takes place at both transcriptional and translational levels. Before transcription can be initiated, RNA polymerases (the enzymes responsible for linking together the mRNA polymer) attach initially onto DNA at a sequence termed the promoter. Certain genes, called transcription factors, together with coactivators and corepressors, can influence mRNA production of other genes because they enable RNA polymerase to recognize the promoter. Transcription factors are regulated either by other upstream proteins or by other modifications of the protein, such as phosphorylation. RNA transcription is capable of significant amplification of the signal it encodes.
Most of the human genome is composed of noncoding DNA, the function of which has not been fully elucidated. Most of the variability among individuals occurs in noncoding DNA; this variability is termed genetic polymorphism. Repetitive noncoding sequences are present in multiple copies throughout the genome in tandem arrays called satellites or microsatellites, according to their length. In addition, single nucleotide changes can be polymorphic in the population. Single nucleotide polymorphisms (SNPs) are the most abundant form of DNA polymorphism in the human genome. Alterations of microsatellite sequences, a phenomenon known as microsatellite instability (MI), is often due to malfunction of DNA repair enzymes. MI occurs in several tumor types, including some lung cancers, and is particularly important in a form of hereditary colon cancer. Restriction fragment length polymorphisms (RFLPs), microsatellites, and SNPs are well suited for genetic linkage studies in humans. Assessment of the statistical significance of linkage is made by calculating the ratio of the likelihood of linkage to the likelihood of random assortment.
Hybridization Assays
The ability to manipulate nucleic acids in vitro is based on molecular hybridization, which is the complementary pairing of bases between the target nucleic acid and a DNA or RNA probe. The process of hybridization can be accomplished on solid support, such as nitrocellulose or nylon membranes, as in Southern blotting; in solution, as in the PCR; or at the cellular or subcellular levels, as for in situ hybridization (ISH).
Probes may be labeled either with a radioisotope or, more commonly, with a variety of nonisotopic reporter molecules by a color reaction. A limitation of ISH is its failure to detect oncogenes activated by mechanisms other than dysregulated transcription, such as point mutations or posttranscriptional modifications. ISH can be used to identify cells that contain RNA for a protein of interest.
FISH is a molecular cytogenetic technique that permits the identification of translocations, deletions, structural rearrangements, LOH, and gene amplification assessment in interphase nuclei using fluorochrome dyes. FISH is especially effective when performed on touch imprints but can also be performed on either frozen sections or formalin-fixed and paraffin-embedded tissue. Computerized, bar code–controlled instruments are available that perform fully automated ISH, including FISH, immunohistochemistry, and a dual-staining combination of ISH and immunohistochemistry, and are becoming more commonly used in clinical practice. Using this instrument, conditions could be tightly controlled, resulting in increased reproducibility, reduced hybridization time, and diminished labor.21
PCR involves the exponential in vitro amplification of a segment of DNA. It also involves a repetitive cycle of heat denaturation of DNA, annealing of sequence-specific oligonucleotide primers flanking the selected DNA fragment to be amplified, and synthesis of the target sequence by heat-stable DNA polymerases. The reaction results in the preferential amplification of the target sequence on the background of extraneous DNA, producing virtually unlimited amounts of the target. Short segments of DNA extracted from formalin-fixed, paraffin-embedded tissues can also be amplified using PCR. Reverse-transcriptase PCR (RT-PCR) is a technique that can quantify gene expression by using PCR amplification of target RNA that has been previously converted to complementary DNA (cDNA) by reverse transcriptase. RT-PCR offers several advantages over Northern blotting because only minute tissue samples are required (e.g., from laser microdissection of tissue) and the technique can be quantitative. Several modifications of the basic PCR technique can be useful for specific indications.
Microarray Technologies
Oligonucleotide and cDNA Microarrays
New genomic and proteomic technologies are emerging that will facilitate a global overview of the complex changes implicit in disease states in terms of gene expression and protein complement, facilitating the dawning of an era of molecular classification of disease.104 To create a cDNA microarray, thousands of synthetic oligonucleotide or cDNA probes are constructed to portions of the target sequence on a support grid. The bound sequences are then hybridized to labeled nucleic acids extracted from the specimen of interest. After hybridization, a reading device scans the entire chip. DNA chips enable the expression level of hundreds of genes to be determined in a single experiment.
In cancer research, microarray technology can identify differences in the transcription profile between tumor and normal tissue or between tumors. Microarray applications have been used mainly to identify differences between gene expression profiling, but they can also be used for mutation screening and for polymorphism genotyping.
Comparative Genomic Hybridization
Comparative genomic hybridization (CGH) is a molecular cytogenetic approach that results in an analysis of gene dosage on a genomewide scale by comparing hybridization of test and reference genomic DNA.63 A cytogenetic pattern of genetic losses and gains is analyzed, and a quantitative change is obtained (compared with the normal reference sample). To increase the
mapping resolution, array CGH has been developed, in which cloned DNA targets are arrayed.107 The DNA targets can be bacterial artificial chromosomes (BACs), yeast artificial chromosomes (YACs), cosmid DNA, or cDNA. With array CGH, mapping resolution reaches the kilobase level and can be used to measure gene copy number with high precision. Array CGH can be performed on DNA obtained from fresh or frozen tissue. However, it is difficult to extract suitable DNA from paraffin-embedded tissue.
mapping resolution, array CGH has been developed, in which cloned DNA targets are arrayed.107 The DNA targets can be bacterial artificial chromosomes (BACs), yeast artificial chromosomes (YACs), cosmid DNA, or cDNA. With array CGH, mapping resolution reaches the kilobase level and can be used to measure gene copy number with high precision. Array CGH can be performed on DNA obtained from fresh or frozen tissue. However, it is difficult to extract suitable DNA from paraffin-embedded tissue.
Single Nucleotide Polymorphism Microarrays
SNPs represent the most abundant form of DNA polymorphism in the human genome. SNP microarrays have several potential applications such as LOH analysis, disease susceptibility studies, and pharmacogenetic and toxicogenetic studies. Using SNP, microarrays could identify patients with different susceptibilities to particular treatments or associated with a given phenotype or molecular profile.
Tissue Microarrays
Tissue microarrays (TMAs) facilitates the simultaneous analysis of gene expression at the cellular level synchronously in a large number of tumors.70 Up to 1,000 cylindrical tissue biopsies obtained from formalin-fixed paraffin-embedded individual tumors can be distributed in a single TMA. Ideally, several samples (generally three) from each tumor are assessed simultaneously for optimal results.55 Sections of the paraffin block containing the microarray enable parallel in situ detection of DNA, RNA, and protein targets in each specimen on the array, and consecutive sections allow the rapid analysis of hundreds of molecular markers in the same set of specimens. Analysis of large numbers of clinical specimens is limited by the time-consuming interpretation of hundreds of specimens on each slide. Instruments allowing automated acquisition and analysis of data from specimens are available. Modified TMAs, called cryoarrays, have been created for frozen tissue samples, allowing preservation of nucleic acids and proteins.55,117
Proteomics
The proteome refers to the protein complement of cells, tissue, or serum and reflects the effects of posttranscriptional regulation. Proteomics refers to the characterization of proteins, protein–ligand interaction screening, and differential protein expression profiling. Proteome array–based methodologies are under active investigation in many fields, including lung cancer. Proteomic spectra are generated from the sample of interest through matrix-assisted laser desorption and ionization time-of-flight mass spectroscopy, and the spectra are subsequently analyzed by complex algorithms. Proteomics is a very powerful tool to search for markers of disease, particularly for early cancer detection. Using similar methods, it may also be possible to identify distinct protein and peptide patterns in bronchoalveolar lavage (BAL) fluid or serum between patients with lung cancer and healthy individuals.46,94 Databases of protein expression in lung cancer are being established. In addition, proteomics is used to evaluate the treatment effects of lung cancer.
Laser Capture Microdissection
Most or all lung tumors are heterogeneous in terms of their tissue composition. Laser capture microdissection (LCM) is a technique that allows pure populations of cells to be isolated rapidly from a tissue section for further study. Analysis of the isolated cells can be at the DNA, RNA, or protein level. Thus, LCM is a powerful technique that enables comparison of two distinct cell populations from a given section of tissue. LCM uses a focused laser beam to isolate and capture cells from glass slides for analysis. The time required to collect the cells is minimal, and the isolation of specific cellular subsets from heterogeneous tissue is more accurate than manual dissection. LCM can be used to obtain cells from both fixed and frozen material. Identification of pure populations of cells can be difficult in frozen sections because it may not be possible to distinguish the different cells reliably.
Activation-Specific Antibodies
There have been significant advances in the development of antibodies that recognize activated forms of proteins for use in both Western blots and immunohistochemistry. Activated forms of oncogene products are key elements in important cell signaling pathways. Phosphospecific antibodies can detect phosphorylated form of proteins (e.g., phospho HER-2/neu) and thus detect activation of the oncogenic protein. Antibodies that can detect proteins that are activated by acetylation are also under active development. The ability to detect activated forms of proteins has important implications for the evaluation of prognostic markers and the monitoring and development of targeted treatments.
RNA Interference (Small Interfering RNAs)
RNA interference, an evolutionary conserved cellular process of gene silencing first identified in plants, is a process whereby double-stranded RNA (dsRNA) binds to and results in the degradation of mRNA, based on complementary base pairing. The siRNAs are short dsRNAs (21 to 23 nucleotides in length) that control important signaling pathways in mammalian cells by blocking the transcription of proteins from coding RNA.31 The precise mechanism of action of gene silencing by siRNA has not yet been fully elucidated, but there is no question that it is a very important mechanism used in the regulation of gene expression. RNA interference technology is being exploited for functional genomic analysis and for the development of highly specific targeted treatments.67 The technology is also being used for the creation of animal models of disease—for example, by blocking the transcription of key tumor suppressor genes. In contrast to antisense RNA, siRNAs do not appear to incite the same nonspecific immune responses when injected into the host and may thus hold promise as therapeutic tools.
Molecular Pathogenesis of Lung Cancer
The role of molecular pathology in cancer diagnosis includes the following: detection of both somatic and germline mutations in oncogenes and loss or mutations in tumor suppressor
genes (TSGs); determination of overexpression of oncogenes; assessment of cytogenetic abnormalities and of clonality; determination of susceptibility to cancer by linkage analysis; molecular profiling of lung cancer with identification of novel prognostic and therapeutic markers; and development of sensitive and specific screening tests.
genes (TSGs); determination of overexpression of oncogenes; assessment of cytogenetic abnormalities and of clonality; determination of susceptibility to cancer by linkage analysis; molecular profiling of lung cancer with identification of novel prognostic and therapeutic markers; and development of sensitive and specific screening tests.
Oncogenes and Tumor Suppressor Genes
Protooncogenes are highly conserved cellular genes that are important in cellular pathways involved in proliferation, apoptosis, and differentiation. Generally, only one of the two alleles in a cell needs to be mutated to confer dominant oncogenic properties by altering the normal structure, expression pattern of the protooncogene, or both. Thus oncogenes are genes that, when mutated in a critical area, promote carcinogenesis in a dominant manner. The activation of an oncogene may be associated with increased expression of its protein products. Overexpression of an oncogene can occur for a number of reasons: genomic amplification; juxtaposition to strong viral or tissue-specific promoters controlling its transcription (e.g., after a translocation event); or constitutive deregulated expression that follows a mutational event. Rearrangements of chromosomes—resulting in truncations, insertions, or deletions—may also result in oncogene activation or inactivation of TSGs. Most oncogenes that have been identified are part of the cell’s growth regulatory pathways or are homologs thereof and include growth factors, growth factor receptors, intracellular signal transducers, cell cycle proteins, and nuclear transcription factors.
Many common sites of LOH exist in lung cancer, both SCLC and NSCLC, suggesting the presence of alterations in TSGs at these sites of chromosome loss.9 Several well-described TSGs are known to be altered in lung cancer and almost certainly play a role in their evolution. TSGs code for proteins whose loss of function results in transformation, in contrast to the activating mutations that generate oncogenic alleles from protooncogenes. Both alleles of a TSG must be either lost, mutated, or inactivated by epigenetic means to result in inactivation of the gene, although haploinsufficiency of certain TSGs, such as PTEN and p27, can result in greater susceptibility to cancer in the experimental setting. When one allele is inactivated in the germline, such a genotype is transmitted to all somatic cells, rendering them more susceptible to a second mutational event that can affect the remaining functional allele. This results in a greater predisposition to cancer in families carrying such germline mutations.
Inherited Predisposition to Lung Cancer
The contribution of hereditary factors to lung cancer development is less known. Published analyses demonstrate a 2.5-fold increased risk attributable to a family history of lung cancer independent of tobacco smoke, suggesting that genetic factors other than those related to metabolizing carcinogens from tobacco smoke may influence the susceptibility to develop lung cancer.3,116 A recent linkage analysis suggests that a major autosomal susceptibility locus for inherited lung cancer is present on 6q23-25.8 Lung cancer has been documented in some genetic syndromes that have an increased predisposition to other cancers. Lung cancer occurs with increased frequency in the Li–Fraumeni syndrome, which results from a germline mutation in the p53 TSG on chromosome 17.75 Donehower and colleagues29 showed that p53 knockout mice are predisposed to develop lung adenocarcinoma.29 Lung cancers have also been described in carriers of inactivating mutations in the retinoblastoma TSG on chromosome 13. Studies have shown that only 10% of smokers develop lung cancer.85 Evidence shows an important role for genetic predisposition in determining risk for lung cancer development among smokers.120 A growing body of data links genetic differences in the capacity to metabolize tobacco carcinogens with the risk for developing lung cancer. Certain enzymes influence lung cancer risk by catalyzing detoxification reactions that enhance the elimination of the toxic products, and genetic polymorphisms of these enzymes appear to relate to lung cancer susceptibility.13,74,143 A genetic profile of each of these factors might define a significant indicator of risk status in a person who smokes, which could be a candidate for early detection and prevention programs.
Genetic Alterations in Sporadic Lung Cancer
K-ras
The ras/raf/mitogen-activated protein kinase (MAPK) pathway is probably the best-characterized signal transduction pathway in cell biology.89 Ras is the most frequently mutated oncogene in human cancer. Mutations in the K-ras oncogene have been most commonly localized in codons 12 and 61. K-ras mutations occur in 30% to 50% of lung adenocarcinomas.18,26,114 Although these mutations are more common in adenocarcinomas than in squamous cell carcinomas, they are very rare in the bronchioloalveolar subtype of lung adenocarcinoma. K-ras mutations have not been described in SCLC.119 Recent studies show that in bronchioloalveolar carcinomas and adenocarcinomas with bronchioloalveolar growth patterns that have mucinous differentiation, there was a significant correlation with the absence of EGFR mutation and presence of K-ras mutation, suggesting that these cancers with mucinous features are unlikely to respond to TKIs.35 Ras proteins have been implicated in the transduction of growth and differentiation signals from activated transmembrane receptors to downstream protein kinases. When ras is mutated, the major defect is a marked decrease in its ability to interact with the guanosine triphosphatase (GTPase)–activating protein RAS-GAP. Hence the Ras protein remains in the GTP-bound or active state, and regulation of cell growth is disturbed. K-ras mutations could transform cells, thus establishing ras as an oncogene.72 Mutations in K-ras predominantly occur in codons 12 (85% of all mutations), 13, and 61 and result in constitutive activation of the extracellular signal (regulated kinase [Erk] signaling pathway in the absence of external stimuli). Westra and colleagues have demonstrated K-ras mutations in early-stage lung tumors, and it is believed that these mutations are involved in tumor initiation and early progression.154 Interestingly, Zhang and colleagues160 demonstrated that wild-type K-ras inhibits mouse lung carcinogenesis and tumorigenic properties of lung tumor cell lines, providing evidence for a tumor suppressor function for K-ras in its nonactivated form.160 A mutated K-ras gene has been shown to confer a worse prognosis to lung adenocarcinomas that were surgically treated with curative intent. However, the prognostic significance of specific K-ras mutations requires additional studies. K-ras mutations
have been detected in atypical adenomatous hyperplasia.113 Six of the eight patients in whose lung tumors K-ras mutations were present had detectable K-ras mutations in sputum 1 to 4 months before clinical detection of the tumor; no mutations were found in sputum of patients with no mutations in their subsequent lung tumors.82,88,157 Thus, the detection of a K-ras mutation may be useful in the early detection of lung adenocarcinoma, perhaps as part of a panel of informative biomarkers. Multiple studies have shown that oncogenic KRAS (e.g., KRASV12 mutant) activates cell-signaling pathways important to cellular transformation.136 Therefore KRAS abnormalities represent an important therapeutic target.116 New therapeutic approaches include the inhibition of K-ras by small molecules that block posttranslational modifications required for the activation of K-ras.42
have been detected in atypical adenomatous hyperplasia.113 Six of the eight patients in whose lung tumors K-ras mutations were present had detectable K-ras mutations in sputum 1 to 4 months before clinical detection of the tumor; no mutations were found in sputum of patients with no mutations in their subsequent lung tumors.82,88,157 Thus, the detection of a K-ras mutation may be useful in the early detection of lung adenocarcinoma, perhaps as part of a panel of informative biomarkers. Multiple studies have shown that oncogenic KRAS (e.g., KRASV12 mutant) activates cell-signaling pathways important to cellular transformation.136 Therefore KRAS abnormalities represent an important therapeutic target.116 New therapeutic approaches include the inhibition of K-ras by small molecules that block posttranslational modifications required for the activation of K-ras.42
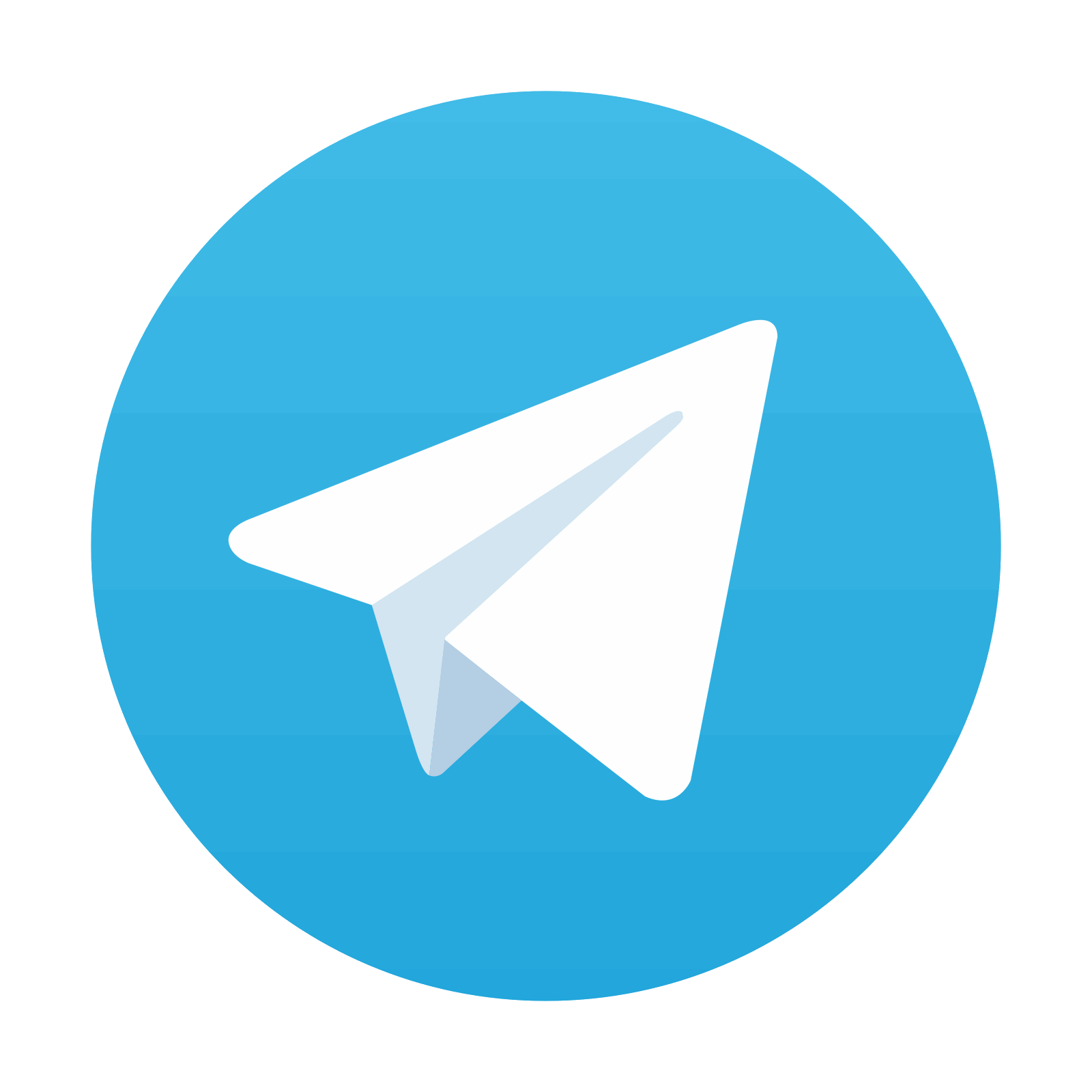
Stay updated, free articles. Join our Telegram channel

Full access? Get Clinical Tree
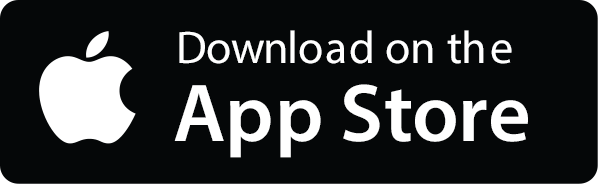
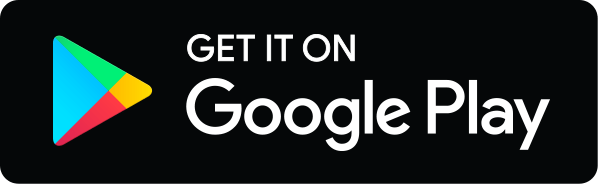
