Overview
Over the past 50 years, new medical devices and related innovations have contributed greatly to the decrease in deaths from cardiovascular causes. Indeed, cardiovascular device development has advanced dramatically over the past several decades, yielding a remarkable increase in the variety and complexity of available devices and diagnostic tests for cardiac illnesses. In ischemic, valvular, myocardial, cardiac rhythm, and peripheral vascular disease, the clinical benefits have been substantial.
* This work represents the professional opinion of the authors and is not an official document, agency guidance, or policy of the U.S. Government, the Department of Health and Human Services, or the Food and Drug Administration, nor should any official endorsement be inferred.
Catheter-based endovascular stents emerged in the 1990s. Initially composed of bare-metal wires and later with drug-eluting coatings, stents have revolutionized the percutaneous treatment of severe coronary atherosclerosis. Metallic stents have been approved as a percutaneous alternative to surgical carotid endarterectomy. In valve disease, a singular advance was surgical valve replacement, which began in the 1960s. In contrast to a mortality rate of 50% at 2 to 3 years in patients with critical aortic stenosis, survival following surgical valve replacement with contemporary mechanical or bioprosthetic devices is 50% to 70% at 10 to 15 years. A new approach to valve replacement is transcatheter aortic and pulmonary valve implantation, less invasive interventions currently at varying stages of development, clinical study, and clinical use.Devices that aid or replace the heart’s pumping function, including ventricular assist devices (VADs) and total implantable artificial hearts, are used less frequently than stents or valves, but their implantation enables survival of some patients who would otherwise succumb without profound cardiac support. Pacemakers, implantable cardioverter-defibrillators (ICDs), radiofrequency catheters, and cryoablation catheters have substantially improved the prognosis of patients with life-limiting and life-threatening cardiac arrhythmias. Synthetic vascular grafts and stent grafts provide effective repair of stenotic peripheral arteries and aneurysmal disease of the thoracic and abdominal aorta. Vascular grafts have also enabled long-term vascular access for hemodialysis treatment in patients with renal failure who lack suitable veins for arteriovenous (AV) fistulas.
Most permanently implanted cardiovascular devices are designed to treat underlying medical conditions or provide enhanced function. Nevertheless, device failure and/or other tissue-biomaterial interactions may cause complications that necessitate reoperation or cause morbidity or death. In some cases, deleterious outcomes occur after many years of uneventful patient benefit ( Figure 3-1 ).

Assessment of the safety and effectiveness of new cardiovascular products, and the ongoing evaluation of approved products, is challenging. The Food and Drug Administration (FDA) Center for Devices and Radiological Health (CDRH) plays an essential role in promoting and protecting the public health by ensuring that medical devices marketed in the United States provide a reasonable assurance of safety and effectiveness and confer a favorable risk/benefit profile for their intended use population.
This chapter discusses the process of cardiovascular medical device development, validation, and regulatory review and describes differences in the regulation of devices versus drugs; it also covers special topics of interest to the practicing physician, including off-label use and the responsibilities of cardiologists to ensure safe and effective use of devices.
Medical Device Development and Differences from Drugs
Development and Implementation of Cardiovascular Medical Devices: An Overview
The medical device development process has become increasingly complex in recent years as a result of the advent of new technologies, regulatory requirements, and the increased importance of reimbursement decisions for successful device commercialization. The entire process requires strategic planning, coordinated decisions, and consistent, rigorous scientific and business methods. Development and clinical use of a medical device comprise a complicated process, progressive but not entirely linear, that includes concept generation, prototype development, intellectual property development, regulatory requirements, reimbursement issues, business models, research and development (including the scientific and engineering work required to transition from an early-stage concept to a user-ready and validated final device), clinical trials, marketing and stakeholder considerations, quality and process management, manufacturing, and sales and distribution. From a technical perspective, key considerations of the development of a design concept responsive to a clinical need include selection and evaluation of suitable biomaterials and incorporation of the materials into a device prototype to evaluate functionality and anticipated potential complications via bench testing and in animal models ( Figure 3-2 ). After FDA approval of an Investigational Device Exemption (IDE), for a significant risk investigational device, a human clinical study of the device may be conducted under carefully monitored clinical trial conditions. All preclinical and clinical data undergo regulatory evaluation prior to market entry via the premarket notification, 510(k), or the premarket approval application (PMA) regulatory pathway (see below). At any point in the development and use of an investigational device, untoward results and subsequent analysis, which frequently includes implant retrieval and pathologic evaluation, may necessitate reassessment of the device concept, modification of biomaterial or design, and adjustments in the management of patients who have received the device. Any of these changes will affect regulatory review.

Differences Between Devices and Drugs and Associated Regulatory Implications
Medical devices and drugs differ in both their development and regulatory pathways; the latter are set forth in the statutory mandate given to the FDA by the U.S. Congress. Drugs are chemical entities that may be metabolized either before or after their intended action. They have a measurable half-life and are ultimately metabolized and/or excreted. Drugs solve a biochemical problem; their action is systemic and cannot be seen directly, and their mechanism of action is often not well understood. In contrast, medical devices predominantly solve a mechanical or other physical problem; their intended action is generally local, and their mechanism can typically be directly or indirectly observed. Furthermore, although drugs are metabolized and/or excreted with a measurable half-life and can be redosed or discontinued with ease, devices are often permanent implants; their removal may have important clinical implications. Therefore, device interactions with the patient are frequently ongoing, and the risks of potential adverse effects can be prolonged for many years. Moreover, unlike a drug regimen, nonadherence with an implanted medical device is not an option; however, nonadherence with adjunctive drug regimens, such as anticoagulants or antiplatelet agents, can cause significant problems.
Surgeons and interventionalists are often involved in the development and evaluation of devices, and operator technique, expertise, and thus the extent of experience with a particular device type, can play a critical role in the successful use of a device. Phase III drug trials often recruit thousands of patients over a relatively short time, but the number of patients available for pivotal clinical trials of new or modified medical devices is typically smaller. Moreover, double-blind, randomized, controlled trials (RCTs) are often not feasible for evaluation of medical devices. Large companies dominate the pharmaceutical industry, but smaller companies are often intimately involved in medical device development from concept through market entry, and the early stages in the process may be marked by iterative innovation in device design and biomaterials.
Table 3-1 summarizes key features of devices and drugs that influence development and regulatory review; they include device-related and population-related factors. Contemporary publications have amplified these differences.
DEVELOPMENTAL FEATURE | DEVICE | DRUG |
---|---|---|
Rate of technology change | Fast | Slow |
Mode of action | Physical effect | Chemical effect |
Duration | Long | Short |
Potential adherence issues | No | Yes |
Learning curve | Yes | No |
Ease of in vitro assessment | High | Low |
Ability to blind treatments | Difficult | Easy |
Ability to recruit large patient groups | Difficult | Easy |
Regulatory Fundamentals
History of Device Regulation and the Medical Device Classification System
The 1976 Medical Device Amendments created a system for the FDA review process for medical devices. Three separate classification levels were designated based on the device’s level of clinical risk. Class I devices include minimal risk devices such as bandages, examination gloves, and certain manual, handheld surgical instruments. The majority of class I devices are exempt from premarket notification and FDA clearance before marketing. These devices are subject to General Controls—the basic requirements of the Food, Drug and Cosmetic Act (as amended) that apply to all medical devices—which include product registration and listing requirements, Good Manufacturing Practices, labeling requirements, banning provisions, and medical device reporting (MDR) requirements.
Class II devices represent intermediate risk, and most require submission of a 510(k) application to the FDA before the device can be cleared for marketing. Examples of class II cardiovascular devices include guidewires, guide catheters, introducer sheaths, hemostasis devices, and computerized electrocardiographic devices. In addition to General Controls, class II devices must also comply with Special Controls, which include device-specific labeling requirements, performance standards, and postmarket surveillance.
The highest risk devices are categorized as class III devices, which are used in supporting or sustaining human life, are of substantial importance in preventing impairment of human health, or present a potential unreasonable risk of illness or injury (21 C.F.R., Part 814). In most cases, Class III devices require the submission and FDA approval of a PMA prior to marketing. Any device in commercial distribution prior to the passage of the 1976 Medical Device Amendments was considered a preamendment device and was effectively grandfathered; that is, it was allowed to stay on the market without additional FDA review, unless the FDA had taken a specific regulatory action to require a PMA. These devices were assigned to the least-regulated class that allowed a reasonable assurance of safety and effectiveness. A device marketed for the first time after 1976 must follow the regulatory requirements for its particular device classification.
Pathways for Regulatory Review of Cardiovascular Devices
The first step in the device evaluation process is to determine the device’s classification level to determine the regulatory requirements. Based on the device classification, a medical device manufacturer would usually take either of two key regulatory pathways: the 510(k) premarket notification submission or submission of a PMA ( Figure 3-3 ).

510(k) Premarket Notification
The 1976 Medical Device Amendments added a premarket notification provision to Section 510(k) of the Food, Drug and Cosmetic Act, requiring that each firm register their manufacturing facility with the FDA. For those devices that require a 510(k) submission prior to marketing, the application should include a description of device design, function, and principles of operation; reference to performance standards if available; a bibliography of all published and unpublished reports; proposed labeling; and manufacturing information. The manufacturer must demonstrate that the new device is “substantially equivalent” to one or more legally marketed devices, known as predicat e devices, in terms of the intended use, technology, and performance. If the new device has different technological characteristics, the differences must not raise new safety or effectiveness concerns, and the manufacturer must demonstrate that the new device is at least as safe and effective as the predicate. The FDA has a statutory requirement of 90 days to review and make a marketing clearance determination. A manufacturer must receive a clearance letter from the FDA allowing it to market the device prior to its commercial distribution in the United States.
The majority of medical devices the FDA has approved for the U.S. market have entered via the 510(k) premarket notification process; most are cleared after FDA review of comprehensive nonclinical testing (bench studies and animal testing, when necessary). For example, percutaneous transluminal coronary angioplasty (PTCA) catheters are considered class II devices. A new PTCA catheter would generally require bench testing alone; however, a new clinical study would be warranted if the indications for use were significantly different, or if the technology raised safety or effectiveness concerns that could only be addressed with a clinical study. Approximately 10% to 15% of 510(k) submissions contain data from clinical trials. For example, an intravascular embolic protection device is a class II device that typically requires clinical data for a determination of substantial equivalence.
Premarket Approval Application
As a condition for FDA approval of a PMA, a manufacturer must demonstrate reasonable assurance of the safety and effectiveness of a device for its indications for use. For class III cardiovascular devices—such as heart valves, pacemakers, intracoronary stents, and circulatory support devices—a demonstration of device safety and effectiveness almost always requires clinical data to form the basis of product approval. In determining the safety and effectiveness of a class III device, some of the factors considered by the FDA include the intended use of the device, the population for which the device is intended, device reliability, and the risk of device use compared with the likely benefit of using the device. To make a determination of a reasonable assurance of safety and effectiveness, the FDA relies on valid scientific evidence . * A hierarchy of the types of data that comprise valid scientific evidence exists, from RCTs, partially controlled studies, studies without matched controls, and well-documented case histories conducted by qualified experts to reports of significant human experience with a marketed device.
* 21 C.F.R. § 860.7(c)(2) Valid scientific evidence is defined as “evidence from well-controlled investigations, partially controlled studies, studies and objective trials without matched controls, well-documented case histories conducted by qualified experts, and reports of significant human experience with a marketed device, from which it can be fairly and reasonably concluded by qualified experts that there is a reasonable assurance of the safety and effectiveness of a device under its conditions of use.”
The main goal of FDA device review is to assess the clinical utility of the device, based on its risk/benefit profile, to determine product safety and effectiveness. The FDA’s interpretation of valid scientific evidence for medical device approval has become more rigorous for cardiovascular devices over the past decade, incorporating greater use of randomized, controlled, and even blinded studies when applicable.Investigational Device Exemption
Clinical studies performed in the United States using investigational devices that present a significant risk to human subjects (some class II devices and all class III devices) are performed under an IDE application approved by the FDA. IDE applications typically provide a detailed device description; proposed indications for use; a report of prior investigations, including all nonclinical studies (bench and animal); previous clinical experience; a summary of the manufacturing process and quality systems; the proposed investigational plan; proposed labeling; and an informed consent document to be used in the study. FDA approval of an IDE gives permission to a manufacturer or clinical investigator to conduct a study of an investigational device, or of an approved device for nonapproved indications, on patients in the United States to generate data in support of the device’s safety and effectiveness. The purpose of an IDE is to “encourage…the discovery and development of useful devices intended for human use,” while at the same time protecting the public health and ensuring that clinical investigations are performed in a safe and ethical manner (21 C.F.R. §812.1[a]). To maintain optimum freedom for scientific investigators in the pursuit of device development, the statutory time requirement for the FDA to complete its review of an IDE application is 30 calendar days. After reviewing the IDE application, the FDA may grant 1) full approval to begin the clinical trial; 2) conditional approval, which indicates that the FDA deems the trial sufficiently safe to commence, but there remain some outstanding issues that need to be addressed by the sponsor prior to full approval; or 3) disapproval. Clinical data obtained from a study under an approved IDE can then be used to support a 510(k) or PMA, depending on the pathway necessary for marketing.
Humanitarian Device Exemption
A Humanitarian device exemption (HDE) application is an option for devices intended for use in a very small patient population (<4000 individuals per year) for an uncommon clinical condition. An HDE application is similar in both form and content to a PMA and has the same safety requirements. However, in contrast to a PMA, in which the devices must provide a reasonable assurance of safety and effectiveness, an HDE application must demonstrate that the device is safe and provides probable benefit to the patient.
Contemporary Regulatory Issues
Randomized Versus Nonrandomized Studies in Medical Device Evaluation
Determination of what constitutes an appropriate level of valid scientific evidence depends on the type of technology used and the risk posed by the device. The FDA considers data from RCTs to be the highest level of scientific data and therefore encourages use of RCTs in cardiovascular device studies. However, the use of an RCT design may be challenging with some cardiovascular devices because of sample size issues or ethical dilemmas. Low adverse-event rates associated with mature device technologies, such as surgical prosthetic heart valves, would require an unfeasible sample size to demonstrate either superiority or noninferiority of the new device compared with the control. For disease entities in which there is either no standard of care, or the standard of care is known to be suboptimal, lack of clinical equipoise can create ethical dilemmas by mandating that patients be randomized to a study arm that may be perceived by clinicians and/or patients to be an inferior treatment. Thus, a proper assessment of device technology must balance the competing demands of maximizing scientific validity against the practical realities of performing (and effectively completing) clinical studies. For this reason, nonrandomized clinical studies may be acceptable in certain situations in support of a marketing application.
For surgical heart valves, a mature technology with well-defined performance profiles and complication rates, the FDA took the approach that a rigorously evaluated historic control dataset could be used in single-arm trials of new heart valves to define acceptable rates of the most frequent complications. In 1993, in consultation with a committee of experts from industry and academia, the FDA developed objective performance criteria (OPC) derived from patient-level data from studies of FDA-approved surgical heart valves, representing a sample size of 800 valve years. This OPC approach is an efficient method to evaluate new surgical heart valves submitted for FDA approval.
Moreover, incremental design changes to an existing cardiovascular device, such as an electrophysiologic ablation catheter well characterized with engineering and animal testing data, may be evaluated for safety and effectiveness with a single-arm clinical study in some circumstances; however, use of a nonrandomized study design must include the careful selection of a suitable historic control (see Chapter 1 ). In addition, a detailed statistical analysis plan should be developed that accounts for differences in baseline clinical covariates and other time-related improvements in cardiovascular disease management that could bias against historic control data. Statistical methodologies such as propensity score analysis are useful to balance measured covariates among nonrandomized treatment arms. However, it must be recognized that all nonrandomized studies are subject to bias, and despite the rigor with which they are applied, propensity score analyses and other methods of statistical adjustment have important limitations. Moreover, because operator technique and expertise can greatly influence clinical outcomes, many studies of novel devices include a “roll-in” phase to account for a physician learning curve.
Endpoints and Surrogate Endpoints in Cardiovascular Device Trials
Ideally, clinical trial endpoints should be objective, readily assessable, clinically important, meaningful to patients, interpretable by physicians, and assessable in a trial of reasonable size. To make a determination of a device’s safety and effectiveness, thorough consideration must be given to select the most informative and relevant endpoints for clinical studies. For most cardiovascular devices, clinical outcome parameters, such as death, myocardial infarction (MI), stroke, and congestive heart failure admission, are used. Composite endpoints representing combinations of clinically important parameters, such as major adverse cardiac events (death, MI, and target lesion revascularization) and target vessel failure (cardiac death, target vessel MI, and target vessel revascularization), are frequently used. Composite endpoints are best suited for well-characterized disease states, when there is consensus that all individual components are clinically important, and for which there is an expectation that all individual components would be affected in the same direction (e.g., trends for reduced rates of death, MI, and stroke).
The main advantages of composite endpoints are amplification of treatment benefits and an increase in the overall number of events so that sample size may be reduced; however, potential limitations on the interpretability of composite endpoint data are evident. For example, in most cases, equal weight applied to components assumes equal importance, which may not be justified, and it may be difficult to find consensus on an acceptable weighting scheme among individual components. There is a chance that the outcomes of individual components could trend in opposite directions (e.g., lower repeat revascularization rates but higher MI rates). Finally, the outcome difference between the new intervention and the control may be driven by the clinically least important component of the composite.
With some cardiovascular devices, successive improvements in device technology have led to decreased rates of adverse events. Improvement in patient outcome is clearly desirable, but it has the effect of making comparative analysis in trials of next-generation devices more difficult. Coronary drug-eluting stent (DES) technology provides one example. * The first two devices to be approved, the Cypher sirolimus-eluting coronary stent (Cordis Corporation, Warren, NJ) and the Taxus paclitaxel-eluting coronary stent (Boston Scientific, Natick, MA), were tested against bare-metal stent controls in their pivotal trials; they demonstrated a reduction in major cardiac adverse-event rates, driven by a dramatic reduction in repeat revascularization rates. In a trial of one DES versus another, low event rates require larger numbers of study patients to demonstrate either superiority or noninferiority of the new device compared with the control stent. One potential approach to designing feasible trials is to enroll a more enriched population that includes higher risk subjects (e.g., non–ST-elevation MI patients) or more complex lesions (long lesions or small vessels) that would be associated with higher event rates.
* It should be noted that coronary DESs are officially classified by the FDA as combination drug-device products, with features of drug as well as device therapies. Based on the FDA’s official designation, CDRH has served as the lead review center for DES, with the Center for Drug Evaluation and Research serving a consultative role. Hence, the clinical testing pathway for DESs has followed the PMA pathway for device approval. Considerations for combination devices are discussed later in this chapter.
A surrogate endpoint is a marker that is intended to substitute for a clinical endpoint and is expected to predict clinical outcomes based on epidemiologic, therapeutic, pathophysiologic, or other scientific evidence. A surrogate endpoint must fulfill two critical criteria to be considered valid: first, the surrogate must be highly predictive of the clinical outcome, and second, it must fully reflect the treatment effect, both positive and negative, on the clinical outcome (see Chapter 1 ). In cardiovascular drug trials, blood pressure reduction and lipid lowering have been used as physiologic and biochemical surrogate endpoints for coronary heart disease and stroke, respectively. Surrogate endpoints have been suggested as alternate outcome measures for technologies such as DESs and devices designed to reduce myocardial infarct size.Percent-diameter stenosis and late lumen loss are potential surrogate markers for clinical effectiveness endpoints for coronary stent trials. Several studies show that these angiographic outcome measures serve as a surrogate for the need for repeat revascularization procedures in the treatment of noncomplex coronary lesions. As a result, percent-diameter stenosis and late lumen loss have the advantage of providing quantitative data for comparisons of different stent implantation strategies. Consequently, they have the potential for increasing the effect size difference between treatments, which requires fewer patients to be studied.
Multiple issues are associated with the exclusive use of imaging-based surrogate endpoints. Angiographic endpoints require optimal image quality, consistent views from the index procedure to follow-up, and standardized core lab protocols and software. By definition, angiographic surrogate endpoints entail a high degree of subject compliance with follow-up angiography. Given the expected low event rates, patients may be reluctant to undergo a protocol-driven, rather than symptom-driven, repeat invasive imaging study. The acceptability of study results could be compromised because of data loss secondary to a substantial subject dropout. In addition, it is uncertain whether imaging-based surrogate endpoint models would apply to more complicated patient and lesion subsets. Finally, although the use of a valid surrogate may result in a reduced sample size to demonstrate device effectiveness, this approach is often inadequate to assess device safety. Alternative trial design strategies that combine conventional clinical outcome measures with a validated surrogate parameter, potentially as co-primary endpoints, may be considered. Because of these considerations, surrogate endpoints may be most useful as primary effectiveness endpoints for second and/or later generation devices (i.e., iterative changes in an approved device). Continued exploration of scientifically valid surrogate endpoints and innovative trial designs may aid in the development of helpful strategies in the assessment of novel technologies.
Study Blinding in Cardiovascular Device Trials
The use of blinding reinforces the integrity of the treatment effect of patient assignment in RCTs. For example, the pivotal studies conducted for the Cypher and Taxus DESs used double-blind study designs, given that the products were visually and radiographically identical in appearance. However, for most cardiovascular device trials, designing a double-blind study cannot be done because of the physical characteristics and/or mode of action of the device, as in the case of a trial of two different DESs in which the operator and catheterization laboratory staff are aware of treatment assignment based on the unique physical properties of each product. Further, neither patient nor operator blinding is possible in a treatment strategy trial such as percutaneous coronary intervention (PCI) versus bypass surgery. Thus, device trials frequently cannot accommodate blinding of both patients and implanting physicians. For example, in the Randomized Evaulation of Mechanical Assistance for the Treatment of Congestive Heart Failure (REMATCH) trial, in which end-stage heart failure patients were randomized to left ventricular assist device therapy or optimal medical management arms, the study could not be blinded. Randomized but unblinded studies have also been used for comparing surgical and interventional therapies for coronary artery obstructions, different types of heart valve prostheses (mechanical vs. biologic substitutes), and open versus transcatheter valve replacement.
Given these limitations on study blinding, it must be recognized that investigator and/or patient bias introduced by the knowledge of treatment assignment may possibly confound clinical study outcomes and diminish the scientific validity of a study. Study designs should therefore incorporate blinding to the maximum extent possible, maintaining the blinding for patients, investigators, and study personnel who conduct follow-up clinical assessments. In addition, the use of objective, rather than subjective, study endpoints and analytical tools to evaluate the potential effect of bias on study outcome augments the scientific validity of the study results.
Use of Foreign Data for U.S. Product Approval
A potential advantage in collecting data from different geographies, in either a single global study or several individual studies, is the ability to evaluate device performance across a more diverse population than can be achieved by a single geographic population alone. Study results could thus be more generalizable to a broader population of patients. Furthermore, demonstration of comparable device performance across different geographies can provide a more robust conclusion of product safety and effectiveness.
Increasingly, studies for cardiovascular devices are conducted in centers outside the United States, and the FDA will consider data obtained from sites outside the United States as supportive evidence for U.S. product approval (21 CFR § 814.15). However, such data must be demonstrated to be applicable to the U.S. population and practice of medicine . Unless consideration of the potential differences in patient populations and study characteristics is made prior to initiating studies outside the United States, the data from such trials might have limited applicability.
A key consideration when assessing data from outside the United States is the generalizability to the U.S. patient population. Important factors include patient demographic and clinical characteristics, geographic differences in medical practice, and differences in study protocols, especially the extent to which patients are monitored for clinical events and long-term follow-up. Prespecified statistical analyses are recommended to evaluate data comparability by testing the homogeneity of demographic and procedural covariates across centers and geographical regions, as well as testing for interactions between treatment and region.
Independent Oversight of Cardiovascular Device Trials
Many cardiovascular device studies evaluate breakthrough technologies that have novel uses and potential unforeseen risks to patients enrolled in clinical trials. To ensure adequate protection of patient safety, the FDA often recommends the use of independent data safety and monitoring boards (DSMBs). Study DSMBs should have monitoring plans to ensure that patients are not subjected to undue risk. In cases where cardiovascular devices are evaluated in multiple, concurrent trials, it may be appropriate to use the same DSMB to streamline safety monitoring from a global perspective. The FDA also strongly recommends the use of independent core labs for imaging and pathology, with clinical events adjudicated by an independent clinical events committee. These independent monitoring bodies complement the role of the study DSMB, reinforce study integrity, and reduce issues of bias and conflict of interest.
Labeling and Off-Label Use of Cardiovascular Devices
One of the most important aspects of the device approval process is the development of understandable and accurate instructions for the use of a product, and the FDA works closely with device manufacturers to craft the product label. Labeling is defined as a “display of written, printed, or graphic matter upon the immediate container of any article,” and it includes “all labels and other written, printed, or graphic matter” (Sections 201[k] and [m] of the Federal Food, Drug, and Cosmetic Act). Device labeling is a means to communicate to physicians and patients a description of the device, how and in whom the device should be used, when it should be used with caution or not used, and the safety and effectiveness outcomes associated with use of the device in one or more clinical studies. The “indication for use” statement identifies the target population, a significant portion of whom have demonstrated through sufficient, valid scientific evidence that use of the device as labeled will provide clinically significant results, and, at the same time, it will not present an unreasonable risk of illness or injury. The label also includes details regarding the clinical studies performed in support of the product’s approval, including the specific populations tested.
Despite specific language in labeling, a physician can use a device in a manner different from the labeled indication because of expectations that the beneficial effects seen in clinical trials may transfer to relatively untested patient subgroups; this is known as off-label use, and it is a means by which a legally marketed device may be used as a physician deems appropriate to benefit an individual patient as part of the practice of medicine. However, both the physician using a device in this way and the patient on whom the device is used should understand that the off-label use may not have been tested sufficiently to establish its full risk/benefit profile, and that the clinical evidence needed to generalize safety and effectiveness expectations to a broader patient population may not be available.
Although off-label use by a physician in the routine practice of medicine is not within the FDA’s regulatory purview, off-label device use diminishes the incentive for a manufacturer to study or seek FDA approval for the indication for which the product is being used off-label. In addition, inadequate safety and effectiveness data to evaluate device performance in important patient subsets limit the ability of a clinician to decide whether a specific product is appropriate for the patient. This lack greatly hinders the process of appropriately informing patients of the risks and benefits of such therapy.
If physicians use a device for an indication beyond the approved labeling, they have the responsibility to be well informed about the product, to base the product’s use on sound scientific rationale, and to maintain records of the product’s use and effectiveness. Specific language introduced by the FDA Modernization Act of 1997 addressed “practice of medicine” (21 U.S.C. 396 § 906, see also Food and Drug Modernization Act of 1997 § 214), stating that “nothing in this act shall be construed to limit or interfere with the authority of a health care practitioner to prescribe or administer any legally marketed device to a patient for any condition or disease within a legitimate health care practitioner-patient relationship.” However, the act did make clear that promotion of medical devices falls under the FDA’s purview, and therefore promotional activity for medical devices must be consistent with the device’s approved labeling.
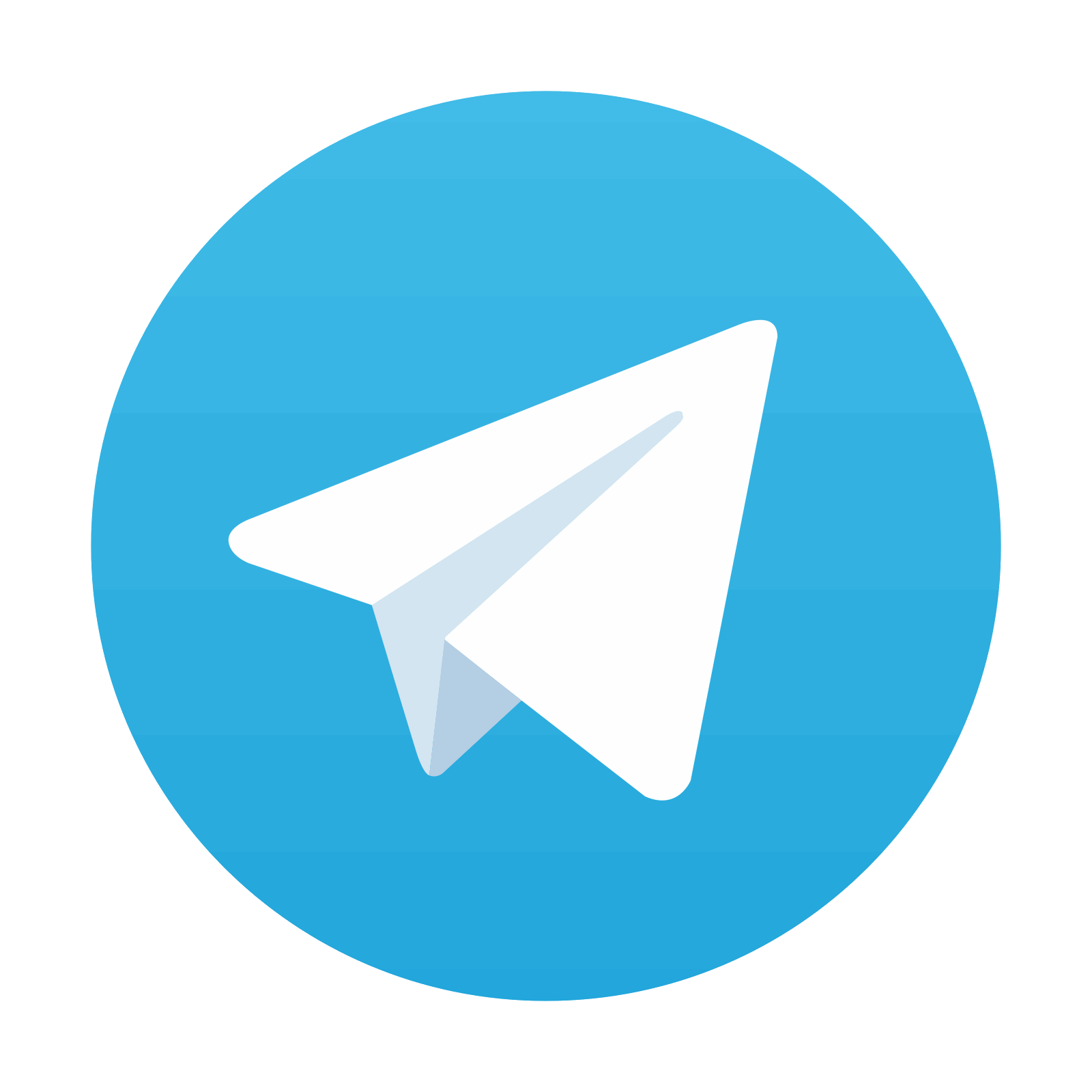
Stay updated, free articles. Join our Telegram channel

Full access? Get Clinical Tree
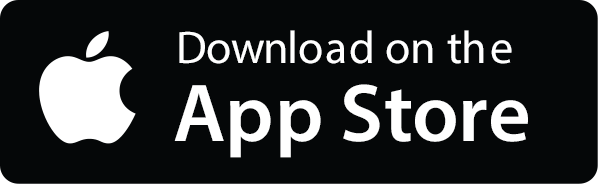
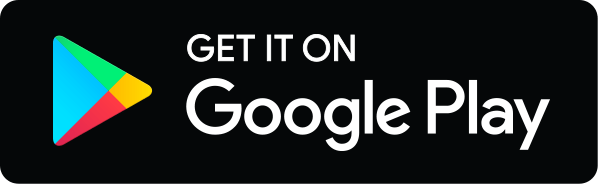